NCBI Bookshelf. A service of the National Library of Medicine, National Institutes of Health.
Balk E, Chung M, Lichtenstein A, et al. Effects of Omega-3 Fatty Acids on Cardiovascular Risk Factors and Intermediate Markers of Cardiovascular Disease. Rockville (MD): Agency for Healthcare Research and Quality (US); 2004 Mar. (Evidence Reports/Technology Assessments, No. 93.)
This publication is provided for historical reference only and the information may be out of date.
This evidence report is 1 of 3 reports prepared by the Tufts-New England Medical Center (Tufts-NEMC) Evidence-based Practice Center (EPC) concerning the health benefits of omega-3 fatty acids on cardiovascular diseases (CVD). These reports are among several that address topics related to omega-3 fatty acids, and that were requested and funded by the Office of Dietary Supplements, National Institutes of Health, through the EPC program at the Agency for Healthcare Research and Quality (AHRQ). Three EPCs - the Tufts-NEMC EPC, the Southern California EPC-RAND, and the University of Ottawa EPC - each produced evidence reports. To ensure consistency of approach, the 3 EPCs collaborated on selected methodological elements, including literature search strategies, rating of evidence, and data table design.
The aim of the reports is to summarize the current evidence on the health effects of omega-3 fatty acids (eicosapentaenoic acid [EPA; chemical abbreviation: 20:5 n-3], docosahexaenoic acid [DHA; 22:6 n-3], alpha-linolenic acid [ALA, 18:3 n-3], and docosapentaenoic acid [DPA, 22:5 n-3]) on the following: CVD, cancer, child and maternal health, eye health, gastrointestinal/renal diseases, asthma, autoimmune diseases, immune-mediated diseases, transplantation, mental health, and neurological diseases and conditions. In addition to informing the research community and the public on the effects of omega-3 fatty acids on various health conditions, it is anticipated that the findings of the reports will also be used to help define the agenda for future research.
The focus of this report is on CVD risk factors and intermediate markers of CVD in humans. The other 2 reports by the Tufts-NEMC EPC focus on CVD outcomes in humans and on arrhythmic electrophysiology in animal and in-vitro studies. In this chapter, the metabolism, physiological functions, and the sources of omega-3 fatty acids are briefly discussed. Subsequent chapters describe the methods used to identify and review studies related to omega-3 fatty acids and CVD - including the analytic framework for this report, findings related to the effects of omega-3 fatty acids on cardiovascular conditions, and recommendations for future research in this area.
Background
Metabolism and Biological Effects of Essential Fatty Acids
Dietary fat is an important source of energy for biological activities in human beings. Dietary fat encompasses saturated fatty acids, which are usually solid at room temperature, and unsaturated fatty acids, which are liquid at room temperature. Unsaturated fatty acids can be further divided into monounsaturated and polyunsaturated fatty acids. Polyunsaturated fatty acids can be classified on the basis of their chemical structure into two groups: omega-3 (n-3) fatty acids and omega-6 (n-6) fatty acids. The omega-3 or n-3 notation means that the first double bond from the methyl end of the molecule is in the third. The same principle applies to the omega-6 or n-6 notation. Despite their differences in structure, all fats contain the same amount of energy (9 kcal/g or 37 kJ/g).
Of all fats found in food, 2 — ALA and linoleic acid (LA, 18:2 n-6) — cannot be synthesized in the human body, yet are necessary for proper physiological functioning. These 2 fats are called essential fatty acids. The essential fatty acids can be converted in the liver to long-chain polyunsaturated fatty acids, which have a higher number of carbon atoms and double bonds. These long-chain polyunsaturated fatty acids retain the omega type (n-3 or n-6) of the parent essential fatty acids.
ALA and LA comprise the bulk of the total polyunsaturated fatty acids consumed in a typical North American diet. Typically, LA comprises 89% of the total polyunsaturated fatty acids consumed, while ALA comprises 9%. Smaller amounts of other polyunsaturated fatty acids make up the remainder 1. Both ALA and LA are present in a variety of foods. For example, LA is present in high concentrations in many commonly used oils, including safflower, sunflower, soy, and corn oil. ALA, which is consumed in smaller quantities, is present in leafy green vegetables and in some commonly used oils, including canola and soybean oil. Some novelty oils, such as flaxseed oil, contain relatively high concentrations of ALA, but these oils are not commonly found in the food supply.
The Institute of Medicine suggests that, for adults 19 and older, an adequate intake (AI) of ALA is 1.1–1.6 g/day, while an adequate daily intake of LA is 11–17 g/day 2. Recommendations regarding AI differ by age and gender groups, and for special conditions such as pregnancy and lactation.
