NCBI Bookshelf. A service of the National Library of Medicine, National Institutes of Health.
PDQ Cancer Information Summaries [Internet]. Bethesda (MD): National Cancer Institute (US); 2002-.
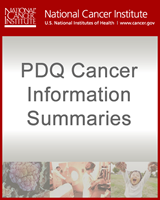
PDQ Cancer Information Summaries [Internet].
Show detailsThis PDQ cancer information summary for health professionals provides comprehensive, peer-reviewed, evidence-based information about the treatment of childhood myelodysplastic neoplasms. It is intended as a resource to inform and assist clinicians in the care of their patients. It does not provide formal guidelines or recommendations for making health care decisions.
This summary is reviewed regularly and updated as necessary by the PDQ Pediatric Treatment Editorial Board, which is editorially independent of the National Cancer Institute (NCI). The summary reflects an independent review of the literature and does not represent a policy statement of NCI or the National Institutes of Health (NIH).
General Information About Childhood Myelodysplastic Neoplasms (MDS)
The myelodysplastic neoplasms (MDS) and myeloproliferative neoplasms (MPN) represent between 5% and 10% of all myeloid malignancies in children. They are a heterogeneous group of disorders. MDS usually presents with cytopenias and is characterized by ineffective hematopoiesis and increased cell death. MPN presents with increased peripheral white blood cell, red blood cell, or platelet counts, and it is associated with increased progenitor cell proliferation and survival. Because both types of syndromes represent disorders of very primitive, multipotential hematopoietic stem cells, curative therapeutic approaches nearly always require allogeneic hematopoietic stem cell transplant.
For information about therapy-related MDS, see the Therapy-Related AML and Therapy-Related Myelodysplastic Neoplasms section in Childhood Acute Myeloid Leukemia Treatment.
For information about MDS associated with GATA1 mutations in children with Down syndrome who are aged 4 years or younger, see Childhood Myeloid Proliferations Associated with Down Syndrome Treatment.
For information about MPN, see Childhood Chronic Myeloid Leukemia Treatment and Juvenile Myelomonocytic Leukemia Treatment.
Clinical Presentation
Patients with myelodysplastic neoplasms (MDS) often present with signs of cytopenias, including pallor, infection, or bruising.
The bone marrow is usually characterized by hypercellularity and dysplastic changes of 10% or more in one or more precursor lineages. Clonal evolution can eventually lead to the development of acute myeloid leukemia (AML). The percentage of abnormal blasts is less than 20%, and they lack common AML recurrent cytogenetic abnormalities (e.g., t(8;21), inv(16), t(15;17), or KMT2A translocations).
The less common hypocellular MDS can be distinguished from aplastic anemia in part by its marked dysplasia, clonal nature, and higher percentage of CD34-positive precursors.[1,2]
References
- Kasahara S, Hara T, Itoh H, et al.: Hypoplastic myelodysplastic syndromes can be distinguished from acquired aplastic anaemia by bone marrow stem cell expression of the tumour necrosis factor receptor. Br J Haematol 118 (1): 181-8, 2002. [PubMed: 12100146]
- Orazi A: Histopathology in the diagnosis and classification of acute myeloid leukemia, myelodysplastic syndromes, and myelodysplastic/myeloproliferative diseases. Pathobiology 74 (2): 97-114, 2007. [PubMed: 17587881]
Risk Factors
Patients with the following germline mutations or inherited disorders have a significantly increased risk of developing myelodysplastic neoplasms (MDS):
- Fanconi anemia: Caused by germline mutations in DNA repair genes.
- Telomere biology disorders (e.g., dyskeratosis congenita): Resulting from mutations in genes regulating telomere length. Genes mutated in dyskeratosis congenita include ACD, CTC1, DKC1, NHP2, NOP10, PARN, RTEL1, TERC, TERT, TINF2, and WRAP53.
- Severe congenital neutropenia: Caused by mutations in the gene encoding elastase. The 15-year cumulative risk of MDS in patients with severe congenital neutropenia, also known as Kostmann syndrome, has been estimated to be 15%, with an annual risk of MDS/acute myeloid leukemia (AML) of 2% to 3%. It is unclear how mutations affecting this protein and how the chronic exposure of granulocyte colony-stimulating factor (G-CSF) contribute to the development of MDS.[4]
- Trisomy 21 syndrome: GATA1 mutations are nearly always present in the transient leukemia associated with Trisomy 21 and MDS in children younger than 3 years with Down syndrome.[5]
A retrospective analysis was performed on genomic DNA from peripheral blood mononuclear cell samples from patients undergoing hematopoietic stem cell transplant for MDS and aplastic anemia. The analysis used a capture assay to target mutations known to predispose to bone marrow failure and MDS. Among the 46 children aged 18 years and younger with MDS, 10 patients (22%) harbored constitutional predisposition genetic mutations (5 GATA2, 1 each of MPL, RTEL1, SBDS, TINF2, and TP53). Only two of these patients were clinically suspected of having genetic mutations before their transplants. Children in this study had a higher incidence of genetic mutations (22%) than adults aged 18 to 40 years (8%).[17]
References
- Alter BP, Giri N, Savage SA, et al.: Malignancies and survival patterns in the National Cancer Institute inherited bone marrow failure syndromes cohort study. Br J Haematol 150 (2): 179-88, 2010. [PMC free article: PMC3125983] [PubMed: 20507306]
- Rosenberg PS, Huang Y, Alter BP: Individualized risks of first adverse events in patients with Fanconi anemia. Blood 104 (2): 350-5, 2004. [PubMed: 15059844]
- Ludwig LS, Gazda HT, Eng JC, et al.: Altered translation of GATA1 in Diamond-Blackfan anemia. Nat Med 20 (7): 748-53, 2014. [PMC free article: PMC4087046] [PubMed: 24952648]
- Rosenberg PS, Zeidler C, Bolyard AA, et al.: Stable long-term risk of leukaemia in patients with severe congenital neutropenia maintained on G-CSF therapy. Br J Haematol 150 (2): 196-9, 2010. [PMC free article: PMC2906693] [PubMed: 20456363]
- Wechsler J, Greene M, McDevitt MA, et al.: Acquired mutations in GATA1 in the megakaryoblastic leukemia of Down syndrome. Nat Genet 32 (1): 148-52, 2002. [PubMed: 12172547]
- Liew E, Owen C: Familial myelodysplastic syndromes: a review of the literature. Haematologica 96 (10): 1536-42, 2011. [PMC free article: PMC3186316] [PubMed: 21606161]