As shown in Figure 1.1, EPA and DHA can act as competitors for the same metabolic pathways as AA. In human studies, the analyses of fatty-acid compositions in both blood phospholipids and adipose tissue showed similar competitive relationship between omega-3 long-chain polyunsaturated fatty acids and AA. General scientific agreement supports an increased consumption of omega-3 fatty acids and reduced intake of omega-6 fatty acids to promote good health. However, for omega-3 fatty acid intakes, the specific quantitative recommendations vary widely among countries not only in terms of different units — ratio, grams, total energy intake — but also in quantity 3. Furthermore, there remain numerous questions relating to the inherent complexities about omega-3 and omega-6 fatty acid metabolism, in particular regarding the inter-relationships between the 2 fatty acids. For example, it remains unclear to what extend ALA is converted to EPA and DHA in humans, and to what extend high intake of omega-6 fatty acids compromises any benefits of omega-3 fatty acid consumption. Without resolution of these 2 foundational questions, it remains difficult to study the importance of omega-6 to omega-3 fatty acid ratio.
Metabolic Pathways of Omega-3 and Omega-6 Fatty Acids
Omega-3 and omega-6 fatty acids share the same pools of enzymes and go through the same oxidation pathways while being metabolized (Figure 1.1). Once ingested, ALA and LA can be elongated and desaturated into long-chain polyunsaturated fatty acids. LA is converted into gamma-linolenic acid (GLA, 18:3 n-6), an omega-6 fatty acid that is a positional isomer of ALA. GLA, in turn, can be converted to the long-chain omega-6 fatty acid, arachidonic acid (AA, 20:4 n-6). ALA can be converted, to a lesser extent, to the long-chain omega-3 fatty acids, EPA and DHA. However, the conversion from parent fatty acids into long-chain polyunsaturated fatty acids occurs slowly in humans, and conversion rates are not well understood. Because of the slow rate of conversion and the importance of long-chain polyunsaturated fatty acids to many physiological processes, humans must augment their level of long-chain polyunsaturated fatty acids by consuming foods that are rich in these important compounds. Meat is the primary food source of AA, while fish is the primary food source of EPA.
The specific biological functions of fatty acids depend on the number and position of double bonds and the length of the acyl chain. Both EPA and AA are 20-carbon fatty acids and are precursors for the formation of prostaglandins, thromboxane, and leukotrienes — hormone-like agents that are members of a larger family of substances called eicosanoids. Eicosanoids are localized tissue hormones that seem to be one of the fundamental regulatory classes of molecules in most higher forms of life. They do not travel in the blood, but are created in the cells to regulate a large number of processes, including the movement of calcium and other substances into and out of cells, dilation and contraction of muscles, inhibition and promotion of clotting, regulation of secretions including digestive juices and hormones, and control of fertility, cell division, and growth 4.
As shown in Figure 1.1, the long-chain omega-6 fatty acid, AA, is the precursor of a group of eicosanoids including series-2 prostaglandins and series-4 leukotrienes. The omega-3 fatty acid, EPA, is the precursor to a group of eicosanoids including series-3 prostaglandins and series-5 leukotrienes. The series-2 prostaglandins and series-4 leukotrienes derived from AA are involved in intense actions (such as accelerating platelet aggregation and enhancing vasoconstriction and the synthesis of inflammatory mediators) in response to physiological stressors. The series-3 prostaglandins and series-5 leukotrienes that are derived from EPA are less physiologically potent than those derived from AA. More specifically, the series-3 prostaglandins are formed at a slower rate and work to attenuate excessive series-2 prostaglandins. Thus, adequate production of the series-3 prostaglandins, which are derived from the omega-3 fatty acid, EPA, may protect against heart attack and stroke as well as certain inflammatory diseases like arthritis, lupus, and asthma 4. In addition, animal studies, have demonstrated that omega-3 fatty acids, such as EPA and DHA, engage in multiple cytoprotective activities that may contribute to antiarrhythmic mechanisms 5. Arrhythmias are a common cause of “sudden death” in heart disease.
In addition to affecting eicosanoid production as described above, EPA also affects lipoprotein metabolism and decreases the production of other compounds - including cytokines, interleukin 1ß (IL), and tumor necrosis factor a (TNF-a) - that have pro-inflammatory effects. These compunds exert pro-inclammatory cellular actions that include stimulating the production of collagenases and inreasing the expression of adhesion molecules necessary for leukocyte extravasation 6. The mechanism responsible for the suppression of cytokine production by omega-3 fatty acids remains unknown, although suppression of eicosanoid production by omega-3 fatty acids may be involved. EPA can also be converted into the longer chain omega-3 form of DPA, and then further elongated and oxygenated into DHA. EPA and DHA are frequently referred to as very long chain omega-3 fatty acids. DHA, which is thought to be important for brain development and functioning, is present in significant amounts in a variety of food products, including fish, fish liver oils, fish eggs, and organ meats. Similarly, AA can convert into an omega-6 form of DPA. Studies have reported that omega-3 fatty acids decrease triglycerides (Tg) and very low density lipoprotein (VLDL) in hypertriglyceridemic subjects, with a concomitant increase in high density lipoprotein (HDL). However, they appear to increase or have no effect on low density lipoprotein (LDL). Omega-3 fatty acids apparently lower Tg by inhibiting VLDL and apolipoprotein B-100 synthesis and decreasing post-prandial lipemia 7. Omega-3 fatty acids, in conjunction with transcription factors (small proteins that bind to the regulatory domains of genes), target the genes governing cellular Tg production and those activating oxidation of excess fatty acids in the liver. Inhibition of fatty acid synthesis and increased fatty acid catabolism reduce the amount of substrate available for Tg production 8.
As noted earlier, omega-6 fatty acids are consumed in larger quantities (>10 times) than omega-3 fatty acids. Maintaining a sufficient intake of omega-3 fatty acids is particularly important since many of the body's physiologic properties depend upon their availability and metabolism.
Population Intake of Omega-3 Fatty Acids in the United States
The major source of omega-3 fatty acids is dietary intake of fish, fish oil, vegetable oils (principally canola and soybean), some nuts including walnuts, and dietary supplements. Two population-based surveys, the third National Health and Nutrition Examination (NHANES III) 1988-94 and the Continuing Food Survey of Intakes by Individuals 1994-98 (CSFII) surveys, are the main source of dietary intake data for the U.S. population. NHANES III collected information on the U.S. population aged =2 months. Mexican Americans and non-Hispanic African-Americans, children =5 years old, and adults = 60 years old were over-sampled to produce more precise estimates for these population groups. There were no imputations for missing 24-hour dietary recall data. A total of 29,105 participants had complete and reliable dietary recall. Complete descriptions of the methods used and fuller analyses are available in the report Effects of Omega-3 Fatty Acids on Cardiovascular Disease, under “Methods: Method to Assess the Dietary Intake of Omega-3 Fatty Acids in the US population” and “Results: Population Intake of Omega-3 Fatty Acids in the United States”. CSFII 1994-96, popularly known as the What We Eat in America survey, addressed the requirements of the National Nutrition Monitoring and Related Research Act of 1990 (Public Law 101–445) for continuous monitoring of the dietary status of the American population. In CSFII 1994-96, an improved data-collection method known as the multiple-pass approach for the 24-hour recall was used. Given the large variation in intake from day-to-day, multiple 24-hours recalls are considered to be the best suited for most nutrition monitoring and will produce stable estimates of mean nutrient intakes from groups of individuals 9. In 1998, the Supplemental Children's Survey, a survey of food and nutrient intake by children under age of 10, was conducted as the supplement to the CSFII 1994-96. The CSFII 1994-96, 1998 surveyed 20,607 people of all ages with over-sampling of low-income population (<130% of the poverty threshold). Dietary intake data by individuals of all ages were collected over 2 nonconsecutive days by use of two 1-day dietary recalls.
Table 1.1 reports the NHANES III survey mean intake ± the standard error of the mean (SEM), as well as, the median and range for each omega-3 fatty acid. Distributions of EPA, DPA, and DHA were very skewed; therefore, the means and standard errors of the means should be used and interpreted with caution. Table 1.2 reports the CSFII survey mean and median intakes for each omega-3 fatty acid, along with SEMs, as reported in Dietary Reference Intakes by the Institute of Medicine 2.
Dietary Sources of Omega-3 Fatty Acids
Omega-3 fatty acids can be found in many different sources of food, including fish, shellfish, some nuts, and various plant oils. Table 1.3 lists the amount of omega-3 fatty acids in some commonly consumed fish, shellfish, nuts, and edible oils, selected from the USDA website http://www.nal.usda.gov/fnic/foodcomp (accessed November 3, 2003; Finfish and Shellfish Products: sr16fg15.pdf; Fats and Oils: sr16fg04.pdf; and Nut and Seed Products: sr16fg12.pdf) 10
Relationship of Dietary Fat and Cardiovascular Disease
Numerous studies have examined the relationship between dietary fat and CVD. Early epidemiology studies noted very low cardiovascular mortality among the Greenland Inuit as compared to mainland Danes, even though both had very high fat diets 11–13. Studies in other populations with high fish intake, including South Pacific Islanders, Japanese, and people from the Mediterranean region, also generally found a low prevalence of CVD despite a prevalence of other risk factors, such as hypertension, similar to that found in other populations 14. However, some epidemiological studies reached the opposite conclusion. The Seven Countries Study, for example, found that coronary heart disease mortality was highest in Eastern Finland, where average fish intake was 60 g per day 15. This finding may in part be due to a positive association between fish consumption and both cigarette smoking and cholesterol levels in Finland; an association not seen in other countries.