- Owen C, Barnett M, Fitzgibbon J: Familial myelodysplasia and acute myeloid leukaemia--a review. Br J Haematol 140 (2): 123-32, 2008. [PubMed: 18173751]
- Ghauri RI, Naveed M, Mannan J: Congenital amegakaryocytic thrombocytopenic purpura (CAMT). J Coll Physicians Surg Pak 24 (4): 285-7, 2014. [PubMed: 24709246]
- Auer PL, Teumer A, Schick U, et al.: Rare and low-frequency coding variants in CXCR2 and other genes are associated with hematological traits. Nat Genet 46 (6): 629-34, 2014. [PMC free article: PMC4050975] [PubMed: 24777453]
- Vinh DC, Patel SY, Uzel G, et al.: Autosomal dominant and sporadic monocytopenia with susceptibility to mycobacteria, fungi, papillomaviruses, and myelodysplasia. Blood 115 (8): 1519-29, 2010. [PMC free article: PMC2830758] [PubMed: 20040766]
- Schwartz JR, Ma J, Lamprecht T, et al.: The genomic landscape of pediatric myelodysplastic syndromes. Nat Commun 8 (1): 1557, 2017. [PMC free article: PMC5691144] [PubMed: 29146900]
- Schwartz JR, Wang S, Ma J, et al.: Germline SAMD9 mutation in siblings with monosomy 7 and myelodysplastic syndrome. Leukemia 31 (8): 1827-1830, 2017. [PMC free article: PMC5540771] [PubMed: 28487541]
- Davidsson J, Puschmann A, Tedgård U, et al.: SAMD9 and SAMD9L in inherited predisposition to ataxia, pancytopenia, and myeloid malignancies. Leukemia 32 (5): 1106-1115, 2018. [PMC free article: PMC5940635] [PubMed: 29535429]
- Narumi S, Amano N, Ishii T, et al.: SAMD9 mutations cause a novel multisystem disorder, MIRAGE syndrome, and are associated with loss of chromosome 7. Nat Genet 48 (7): 792-7, 2016. [PubMed: 27182967]
- Chen DH, Below JE, Shimamura A, et al.: Ataxia-Pancytopenia Syndrome Is Caused by Missense Mutations in SAMD9L. Am J Hum Genet 98 (6): 1146-1158, 2016. [PMC free article: PMC4908176] [PubMed: 27259050]
- Wong JC, Bryant V, Lamprecht T, et al.: Germline SAMD9 and SAMD9L mutations are associated with extensive genetic evolution and diverse hematologic outcomes. JCI Insight 3 (14): , 2018. [PMC free article: PMC6124395] [PubMed: 30046003]
- Keel SB, Scott A, Sanchez-Bonilla M, et al.: Genetic features of myelodysplastic syndrome and aplastic anemia in pediatric and young adult patients. Haematologica 101 (11): 1343-1350, 2016. [PMC free article: PMC5394862] [PubMed: 27418648]
Molecular Abnormalities
Molecular features of myelodysplastic neoplasms (MDS)
Compared with MDS in adults, pediatric MDS is associated with a distinctive constellation of genetic alterations. In adults, MDS often evolves from clonal hematopoiesis and is characterized by mutations in TET2, DNMT3A, DDX41 and TP53. In contrast, mutations in these genes are rare in pediatric MDS, while mutations in GATA2, SAMD9, SAMD9L, ETV6, SETBP1, ASXL1, and RAS/MAPK pathway genes are observed in subsets of children with MDS.[1,2]
A report of the genomic landscape of pediatric MDS described the results of whole-exome sequencing for 32 pediatric patients with primary MDS and targeted sequencing for another 14 cases.[1] These 46 cases were equally divided between childhood MDS with low blasts (cMDS-LB) (previously called refractory cytopenia of childhood) and childhood MDS with increased blasts (cMDS-IB) (previously called MDS with excess blasts [MDS-EB)]). The results from the report include the following:
- Mutations in RAS/MAPK pathway genes were observed in 43% of primary MDS cases, with mutations most commonly involving PTPN11 and NRAS but with mutations also observed in other pathway members (e.g., BRAF [non–BRAF V600E], CBL, and KRAS). RAS/MAPK mutations were more common in patients with cMDS-IB (65%) than in patients with cMDS-LB (17%).
- Germline variants in SAMD9 (n = 4) or SAMD9L (n = 4) were observed in 17% of patients with primary MDS, with seven of eight mutations occurring in patients with cMDS-LB. These cases all showed loss of material on chromosome 7. Approximately 40% of patients with deletions of part or all of chromosome 7 had germline SAMD9 or SAMD9L variants.
- GATA2 mutations were observed in three cases (7%), and all cases were confirmed or presumed to be germline.
- Deletions involving chromosome 7 were the most common copy number alteration and were observed in 41% of cases. Loss of part or all of chromosome 7 was most commonly observed in SAMD9 and SAMD9L cases (100%) and in cMDS-IB patients with a RAS/MAPK mutation (71%).
- Other genes that were mutated in more than 1 of the 46 cases studied included SETBP1, ETV6, and TP53.
A second report described the application of a targeted sequencing panel of 105 genes to 50 pediatric patients with MDS (cMDS-LB = 31 and cMDS-IB = 19) and was enriched for cases with monosomy 7 (48%).[1,2] SAMD9 and SAMD9L were not included in the gene panel. The second report described the following results:
- Germline GATA2 mutations were observed in 30% of patients, and RUNX1 mutations were observed in 6% of patients.
- Somatic mutations were observed in 34% of patients and were more common in patients with cMDS-IB than in patients with cMDS-LB (68% vs. 13%).
- The most commonly mutated gene was SETBP1 (18%); less commonly mutated genes included ASXL1, RUNX1, and RAS/MAPK pathway genes (PTPN11, NRAS, KRAS, NF1). Twelve percent of cases showed mutations in RAS/MAPK pathway genes.
Patients with germline GATA2 mutations, in addition to MDS, show a wide range of hematopoietic and immune defects as well as nonhematopoietic manifestations.[3] The former defects include monocytopenia with susceptibility to atypical mycobacterial infection and DCML deficiency (loss of dendritic cells, monocytes, and B and natural killer lymphoid cells). The resulting immunodeficiency leads to increased susceptibility to warts, severe viral infections, mycobacterial infections, fungal infections, and human papillomavirus–related cancers. The nonhematopoietic manifestations include deafness and lymphedema.
Germline GATA2 mutations were studied in 426 pediatric patients with primary MDS and 82 cases with secondary MDS who were enrolled in consecutive studies of the European Working Group of MDS in Childhood (EWOG-MDS).[4] The study had the following results:
- Germline GATA2 mutations were identified in 7% of pediatric patients with primary MDS. While the median age of patients presenting with GATA2 mutations was 12.3 years in the EWOG-MDS pediatric population, most cases of germline GATA2-related myeloid neoplasms occur during adulthood.[5]
- GATA2 mutations were more common in patients with cMDS-IB (15%) than in patients with cMDS-LB (4%).
- Among patients with GATA2 mutations, 46% presented with cMDS-IB, and 70% showed monosomy 7.