The apparent paradox of low levels of CVD in people with high fat diets was explained by the high consumption of marine sources of very long chain, highly polyunsaturated omega-3 fatty acids 16. Since these early studies, hundreds of observational and clinical trials have been conducted to analyze the effect of both marine and plant sources of omega-3 fatty acids on CVD and a wide range of CVD risk factors and intermediate markers of CVD, and to define and explain the potential benefits of increased intake of the omega-3 fatty acids.
Omega-3 Fatty Acids and Cardiovascular Disease Risk Factors
A large number of putative risk factors for and intermediate markers of CVD exist, including markers for different aspects of CVD, markers for risk factors of CVD, and markers for other factors related to cardiovascular health. However, the relationship between most of these laboratory measurements and diagnostic tests and aspects of atherosclerosis such as inflammation, are generally unproven. The relationships between these factors and actual clinical disease and events are generally even more theoretical. Nevertheless, as the science of atherosclerosis advances, our understanding of these relationships is improving.
Several measurable factors are generally well accepted to be associated with risk of CVD. These include serum lipoproteins, blood pressure, diabetes mellitus, and related metabolic disorders. Improvement or suppression of these factors has been shown to reduce the risk of CVD. Inflammation is becoming accepted as a cause of atherogenesis, although potential treatments have yet to show reduction of cardiovascular events. Thrombosis and oxidation (free radicals) are also involved in atherogenesis, although their effect on the risk of CVD is less clear (except in people with specific hypercoagulable conditions). Several cardiovascular processes are also risk factors for cardiovascular events. These include atherogenesis, vascular dysfunction, arrhythmias, and cardiac dysfunction among others. These processes generally do not cause symptoms until they are fairly advanced. They may also be reversed, thus potentially reducing cardiovascular morbidity and mortality.
Both in trials and in patient care, surrogate markers for disease or risk of disease are useful measures for tracking people's health. Understanding how omega-3 fatty acids affect these various intermediate markers of CVD can help efforts to explain how omega-3 fatty acids affect clinical CVD. Understanding the relationship between omega-3 fatty acids and intermediate markers would also be helpful in determining who could most benefit (or could be most harmed) from adjusting omega-3 fatty acid intake, and would help efforts to track their effect on cardiovascular risk factors. The following sections briefly summarize the relationship between omega-3 fatty acids and selected risk factors for and intermediate markers of CVD.
Improvement of Lipoproteins
Elevated serum low density lipoprotein (LDL) and depressed high density lipoprotein (HDL), especially when accompanied by elevated triglycerides (Tg), are well-known risk factors for CVD. Studies have reported that omega-3 fatty acids decrease Tg and very low density lipoprotein (VLDL) in hypertriglyceridemic subjects, with a concomitant increase in HDL. However, they appear to increase or have no effect on LDL. Omega-3 fatty acids apparently lower Tg by inhibiting VLDL and apolipoprotein B-100 (apo B-100) synthesis and decreasing post-prandial lipemia 7. Omega-3 fatty acids, in conjunction with transcription factors (small proteins that bind to the regulatory domains of genes), target the genes governing cellular Tg production and those activating oxidation of excess fatty acids in the liver. Inhibition of fatty acid synthesis and increased fatty acid catabolism reduce the amount of substrate available for Tg production 8.
Numerous other lipids and associated proteins are involved in lipid metabolism and thus possibly in atherogenesis and CVD; although they are less commonly measured. These include, among others, lipoprotein (a) [Lp(a)]; apolipoproteins (apo) A-I, B-48, B-100, C-III; and free fatty acids.
Reduction of Thrombosis
Blockage of coronary, cerebral and peripheral vessels due to thrombosis is a leading cause of CVD. Omega-3 fatty acids affect the clotting system in a number of ways. EPA competes with AA for the cyclo-oxygenase enzyme, thus reducing thromboxane A2 (TX), a thrombotic agent. DHA may further inhibit cyclo-oxygenase 17. Omega-3 fatty acids also inhibit TXB2 production, platelet aggregation, and platelet adhesion, although much less so than aspirin. Omega-3 fatty acids also lead to endothelial formation of prostaglandin I3 (PG), PGI2, and nitrous oxide, all of which reduce vasoconstriction 17, 18. However, knowledge about the role of omega-3 fatty acids on coagulation factors and fibrinolysis is incomplete.