- Familial MDS/acute myeloid leukemia (AML) was identified in 12 of 53 patients with GATA2 mutations for whom detailed family histories were available.
- Nonhematologic phenotypes of GATA2 deficiency were present in 51% of patients with MDS who had GATA2 mutations and included deafness (9%), lymphedema/hydrocele (23%), and immunodeficiency (39%).
SAMD9 and SAMD9L germline mutations are both associated with pediatric MDS cases in which there is an additional loss of all or part of chromosome 7.[6,7]
In 2016, SAMD9 was identified as the cause of the MIRAGE syndrome (myelodysplasia, infection, restriction of growth, adrenal hypoplasia, genital phenotypes, and enteropathy), which is associated with early-onset MDS with monosomy 7.[8] Subsequently, mutations in SAMD9L were identified in patients with ataxia pancytopenia syndrome (ATXPC; OMIM 159550). SAMD9 and SAMD9L mutations were also identified as the cause of the myelodysplasia and leukemia syndrome with monosomy 7 (MLSM7; OMIM 252270),[9] a syndrome first identified in phenotypically normal siblings who developed MDS or AML associated with monosomy 7 during childhood.[10]
- Both SAMD9 and SAMD9L are located at chromosome 7q21.2. Cases of MDS in patients with SAMD9 or SAMD9L mutations often show monosomy 7, with the remaining chromosome 7 having wild-type SAMD9 and SAMD9L. This results in the loss of the enhanced growth-suppressing activity of the mutated gene.
- Phenotypically normal patients with SAMD9 or SAMD9L mutations and monosomy 7 may progress to MDS or AML or, alternatively, may show loss of their monosomy 7 with a return of normal hematopoiesis.[10] The former outcome is associated with the acquisition of mutations in genes associated with MDS/AML (e.g., ETV6 or SETBP1). The latter outcome is associated with genetic alterations (e.g., revertant mutations or copy-neutral loss of heterozygosity with retention of the wild-type allele) that result in normalization of SAMD9 or SAMD9L activity. These observations suggest that monitoring patients with SAMD9- or SAMD9L-related monosomy 7, using clinical sequencing for acquired mutations in genes associated with progression to AML, may identify those at high risk of leukemic transformation. Such patients may benefit most from hematopoietic stem cell transplant.[10]
The presence of an isolated monosomy 7 is the most common cytogenetic abnormality, although it does not appear to portend a poor prognosis, compared with its presence in overt AML. However, the presence of monosomy 7 in combination with other cytogenetic abnormalities is associated with a poor prognosis.[11,12] The relatively common abnormalities of -Y, 20q-, and 5q- in adults with MDS are rare in childhood MDS. The presence of cytogenetic abnormalities that are found in AML (t(8;21)(q22;q22.1), inv(16)(p13.1;q22) or t(16;16)(p13.1;q22), and APL with PML::RARA gene fusions) defines disease that should be treated as AML and not MDS, regardless of blast percentage. The World Health Organization (WHO) notes that whether this should also apply to other recurring genetic abnormalities remains controversial.[13]
References
- Schwartz JR, Ma J, Lamprecht T, et al.: The genomic landscape of pediatric myelodysplastic syndromes. Nat Commun 8 (1): 1557, 2017. [PMC free article: PMC5691144] [PubMed: 29146900]
- Pastor V, Hirabayashi S, Karow A, et al.: Mutational landscape in children with myelodysplastic syndromes is distinct from adults: specific somatic drivers and novel germline variants. Leukemia 31 (3): 759-762, 2017. [PubMed: 27876779]
- Collin M, Dickinson R, Bigley V: Haematopoietic and immune defects associated with GATA2 mutation. Br J Haematol 169 (2): 173-87, 2015. [PMC free article: PMC4409096] [PubMed: 25707267]
- Wlodarski MW, Hirabayashi S, Pastor V, et al.: Prevalence, clinical characteristics, and prognosis of GATA2-related myelodysplastic syndromes in children and adolescents. Blood 127 (11): 1387-97; quiz 1518, 2016. [PubMed: 26702063]
- Wlodarski MW, Collin M, Horwitz MS: GATA2 deficiency and related myeloid neoplasms. Semin Hematol 54 (2): 81-86, 2017. [PMC free article: PMC5650112] [PubMed: 28637621]
- Davidsson J, Puschmann A, Tedgård U, et al.: SAMD9 and SAMD9L in inherited predisposition to ataxia, pancytopenia, and myeloid malignancies. Leukemia 32 (5): 1106-1115, 2018. [PMC free article: PMC5940635] [PubMed: 29535429]
- Schwartz JR, Wang S, Ma J, et al.: Germline SAMD9 mutation in siblings with monosomy 7 and myelodysplastic syndrome. Leukemia 31 (8): 1827-1830, 2017. [PMC free article: PMC5540771] [PubMed: 28487541]
- Narumi S, Amano N, Ishii T, et al.: SAMD9 mutations cause a novel multisystem disorder, MIRAGE syndrome, and are associated with loss of chromosome 7. Nat Genet 48 (7): 792-7, 2016. [PubMed: 27182967]
- Chen DH, Below JE, Shimamura A, et al.: Ataxia-Pancytopenia Syndrome Is Caused by Missense Mutations in SAMD9L. Am J Hum Genet 98 (6): 1146-1158, 2016. [PMC free article: PMC4908176] [PubMed: 27259050]
- Wong JC, Bryant V, Lamprecht T, et al.: Germline SAMD9 and SAMD9L mutations are associated with extensive genetic evolution and diverse hematologic outcomes. JCI Insight 3 (14): , 2018. [PMC free article: PMC6124395] [PubMed: 30046003]
- Göhring G, Michalova K, Beverloo HB, et al.: Complex karyotype newly defined: the strongest prognostic factor in advanced childhood myelodysplastic syndrome. Blood 116 (19): 3766-9, 2010. [PubMed: 20802024]
- Haase D, Germing U, Schanz J, et al.: New insights into the prognostic impact of the karyotype in MDS and correlation with subtypes: evidence from a core dataset of 2124 patients. Blood 110 (13): 4385-95, 2007. [PubMed: 17726160]
- Arber DA, Vardiman JW, Brunning RD: Acute myeloid leukaemia with recurrent genetic abnormalities. In: Swerdlow SH, Campo E, Harris NL, et al., eds.: WHO Classification of Tumours of Haematopoietic and Lymphoid Tissues. 4th ed. International Agency for Research on Cancer, 2008, pp 110-23.
World Health Organization (WHO) Classification of Bone Marrow and Peripheral Blood Findings for MDS
Pediatric myelodysplastic neoplasms (MDS) can be grouped into several general categories, each with distinctive clinical and biological characteristics, as follows:[1]
- MDS arising from an inherited bone marrow failure syndrome, such as Fanconi anemia, severe congenital neutropenia, and Shwachman-Diamond syndrome, or a germline predisposition syndrome that confers higher risk of myeloid malignancy.
- MDS arising from severe aplastic anemia.
- Secondary MDS arising from cytotoxic exposures, such as high-dose alkylating chemotherapy.
Primary MDS includes cases of MDS beyond those listed above, acknowledging that some of the cases characterized as primary MDS are also associated with predisposition syndromes.
Distinguishing MDS from similar-appearing, reactive causes of dysplasia and/or cytopenias can be difficult. In general, the finding of ≥10% dysplasia in a cell lineage is a diagnostic criterion for MDS. However, the 2016 WHO guidelines caution that reactive etiologies, rather than clonal ones, may have ≥10% dysplasia and should be excluded, especially when dysplasia is subtle and/or restricted to a single lineage.[2]
The French-American-British (FAB) classification of MDS was not completely applicable to children.[3,4] Traditionally, MDS classification systems have been divided into several distinct categories based on the presence of the following:[4-7]
- Myelodysplasia.
- Types of cytopenia.
- Specific chromosomal abnormalities.
- Percentage of myeloblasts.
A modified classification schema for MDS and myeloproliferative disorders (MPDs) that included subsections on pediatric MDS and MPD was initially proposed in 2003 [8] and then published by the WHO in 2008.[9] A 2016 revision to the WHO classification removed focus on the specific lineage (anemia, thrombocytopenia, or neutropenia) and distinguished cases with dysplasia in single versus multiple lineages.
The 5th edition of the WHO Classification of Hematolymphoid Tumors includes a separate category for childhood myelodysplastic neoplasms. The WHO classification and defining features of MDS are summarized in Table 1.[10]
Table 1. World Health Organization Classification and Defining Features of Myelodysplastic Neoplasms (MDS)a
Blasts | Cytogenetics | Mutations | ||
---|---|---|---|---|
MDS with defining genetic abnormalities: | ||||
MDS with low blasts and isolated 5q deletion (MDS-5q) | <5% BM and <2% PB | 5q deletion alone, or with 1 other abnormality other than monosomy 7 or 7q deletion | ||
MDS with low blasts and SF3B1 mutationb (MDS-SF3B1) | Absence of 5q deletion, monosomy 7, or complex karyotype | SF3B1 | ||
MDS with biallelic TP53 inactivation (MDS-biTP53) | <20% BM and PB | Usually complex | Two or more TP53 mutations, or 1 mutation with evidence of TP53 copy number loss or cnLOH | |
MDS, morphologically defined: | ||||
MDS with low blasts (MDS-LB) | <5% BM and <2% PB | |||
MDS, hypoplasticc (MDS-h) | ||||
MDS with increased blasts (MDS-IB): | ||||
MDS-IB1 | 5%–9% BM or 2%–4% PB | |||
MDS-IB2 | 10%–19% BM or 5%–19% PB or Auer rods | |||
MDS with fibrosis (MDS-f) | 5%–19% BM; 2%–19% PB | |||
BM = bone marrow; cnLOH = copy neutral loss of heterozygosity; PB = peripheral blood. | ||||
aCredit: Khoury, J.D., Solary, E., Abla, O. et al. The 5th edition of the World Health Organization Classification of Haematolymphoid Tumours: Myeloid and Histiocytic/Dendritic Neoplasms. Leukemia 36, 1703–1719 (2022). https://doi | ||||
bDetection of ≥15% ring sideroblasts may substitute for SF3B1 mutation. Acceptable related terminology: MDS with low blasts and ring sideroblasts. | ||||
cBy definition, ≤25% bone marrow cellularity, age adjusted. |
- Childhood MDS with low blasts (cMDS-LB) includes cases with less than 5% blasts in the bone marrow and less than 2% blasts in the peripheral blood. This group replaces the prior category of refractory cytopenia of childhood. Patients with cMDS-LB may be further categorized as hypocellular or not otherwise specified.
- As in the 2016 WHO guidelines, reactive etiologies, rather than clonal ones, may have more than 10% dysplasia and should be excluded. Childhood MDS with increased blasts (cMDS-IB) includes the patients with 5% to 19% blasts in the bone marrow or 2% to 19% blasts in the peripheral blood. When children present with dysplasia and blast count <20% but genetic testing reveals recurrent cytogenetic abnormalities that are usually associated with acute myeloid leukemia (AML), a diagnosis of AML is made, and patients are treated accordingly.
The International Prognostic Scoring System (IPSS) is used to determine the risk of progression to AML and the outcome in adult patients with MDS. When this system was applied to children with MDS or juvenile myelomonocytic leukemia (JMML), only a blast count of less than 5% and a platelet count of more than 100 × 109/L were associated with a better survival in MDS, and a platelet count of more than 40 × 109/L predicted a better outcome in JMML.[11] These results suggest that MDS and JMML in children may be significantly different disorders than adult-type MDS.
The median survival for children with high-risk MDS remains substantially better than for adults, and the presence of monosomy 7 in children has not had the same adverse prognostic impact as in adults with MDS.[12] However, the presence of monosomy 7 in combination with other cytogenetic abnormalities is associated with a poor prognosis.[13,14] In one retrospective analysis, only the revised IPSS (R-IPSS) very poor–risk subgroup, defined as having complex cytogenetics (i.e., >3 abnormalities), was found to have a significant adverse prognostic impact on overall survival and relapse risk after transplant.[15] The relatively common abnormalities of -Y, 20q-, and 5q- in adults with MDS are rare in childhood MDS. Patients with recurrent cytogenetic abnormalities that are found in AML should be treated for AML and not MDS, regardless of blast percentage.
The R-IPSS prognostic groups and associated cytogenetic abnormalities include the following:[15]
- Very good prognostic group: -Y; del(11q).
- Good prognostic group: Normal; del(5q); del(20q); del(12p); double including del(5q).
- Intermediate prognostic group: del(7q); +8; i(17q); +19; any other single or double independent clones.
- Poor prognostic group: -7; inv(3)/t(3q)/del(3q); double including -7/del(7q); complex: 3 abnormalities.
- Very poor prognostic group: Complex: >3 abnormalities.
The IPSS can help to distinguish low-risk from high-risk MDS. However, its utility in children with MDS is more limited than in adults because many characteristics differ between children and adults.[11,16]
Genomic characterization of pediatric primary MDS has identified specific subsets defined by alterations in selected genes. For example, germline mutations in either GATA2,[17] SAMD9, or SAMD9L [18-20] are especially common in children with deletions of all or part of chromosome 7. Spontaneous remission of MDS in young children with SAMD9 or SAMD9L mutations led to the discovery that somatic genetic rescue can lead to phenotypic correction.[21] Genomic characterization has also shown that primary MDS in children differs from adult MDS at the molecular level.[19,22] For more information about MDS, see the Molecular Abnormalities section.