Many markers of coagulability exist, including the numerous factors involved in the clotting cascade, homocysteine, bleeding time, and platelet aggregation. Except among people with specific hypercoagulable conditions, it is not clear that any of these measures, among others, are predictive of CVD or that modification of their levels modifies risk of CVD.
Reduction of Inflammation, Atherogenesis, and Leukocyte Activity
Awareness of the effect of inflammation on atherogenesis (atheromatous plaque formation) and the risk of cardiovascular events is increasing. Leukocytes (white blood cells) are the blood cells that respond to injury or infection with a protective inflammatory response and an immune response. However, leukocytes are prominent cells in the atheromatous plaque in major blood vessels, which suggests that early plaque formation has an inflammatory component. PGE2 and leukotriene B4 (LT) have pro-inflammatory biological actions, and together they can cause vascular leakage and extravasation of fluid. The omega-6 fatty acid, AA, is the progenitor of both PGE2 and LTB4 via the cyclo-oxygenase and 5-lipo-oxygenase enzymatic pathways, respectively. EPA is the omega-3 homologue of AA; the 2 fatty acids differ only in that EPA has 1 additional double bond at the third carbon. EPA can thus inhibit AA metabolism competitively via the enzymatic pathways and can suppress production of the omega-6 fatty acid eicosanoid inflammatory mediators. Although EPA promotes the formation of PGE3 and LTB5, these eicosanoids are far less active as pro-inflammatory agents than the corresponding derivatives of AA 8. Furthermore, other pro-inclammatory factors, such as IL-1ß and TNF-a, can be suppressed by the effect of long-chain polynsaturated fatty acids on lipoprotein metabolism 6.
C-reactive protein (CRP) is a well-described marker of inflammation and rises in response to injury, infection, and other inflammatory stimuli. In patients with either angina or risk factors for atherosclerosis, increased CRP has been associated with increased relative risk of nonfatal myocardial infarction and overall cardiovascular mortality 19. It is unclear whether reduction in CRP would result in reduced risk of CVD. Trials commonly measure other inflammatory markers including IL-6 and vascular cell adhesion molecule 1 (VCAM-1). Less is known about their association with CVD.
Reduction of Arrhythmia
Cardiac arrhythmias can be fatal, causing sudden death, or can result in stroke, myocardial infarction, congestive heart failure, and peripheral embolisms, among other types of CVD. Animal studies have shown that fatal ventricular fibrillation could be essentially abolished by high-level feeding with omega-3 fatty acids 20. Omega-3 fatty acids appear to act in multiple ways to prevent arrhythmias. Various animal and in vitro experiments have shown that omega-3 fatty acids directly modulate sodium, potassium, and calcium channels 21. By incorporating into cell membrane phospholipids, the excitation-contraction coupling that can result in arrhythmia is reduced 22. Omega-3 fatty acids also modulate various intracellular enzymes involved in controlling the contraction and relaxation cycles of myocytes 23. EPA and DHA also affect adrenoceptors, membrane proteins whose function in the heart is to transmit the neuroendocrine message of the catecholamines (adrenaline and its derivatives) 24. The activity of DHA is thus similar in principle to that of ß-blockers, a group of key cardiovascular drugs used to decrease the cardiac effects of catecholamines. Omega-3 long-chain polyunsaturated fatty acids also appear to act similarly to another group of cardiovascular durgs, calcium channel blockers, by increasing intracellular calcium sequestration and interfering with receptor-operated calcium channels, thus lowering calcium influx 22. The effect of omega-3 fatty acids on prostanoids and leukotrienes also theoretically reduces the arrhythmia potential of cardiac myocytes.
The risk of ventricular arrhythmia is most commonly measured by 24 hour ambulatory electrocardiography recordings, in which a continuous electrocardiogram (ECG) is taken for generally 24 hours. Various measures of heart rate variability are calculated, primarily based on the standard deviation (SD) of the duration of time between heart beats. Other common ECG measurements are also followed as indicators or risk of arrhythmia or cardiac ischemia.
Blood Pressure
Hypertension is well recognized as one of the leading causes of CVD. The recent Joint National Committee report (JNC 7) emphasizes the risks of blood pressure that is even slightly elevated above 120/80 mm Hg 25. Lifestyle modification, including reduction of sodium and alcohol intake, weight loss, diets high in fruits and vegetables and low-fat dairy products, and exercise has been shown to reduce blood pressure, often as much as medication use. Early investigations into the way in which fatty fish consumption may lower CVD found that omega-3 fatty acids possibly reduce blood pressure 26. While the mechanisms for such an effect remain uncertain, the most compelling hypothesis is that by altering the balance between vasoconstrictive TXA2 and vasodilatory PGI3, as described in the section on inflammation, overall blood vessel capacitance increases and thus blood pressure falls 27. However, the baseline balance of vasoactive and regulatory hormones may be altered in people with frank hypertension or other types of CVD The question thus arises whether the effect of omega-3 intake on blood pressure is altered in people with hypertension.