References
- Wlodarski MW, Sahoo SS, Niemeyer CM: Monosomy 7 in Pediatric Myelodysplastic Syndromes. Hematol Oncol Clin North Am 32 (4): 729-743, 2018. [PubMed: 30047423]
- Arber DA, Orazi A, Hasserjian R, et al.: The 2016 revision to the World Health Organization classification of myeloid neoplasms and acute leukemia. Blood 127 (20): 2391-405, 2016. [PubMed: 27069254]
- Bennett JM, Catovsky D, Daniel MT, et al.: Proposals for the classification of the myelodysplastic syndromes. Br J Haematol 51 (2): 189-99, 1982. [PubMed: 6952920]
- Mandel K, Dror Y, Poon A, et al.: A practical, comprehensive classification for pediatric myelodysplastic syndromes: the CCC system. J Pediatr Hematol Oncol 24 (7): 596-605, 2002. [PubMed: 12368708]
- Bennett JM: World Health Organization classification of the acute leukemias and myelodysplastic syndrome. Int J Hematol 72 (2): 131-3, 2000. [PubMed: 11039659]
- Head DR: Proposed changes in the definitions of acute myeloid leukemia and myelodysplastic syndrome: are they helpful? Curr Opin Oncol 14 (1): 19-23, 2002. [PubMed: 11790975]
- Nösslinger T, Reisner R, Koller E, et al.: Myelodysplastic syndromes, from French-American-British to World Health Organization: comparison of classifications on 431 unselected patients from a single institution. Blood 98 (10): 2935-41, 2001. [PubMed: 11698274]
- Hasle H, Niemeyer CM, Chessells JM, et al.: A pediatric approach to the WHO classification of myelodysplastic and myeloproliferative diseases. Leukemia 17 (2): 277-82, 2003. [PubMed: 12592323]
- Brunning RD, Porwit A, Orazi A, et al.: Myelodysplastic syndromes/neoplasms overview. In: Swerdlow SH, Campo E, Harris NL, et al., eds.: WHO Classification of Tumours of Haematopoietic and Lymphoid Tissues. 4th ed. International Agency for Research on Cancer, 2008, pp 88-93.
- Khoury JD, Solary E, Abla O, et al.: The 5th edition of the World Health Organization Classification of Haematolymphoid Tumours: Myeloid and Histiocytic/Dendritic Neoplasms. Leukemia 36 (7): 1703-1719, 2022. [PMC free article: PMC9252913] [PubMed: 35732831]
- Hasle H, Baumann I, Bergsträsser E, et al.: The International Prognostic Scoring System (IPSS) for childhood myelodysplastic syndrome (MDS) and juvenile myelomonocytic leukemia (JMML). Leukemia 18 (12): 2008-14, 2004. [PubMed: 15496981]
- Hasle H, Niemeyer CM: Advances in the prognostication and management of advanced MDS in children. Br J Haematol 154 (2): 185-95, 2011. [PubMed: 21554264]
- Göhring G, Michalova K, Beverloo HB, et al.: Complex karyotype newly defined: the strongest prognostic factor in advanced childhood myelodysplastic syndrome. Blood 116 (19): 3766-9, 2010. [PubMed: 20802024]
- Haase D, Germing U, Schanz J, et al.: New insights into the prognostic impact of the karyotype in MDS and correlation with subtypes: evidence from a core dataset of 2124 patients. Blood 110 (13): 4385-95, 2007. [PubMed: 17726160]
- Yamamoto S, Kato M, Watanabe K, et al.: Prognostic value of the revised International Prognostic Scoring System five-group cytogenetic abnormality classification for the outcome prediction of hematopoietic stem cell transplantation in pediatric myelodysplastic syndrome. Bone Marrow Transplant 56 (12): 3016-3023, 2021. [PubMed: 34508178]
- Cutler CS, Lee SJ, Greenberg P, et al.: A decision analysis of allogeneic bone marrow transplantation for the myelodysplastic syndromes: delayed transplantation for low-risk myelodysplasia is associated with improved outcome. Blood 104 (2): 579-85, 2004. [PubMed: 15039286]
- Wlodarski MW, Hirabayashi S, Pastor V, et al.: Prevalence, clinical characteristics, and prognosis of GATA2-related myelodysplastic syndromes in children and adolescents. Blood 127 (11): 1387-97; quiz 1518, 2016. [PubMed: 26702063]
- Narumi S, Amano N, Ishii T, et al.: SAMD9 mutations cause a novel multisystem disorder, MIRAGE syndrome, and are associated with loss of chromosome 7. Nat Genet 48 (7): 792-7, 2016. [PubMed: 27182967]
- Schwartz JR, Ma J, Lamprecht T, et al.: The genomic landscape of pediatric myelodysplastic syndromes. Nat Commun 8 (1): 1557, 2017. [PMC free article: PMC5691144] [PubMed: 29146900]
- Davidsson J, Puschmann A, Tedgård U, et al.: SAMD9 and SAMD9L in inherited predisposition to ataxia, pancytopenia, and myeloid malignancies. Leukemia 32 (5): 1106-1115, 2018. [PMC free article: PMC5940635] [PubMed: 29535429]
- Sahoo SS, Pastor VB, Goodings C, et al.: Clinical evolution, genetic landscape and trajectories of clonal hematopoiesis in SAMD9/SAMD9L syndromes. Nat Med 27 (10): 1806-1817, 2021. [PMC free article: PMC9330547] [PubMed: 34621053]
- Pastor V, Hirabayashi S, Karow A, et al.: Mutational landscape in children with myelodysplastic syndromes is distinct from adults: specific somatic drivers and novel germline variants. Leukemia 31 (3): 759-762, 2017. [PubMed: 27876779]
Treatment of Childhood MDS
Treatment options for children with myelodysplastic neoplasms (MDS) include the following:
- Hematopoietic stem cell transplant (HSCT) with or without chemotherapy.