Diabetes
Although long-chain omega-3 fatty acids appear to have an overall beneficial effect on CVD, their effect on glucose homeostasis is less clear. Omega-3 fatty acids may, in fact, have a detrimental effect on glucose tolerance 28. Theoretical benefits of omega-3 fatty acids to diabetic management include reducing Tg, increasing HDL, increasing glucose-induced insulin secretion, and possibly lowering insulin resistance 28, 29. However, omega-3 fatty acids may worsen glucose tolerance in patients with clear cut diabetes and may, in fact, worsen insulin resistance 28.
Thus, important questions relate to the level of markers of glucose tolerance, such as fasting blood glucose (FBS), glycohemoglobin or hemoglobin A1c (Hgb A1c), and fasting insulin levels, in people with both diabetes and insulin resistance and people without glucose tolerance impairment.
Cardiovascular Diagnostic Tests
The metabolic effects of omega-3 fatty acids on lipoproteins, thrombosis, inflammation, arrhythmia and blood pressure all have potential effects on blood vessels and the heart, which eventually can lead to clinical CVD. In addition, there are numerous diagnostic tests of cardiovascular health that are known to be predictive of future cardiovascular events both in people with and without a known history of CVD. Improvements in these diagnostic tests are commonly used as indicators of effective prophylaxis or treatment.
Among the tests of vascular health that have been assessed in omega-3 fatty acid trials are coronary arteriography (to measure coronary vessel stenosis), carotid intima-media thickness (IMT, which measures the thickness of the carotid artery wall, a measure of atherosclerosis), carotid Doppler ultrasonography or magnetic resonance arteriography (to measure carotid and extra-carotid stenosis), ankle brachial index (to measure peripheral blood flow), and endothelium-dependent vasorelaxation (an invasive or minimally invasive test of endothelial function). Other useful diagnostic tests measure heart function, including the exercise tolerance test (treadmill or stress test) and cardiac ultrasonography (which measures heart wall, chamber and valve structure and function).
Association of Omega-3 Fatty Acid Intake and Tissue Levels
The fatty acid composition of the cell membrane is a dynamic system, and the regulatory mechanisms are not fully understood. Since omega-3 fatty acids cannot be synthesized in the human body, the amount of total omega-3 fatty acids stored in adipose tissue is believed to be associated primarily with the amount of long-term omega-3 fatty acid dietary intake 30, while the amount incorporated into red blood cell membrane phospholipids is believed to be associated with short-term intake 31. Studies have consistently shown that populations whose diets are rich in fish (and thus omega-3 fatty acids) have relatively high omega-3 fatty acid content in plasma phospholipids 32–35. However, it remains less clear whether there is a reliable dose-response correlation between dietary omega-3 fatty acid intake and fatty acid profiles of plasma phospholipids, LDL fractions of serum phospholipids and cholesteryl esters, and blood cell phospholipids 36. Further, the metabolism from ALA - the main source of dietary omega-3 fatty acids - to its longer chain metabolites and then to eicosanoids is not well understood. Thus, the association between fatty acid intake and measurable tissue levels is not straightforward. Further complicating measurement estimates of total body stores of omega-3 fatty acids is that there are numerous measurable levels, including cell membrane phospholipids and triglycerides from the 3 major blood cell lines (erythrocytes, leukocytes and platelets), plasma triglycerides, plasma free fatty acids, and adipose cells. In addition, there is continuous movement of fatty acids between compartments, and each compartment incorporates fatty acids differently. As discussed above, under Metabolic Pathways of Omega-3 and Omega-6 Fatty Acids, omega-3 fatty acid metabolism is in part dependent on omega-6 fatty acid levels, further confounding associations between dietary intake and blood levels.