HSCT
MDS and associated disorders usually involve a primitive hematopoietic stem cell. Thus, allogeneic HSCT is considered the optimal approach to treatment for pediatric patients with MDS. Although matched sibling donor transplant is preferred, similar survival has been noted with well-matched, unrelated cord blood and haploidentical approaches.[1-5]
Because survival after HSCT is improved in children with early forms of MDS (refractory anemia), transplant before progression to late MDS or acute myeloid leukemia (AML) should be considered. HSCT should especially be considered when transfusions or other treatments are required, as is usually the case in patients with severe symptomatic cytopenias.[4,6] The 8-year disease-free survival (DFS) rates for children with various stages of MDS has been reported to be 65% for those treated with HLA matched donor transplants and 40% for those treated with mismatched unrelated donor transplants.[6][Level of evidence C2] A 3-year DFS rate of 50% was reported with the use of unrelated cord blood donor transplants for children with MDS when the transplants were done after the year 2001.[7][Level of evidence C2]
When making treatment decisions, certain data should be considered, including the question of whether chemotherapy should be used in high-risk MDS. For example, survival as high as 80% has been reported for patients with early-stage MDS who proceeded to transplant within a few months of diagnosis. Additionally, early transplant and no pretransplant chemotherapy have been associated with improved survival in children with MDS.[8][Level of evidence C1] DFS rates have been estimated to be 50% to 70% for pediatric patients with advanced MDS using myeloablative transplant preparative regimens.[4,6,9-11] While nonmyeloablative preparative transplant regimens are being tested in patients with MDS and AML, such regimens are still investigational for children with these disorders. However, these regimens may be reasonable in the setting of a clinical trial or when a patient’s organ function is compromised in such a way that they would not tolerate a myeloablative regimen.[12-15]; [16][Level of evidence C1]
Evidence (HSCT):
- The Children's Cancer Group 2891 trial accrued patients between 1989 and 1995, including children with MDS.[9] There were 77 patients enrolled, including patients with refractory anemia (n = 2), refractory anemia with excess blasts (RAEB) (n = 33), refractory anemia with excess blasts in transformation (RAEB-T) (n = 26), or AML with antecedent MDS (n = 16). Patients were randomly assigned to receive either standard or intensively timed induction. Subsequently, patients were allocated to allogeneic HSCT if there was a suitable family donor or randomly assigned to either autologous HSCT or chemotherapy.
- Patients with refractory anemia or RAEB had a lower remission rate (45%). Those with RAEB-T (69%) or AML with a history of MDS (81%) had similar remission rates compared with de novo AML (77%).
- The 6-year survival rates were lower for those with refractory anemia or RAEB (28%) and RAEB-T (30%).
- Patients with AML and antecedent MDS had a similar outcome to those with de novo AML (survival rates, 50% vs. 45%, respectively).
- Allogeneic HSCT appeared to improve survival (P = .08).
- Based on the results of the EWOG-MDS 98 study, HSCT was verified as an important therapeutic approach necessary to achieve prolonged survival. For many patients, HSCT is the sole therapy received.[17] Children with RAEB (n = 53), RAEB-T (n = 29), and myelodysplasia-related AML (n = 15) were treated with an HSCT from various sources (related and unrelated) using the preparative regimen of busulfan, cyclophosphamide, and melphalan. Among this group, 73 were treated without the use of intensive therapy before the HSCT preparative regimen.
- Children with a diagnosis of RAEB and RAEB-T had equivalent event-free survival (EFS) rates of 63% (95% confidence interval [CI], 49%–77%) and 64% (95% CI, 46%–82%), respectively.
- For those with a morphological marrow blast percentage before HSCT of less than 5%, 5% to 20%, or 20% or higher, the EFS rates were 62% (95% CI, 41%–83%), 65% (95% CI, 50%–80%) and 45% (95% CI, 23%–67%), respectively.
- In the entire cohort (n = 97), patients who received low-dose therapy or no therapy before the preparative regimen (n = 73) had similar EFS rates compared with those who received prior intensive chemotherapy (58% [95% CI, 46%–70%] vs. 62% [95% CI, 42%–82%]).
- The outcomes of patients who received unrelated donor cells were like the outcomes of patients who received matched-family donor cells.
- A single-institution retrospective analysis reported on 37 consecutive children with various types of MDS who underwent HSCT using various donor types. Some patients were treated with pre-HSCT chemotherapy (n = 7).[8]
- In multivariate analysis, improved DFS was associated with avoiding pre-HSCT chemotherapy (relative risk [RR], 0.30; P = .03) and a shorter interval (<140 days) between diagnosis and HSCT (RR, 0.27; P = .02).
- In the 16 children who did not receive pre-HSCT chemotherapy and underwent transplant fewer than 140 days from diagnosis, the 3-year overall survival (OS) and DFS rates were both 80% (95% CI, 51%–93%).
When analyzing these results, it is important to consider that the subtype RAEB-T is likely to represent patients with overt AML, while refractory anemia and RAEB represents MDS. The World Health Organization classification has omitted the category of RAEB-T, concluding that it is essentially AML.
Because MDS in children is often associated with inherited predisposition syndromes, reports of transplant in small numbers of patients with these disorders have been documented. For example, in patients with Fanconi anemia and AML or advanced MDS, the 5-year OS rate has been reported to be 33% to 55%.[18,19][Level of evidence C1]
While some patients with inherited predisposition syndromes require significant modification of their transplant approaches because of excess toxicity (e.g., Fanconi anemia), other syndromes have no detectable excessive toxicity associated with the transplant process. Inherited GATA2 deficiency is a good example of the latter. One study compared HSCT outcomes of 65 children with GATA2 germline mutations and MDS with the outcomes of 404 children with MDS and wild-type germline GATA2. Rates of DFS, relapse, and nonrelapse mortality were similar in the two populations.[20]
Second transplants have also been used in pediatric patients with MDS/myeloproliferative disorders who relapse or experience graft failure. The 3-year OS rates were 33% for those who underwent a second transplant after relapse and 57% for those who underwent transplant after initial graft failure.[21][Level of evidence C1]
For patients with clinically significant cytopenias, supportive care that includes transfusions and prophylactic antibiotics are considered the standard of care. The use of hematopoietic growth factors can improve the hematopoietic status, but there are concerns that such treatment could accelerate conversion to AML.[22]
Other therapies
In general, the primary aim for children with newly diagnosed MDS is to rule out AML-associated somatic mutations, which would indicate the need to treat according to AML guidelines. Thereafter, the objective should be to provide supportive care while looking for an appropriate donor for HSCT. During this time, close monitoring for the emergence of AML is imperative.[23] Therapies used in adult MDS have not been shown to be beneficial in childhood MDS, likely owing to differences in underlying mutational etiologies.