Figures
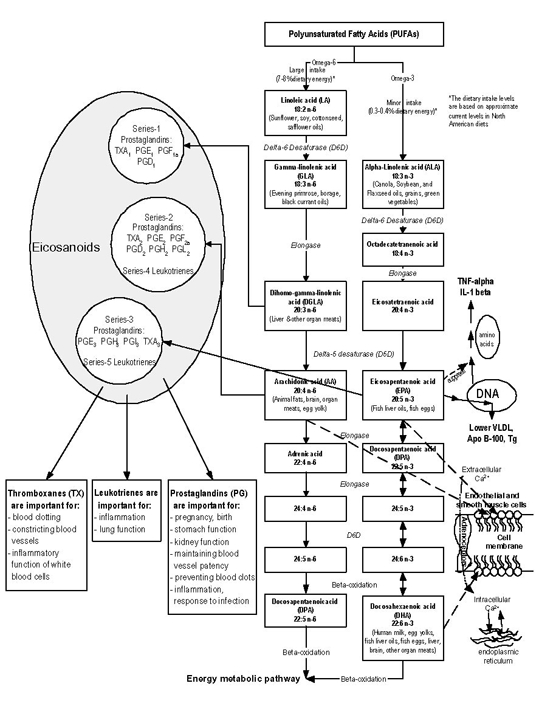
Figure 1.1 Classical omega-3 and omega-6 fatty acid synthesis pathways and the role of omega-3 fatty acid in regulating health/disease markers
Tables
Table 1.1 Estimates of the mean ± standard error of the mean (SEM) intake of linoleic acid (LA), alpha-linolenic acid (ALA), eicosapentaenoic acid (EPA), and docosahexaenoic acid (DHA) in the United States population, based on analyses of a single 24-hour dietary recall of NHANES III data
Grams/day | % Kcal/day | |||
---|---|---|---|---|
Mean±SEM | Median (range) a | Mean±SEM | Median (range) a | |
LA (18:2 n-6) | 14.1±0.2 | 9.9 (0 – 168) | 5.79±0.05 | 5.30 (0 – 39.4) |
ALA (18:3 n-3) | 1.33±0.02 | 0.90 (0 – 17) | 0.55±0.004 | 0.48 (0 – 4.98) |
EPA (20:5 n-3) | 0.04±0.003 | 0.00 (0 – 4.1) | 0.02±0.001 | 0.00 (0 – 0.61) |
DHA (22:6 n-3) | 0.07±0.004 | 0.00 (0 – 7.8) | 0.03±0.002 | 0.00 (0 –2.86) |
- a
The distributions are not adjusted for the over-sampling of Mexican Americans, non-Hispanic African-Americans, children ≤ 5 years old, and adults ≥ 60 years old in the NHANES III dataset.
Table 1.2 Mean, range, median, and standard error of the mean (SEM) of usual daily intakes of linoleic acid (LA), total omega-3 fatty acids (n-3 FA), alpha-linolenic acid (ALA), eicosapentaenoic acid (EPA), docosapentaenoic acid (DPA) and docosahexaenoic acid (DHA) in the US population, based on CSFII data (1994-1996, 1998)
Grams/day | ||
---|---|---|
Mean±SEM | Median±SEM | |
LA (18:2 n-6) | 13.0±0.1 | 12.0±0.1 |
Total n-3 FA | 1.40±0.01 | 1.30±0.01 |
ALA (18:3 n-3) | 1.30±0.01 | 1.21±0.01 |
EPA (20:5 n-3) | 0.028 | 0.004 |
DPA (22:5 n-3) | 0.013 | 0.005 |
DHA (22:6 n-3) | 0.057±0.018 | 0.046±0.013 |
Table 1.3 The omega-3 fatty acid content, in grams per 100 g food serving, of a representative sample of commonly consumed fish, shellfish, and fish oils, and nuts and seeds, and plant oils that contain at least 5 g omega-3 fatty acids per 100 g (from USDA website http://www.nal.usda.gov/fnic/foodcomp, 2003)
Food item | EPA | DHA | ALA |
---|---|---|---|
Fish (Rawa) | |||
Anchovy, European | 0.6 | 0.9 | - |
Bass, Freshwater, Mixed Sp. | 0.2 | 0.4 | 0.1 |
Bass, Striped | 0.2 | 0.6 | trace |
Bluefish | 0.2 | 0.5 | - |
Carp | 0.2 | 0.1 | 0.3 |
Catfish, Channel | trace | 0.2 | 0.1 |
Cod, Atlantic | trace | 0.1 | trace |
Cod, Pacific | trace | 0.1 | trace |
Eel, Mixed Sp. | trace | trace | 0.4 |
Flounder & Sole Sp. | trace | 0.1 | trace |
Grouper, Mixed Sp. | trace | 0.2 | trace |
Haddock | trace | 0.1 | trace |
Halibut, Atlantic and Pacific | trace | 0.3 | trace |
Halibut, Greenland | 0.5 | 0.4 | trace |
Herring, Atlantic | 0.7 | 0.9 | 0.1 |
Herring, Pacific | 1.0 | 0.7 | trace |
Mackerel, Atlantic | 0.9 | 1.4 | 0.2 |
Mackerel, Pacific and Jack | 0.6 | 0.9 | trace |
Mullet, Striped | 0.2 | 0.1 | trace |
Ocean Perch, Atlantic | trace | 0.2 | trace |
Pike, Northern | trace | trace | trace |
Pike, Walleye | trace | 0.2 | trace |
Pollock, Atlantic | trace | 0.4 | - |
Pompano, Florida | 0.2 | 0.4 | - |
Roughy, Orange | trace | - | trace |
Salmon, Atlantic, Farmed | 0.6 | 1.3 | trace |
Salmon, Atlantic, Wild | 0.3 | 1.1 | 0.3 |
Salmon, Chinook | 1.0 | 0.9 | trace |
Salmon, Chinook, Smokedb | 0.2 | 0.3 | - |
Salmon, Chum | 0.2 | 0.4 | trace |
Salmon, Coho, Farmed | 0.4 | 0.8 | trace |
Salmon, Coho, Wild | 0.4 | 0.7 | 0.2 |
Salmon, Pink | 0.4 | 0.6 | trace |
Salmon, Pink, Cannedc | 0.9 | 0.8 | trace |
Salmon, Sockeye | 0.6 | 0.7 | trace |
Sardine, Atlantic, Canned in Oild | 0.5 | 0.5 | 0.5 |
Seabass, Mixed Sp. | 0.2 | 0.4 | - |
Seatrout, Mixed Sp. | 0.2 | 0.2 | trace |
Shad, American | 1.1 | 1.3 | 0.2 |
Shark, Mixed Sp. | 0.3 | 0.5 | trace |
Snapper, Mixed Sp. | trace | 0.3 | trace |
Swordfish | 0.1 | 0.5 | 0.2 |
Trout, Mixed Sp. | 0.2 | 0.5 | 0.2 |
Trout, Rainbow, Farmed | 0.3 | 0.7 | trace |
Trout, Rainbow, Wild | 0.2 | 0.4 | 0.1 |
Tuna, Fresh, Bluefin | 0.3 | 0.9 | - |
Tuna, Fresh, Skipjack | trace | 0.2 | - |
Tuna, Fresh, Yellowfin | trace | 0.2 | trace |
Tuna, Light, Canned in Oile | trace | 0.1 | trace |
Tuna, Light, Canned in Watere | trace | 0.2 | trace |
Tuna, White, Canned in Oile | trace | 0.2 | 0.2 |
Tuna, White, Canned in Watere | 0.2 | 0.6 | trace |
Whitefish, Mixed Sp. | 0.3 | 0.9 | 0.2 |
Whitefish, Mixed Sp., Smoked | trace | 0.2 | - |
Wolffish, Atlantic | 0.4 | 0.3 | trace |
Shellfish (Raw) | |||
Abalone, Mixed Sp. | trace | - | - |
Clam, Mixed Sp. | trace | trace | trace |
Crab, Blue | 0.2 | 0.2 | - |
Crayfish, Mixed Sp., Farmed | trace | 0.1 | trace |
Lobster, Northern | - | - | - |
Mussel, Blue | 0.2 | 0.3 | trace |
Oyster, Eastern, Farmed | 0.2 | 0.2 | trace |
Oyster, Eastern, Wild | 0.3 | 0.3 | trace |
Oyster, Pacific | 0.4 | 0.3 | trace |
Scallop, Mixed Sp. | trace | 0.1 | - |
Shrimp, Mixed Sp. | 0.3 | 0.2 | trace |
Squid, Mixed Sp. | 0.1 | 0.3 | trace |
Fish Oils | |||
Cod Liver Oil | 6.9 | 11.0 | 0.9 |
Herring Oil | 6.3 | 4.2 | 0.8 |
Menhaden Oil | 13.2 | 8.6 | 1.5 |
Salmon Oil | 13.0 | 18.2 | 1.1 |
Sardine Oil | 10.1 | 10.7 | 1.3 |
Nuts and Seeds | |||
Butternuts, Dried | - | - | 8.7 |
Flaxseed | 18.1 | ||
Walnuts, English | - | - | 9.1 |
Plant Oils | |||
Canola (Rapeseed) | - | - | 9.3 |
Flaxseed Oil | - | - | 53.3 |
Soybean Lecithin Oil | - | - | 5.1 |
Soybean Oil | - | - | 6.8 |
Walnut Oil | - | - | 10.4 |
Wheatgerm Oil | - | - | 6.9 |
trace = <0.1; - = 0 or no data; Sp. = species.
- a
Except as indicated.
- b
Lox.
- c
Solids with bone and liquid.
- d
Drained solids with bone.
- e
Drained solids.