Other therapies for MDS that have been studied and may be applicable include the following:
- Agents such as lenalidomide, an analog of thalidomide, have been tested based on findings that demonstrated increased activity in the bone marrow of patients with MDS. Lenalidomide has shown the most efficacy in patients with 5q- syndrome, especially those with thrombocytosis. The U.S. Food and Drug Administration approved lenalidomide for use in adults with this finding.[24]
References
- Uberti JP, Agovi MA, Tarima S, et al.: Comparative analysis of BU and CY versus CY and TBI in full intensity unrelated marrow donor transplantation for AML, CML and myelodysplasia. Bone Marrow Transplant 46 (1): 34-43, 2011. [PMC free article: PMC3169009] [PubMed: 20400989]
- Nemecek ER, Guthrie KA, Sorror ML, et al.: Conditioning with treosulfan and fludarabine followed by allogeneic hematopoietic cell transplantation for high-risk hematologic malignancies. Biol Blood Marrow Transplant 17 (3): 341-50, 2011. [PMC free article: PMC2974965] [PubMed: 20685259]
- Shaw PJ, Kan F, Woo Ahn K, et al.: Outcomes of pediatric bone marrow transplantation for leukemia and myelodysplasia using matched sibling, mismatched related, or matched unrelated donors. Blood 116 (19): 4007-15, 2010. [PMC free article: PMC2981549] [PubMed: 20671124]
- Parikh SH, Mendizabal A, Martin PL, et al.: Unrelated donor umbilical cord blood transplantation in pediatric myelodysplastic syndrome: a single-center experience. Biol Blood Marrow Transplant 15 (8): 948-55, 2009. [PubMed: 19589484]
- Locatelli F, Merli P, Pagliara D, et al.: Outcome of children with acute leukemia given HLA-haploidentical HSCT after αβ T-cell and B-cell depletion. Blood 130 (5): 677-685, 2017. [PubMed: 28588018]
- Woodard P, Carpenter PA, Davies SM, et al.: Unrelated donor bone marrow transplantation for myelodysplastic syndrome in children. Biol Blood Marrow Transplant 17 (5): 723-8, 2011. [PMC free article: PMC3033968] [PubMed: 20813197]
- Madureira AB, Eapen M, Locatelli F, et al.: Analysis of risk factors influencing outcome in children with myelodysplastic syndrome after unrelated cord blood transplantation. Leukemia 25 (3): 449-54, 2011. [PMC free article: PMC3365546] [PubMed: 21135856]
- Smith AR, Christiansen EC, Wagner JE, et al.: Early hematopoietic stem cell transplant is associated with favorable outcomes in children with MDS. Pediatr Blood Cancer 60 (4): 705-10, 2013. [PMC free article: PMC3668778] [PubMed: 23152304]
- Woods WG, Barnard DR, Alonzo TA, et al.: Prospective study of 90 children requiring treatment for juvenile myelomonocytic leukemia or myelodysplastic syndrome: a report from the Children's Cancer Group. J Clin Oncol 20 (2): 434-40, 2002. [PubMed: 11786571]
- Andolina JR, Kletzel M, Tse WT, et al.: Allogeneic hematopoetic stem cell transplantation in pediatric myelodysplastic syndromes: improved outcomes for de novo disease. Pediatr Transplant 15 (3): 334-43, 2011. [PubMed: 21492354]
- Al-Seraihy A, Ayas M, Al-Nounou R, et al.: Outcome of allogeneic stem cell transplantation with a conditioning regimen of busulfan, cyclophosphamide and low-dose etoposide for children with myelodysplastic syndrome. Hematol Oncol Stem Cell Ther 4 (3): 121-5, 2011. [PubMed: 21982885]
- Champlin R: Hematopoietic stem cell transplantation for treatment of myleodysplastic syndromes. Biol Blood Marrow Transplant 17 (1 Suppl): S6-8, 2011. [PubMed: 21195312]
- Nelson RP, Yu M, Schwartz JE, et al.: Long-term disease-free survival after nonmyeloablative cyclophosphamide/fludarabine conditioning and related/unrelated allotransplantation for acute myeloid leukemia/myelodysplasia. Bone Marrow Transplant 45 (8): 1300-8, 2010. [PubMed: 20062092]
- Warlick ED: Optimizing stem cell transplantation in myelodysplastic syndromes: unresolved questions. Curr Opin Oncol 22 (2): 150-4, 2010. [PubMed: 20010295]
- Pulsipher MA, Boucher KM, Wall D, et al.: Reduced-intensity allogeneic transplantation in pediatric patients ineligible for myeloablative therapy: results of the Pediatric Blood and Marrow Transplant Consortium Study ONC0313. Blood 114 (7): 1429-36, 2009. [PubMed: 19528536]
- Gao L, Gao L, Gong Y, et al.: Reduced-intensity conditioning therapy with fludarabine, idarubicin, busulfan and cytarabine for allogeneic hematopoietic stem cell transplantation in acute myeloid leukemia and myelodysplastic syndrome. Leuk Res 37 (11): 1482-7, 2013. [PubMed: 24054720]
- Strahm B, Nöllke P, Zecca M, et al.: Hematopoietic stem cell transplantation for advanced myelodysplastic syndrome in children: results of the EWOG-MDS 98 study. Leukemia 25 (3): 455-62, 2011. [PubMed: 21212791]
- Mitchell R, Wagner JE, Hirsch B, et al.: Haematopoietic cell transplantation for acute leukaemia and advanced myelodysplastic syndrome in Fanconi anaemia. Br J Haematol 164 (3): 384-95, 2014. [PMC free article: PMC4060801] [PubMed: 24172081]
- Ayas M, Saber W, Davies SM, et al.: Allogeneic hematopoietic cell transplantation for fanconi anemia in patients with pretransplantation cytogenetic abnormalities, myelodysplastic syndrome, or acute leukemia. J Clin Oncol 31 (13): 1669-76, 2013. [PMC free article: PMC3635221] [PubMed: 23547077]
- Bortnick R, Wlodarski M, de Haas V, et al.: Hematopoietic stem cell transplantation in children and adolescents with GATA2-related myelodysplastic syndrome. Bone Marrow Transplant 56 (11): 2732-2741, 2021. [PMC free article: PMC8563415] [PubMed: 34244664]
- Kato M, Yoshida N, Inagaki J, et al.: Salvage allogeneic stem cell transplantation in patients with pediatric myelodysplastic syndrome and myeloproliferative neoplasms. Pediatr Blood Cancer 61 (10): 1860-6, 2014. [PubMed: 24976435]
- Zwierzina H, Suciu S, Loeffler-Ragg J, et al.: Low-dose cytosine arabinoside (LD-AraC) vs LD-AraC plus granulocyte/macrophage colony stimulating factor vs LD-AraC plus Interleukin-3 for myelodysplastic syndrome patients with a high risk of developing acute leukemia: final results of a randomized phase III study (06903) of the EORTC Leukemia Cooperative Group. Leukemia 19 (11): 1929-33, 2005. [PubMed: 16151466]
- Locatelli F, Strahm B: How I treat myelodysplastic syndromes of childhood. Blood 131 (13): 1406-1414, 2018. [PubMed: 29438960]
- Yazji S, Giles FJ, Tsimberidou AM, et al.: Antithymocyte globulin (ATG)-based therapy in patients with myelodysplastic syndromes. Leukemia 17 (11): 2101-6, 2003. [PubMed: 12931212]
Treatment Options Under Clinical Evaluation
Information about National Cancer Institute (NCI)–supported clinical trials can be found on the NCI website. For information about clinical trials sponsored by other organizations, see the ClinicalTrials.gov website.
Latest Updates to This Summary (03/06/2024)
The PDQ cancer information summaries are reviewed regularly and updated as new information becomes available. This section describes the latest changes made to this summary as of the date above.
This is a new summary.
This summary is written and maintained by the PDQ Pediatric Treatment Editorial Board, which is editorially independent of NCI. The summary reflects an independent review of the literature and does not represent a policy statement of NCI or NIH. More information about summary policies and the role of the PDQ Editorial Boards in maintaining the PDQ summaries can be found on the About This PDQ Summary and PDQ® Cancer Information for Health Professionals pages.
About This PDQ Summary
Purpose of This Summary
This PDQ cancer information summary for health professionals provides comprehensive, peer-reviewed, evidence-based information about the treatment of childhood myelodysplastic neoplasms. It is intended as a resource to inform and assist clinicians in the care of their patients. It does not provide formal guidelines or recommendations for making health care decisions.
Reviewers and Updates
This summary is reviewed regularly and updated as necessary by the PDQ Pediatric Treatment Editorial Board, which is editorially independent of the National Cancer Institute (NCI). The summary reflects an independent review of the literature and does not represent a policy statement of NCI or the National Institutes of Health (NIH).
Board members review recently published articles each month to determine whether an article should:
- be discussed at a meeting,
- be cited with text, or
- replace or update an existing article that is already cited.
Changes to the summaries are made through a consensus process in which Board members evaluate the strength of the evidence in the published articles and determine how the article should be included in the summary.
The lead reviewers for Childhood Myelodysplastic Neoplasms Treatment are:
- Alan Scott Gamis, MD, MPH (Children's Mercy Hospital)
- Karen J. Marcus, MD, FACR (Dana-Farber Cancer Institute/Boston Children's Hospital)
- Jessica Pollard, MD (Dana-Farber/Boston Children's Cancer and Blood Disorders Center)
- Michael A. Pulsipher, MD (Children's Hospital Los Angeles)
- Rachel E. Rau, MD (University of Washington School of Medicine, Seatle Children’s)
- Lewis B. Silverman, MD (Dana-Farber Cancer Institute/Boston Children's Hospital)
- Malcolm A. Smith, MD, PhD (National Cancer Institute)
- Sarah K. Tasian, MD (Children's Hospital of Philadelphia)
Any comments or questions about the summary content should be submitted to Cancer.gov through the NCI website's Email Us. Do not contact the individual Board Members with questions or comments about the summaries. Board members will not respond to individual inquiries.
Levels of Evidence
Some of the reference citations in this summary are accompanied by a level-of-evidence designation. These designations are intended to help readers assess the strength of the evidence supporting the use of specific interventions or approaches. The PDQ Pediatric Treatment Editorial Board uses a formal evidence ranking system in developing its level-of-evidence designations.
Permission to Use This Summary
PDQ is a registered trademark. Although the content of PDQ documents can be used freely as text, it cannot be identified as an NCI PDQ cancer information summary unless it is presented in its entirety and is regularly updated. However, an author would be permitted to write a sentence such as “NCI’s PDQ cancer information summary about breast cancer prevention states the risks succinctly: [include excerpt from the summary].”
The preferred citation for this PDQ summary is:
PDQ® Pediatric Treatment Editorial Board. PDQ Childhood Myelodysplastic Neoplasms Treatment. Bethesda, MD: National Cancer Institute. Updated <MM/DD/YYYY>. Available at: https://www.cancer.gov/types/leukemia/hp/child-aml-treatment-pdq/myeloid-dysplastic-neoplasms-treatment-pdq. Accessed <MM/DD/YYYY>.
Images in this summary are used with permission of the author(s), artist, and/or publisher for use within the PDQ summaries only. Permission to use images outside the context of PDQ information must be obtained from the owner(s) and cannot be granted by the National Cancer Institute. Information about using the illustrations in this summary, along with many other cancer-related images, is available in Visuals Online, a collection of over 2,000 scientific images.
Disclaimer
Based on the strength of the available evidence, treatment options may be described as either “standard” or “under clinical evaluation.” These classifications should not be used as a basis for insurance reimbursement determinations. More information on insurance coverage is available on Cancer.gov on the Managing Cancer Care page.
Contact Us
More information about contacting us or receiving help with the Cancer.gov website can be found on our Contact Us for Help page. Questions can also be submitted to Cancer.gov through the website’s Email Us.
- General Information About Childhood Myelodysplastic Neoplasms (MDS)
- Clinical Presentation
- Risk Factors
- Molecular Abnormalities
- World Health Organization (WHO) Classification of Bone Marrow and Peripheral Blood Findings for MDS
- Treatment of Childhood MDS
- Treatment Options Under Clinical Evaluation
- Latest Updates to This Summary (03/06/2024)
- About This PDQ Summary
- Review Myelodysplastic Syndromes Treatment (PDQ®): Health Professional Version.[PDQ Cancer Information Summari...]Review Myelodysplastic Syndromes Treatment (PDQ®): Health Professional Version.PDQ Adult Treatment Editorial Board. PDQ Cancer Information Summaries. 2002
- Review Myelodysplastic/Myeloproliferative Neoplasms Treatment (PDQ®): Health Professional Version.[PDQ Cancer Information Summari...]Review Myelodysplastic/Myeloproliferative Neoplasms Treatment (PDQ®): Health Professional Version.PDQ Adult Treatment Editorial Board. PDQ Cancer Information Summaries. 2002
- Review Childhood Rhabdomyosarcoma Treatment (PDQ®): Health Professional Version.[PDQ Cancer Information Summari...]Review Childhood Rhabdomyosarcoma Treatment (PDQ®): Health Professional Version.PDQ Pediatric Treatment Editorial Board. PDQ Cancer Information Summaries. 2002
- Review Childhood Craniopharyngioma Treatment (PDQ®): Health Professional Version.[PDQ Cancer Information Summari...]Review Childhood Craniopharyngioma Treatment (PDQ®): Health Professional Version.PDQ Pediatric Treatment Editorial Board. PDQ Cancer Information Summaries. 2002
- Review Childhood Ependymoma Treatment (PDQ®): Health Professional Version.[PDQ Cancer Information Summari...]Review Childhood Ependymoma Treatment (PDQ®): Health Professional Version.PDQ Pediatric Treatment Editorial Board. PDQ Cancer Information Summaries. 2002
- Childhood Myelodysplastic Neoplasms Treatment (PDQ®) - PDQ Cancer Information Su...Childhood Myelodysplastic Neoplasms Treatment (PDQ®) - PDQ Cancer Information Summaries
- Uncharacterized protein CELE_B0228.8 [Caenorhabditis elegans]Uncharacterized protein CELE_B0228.8 [Caenorhabditis elegans]gi|115533979|ref|NP_495630.2|Protein
- BB323930 RIKEN full-length enriched, 4 days neonate male adipose Mus musculus cD...BB323930 RIKEN full-length enriched, 4 days neonate male adipose Mus musculus cDNA clone B430101L16 3', mRNA sequencegi|16403486|gnl|dbEST|9999421|dbj|B 30.2|Nucleotide
- Mus musculus BAC clone RP23-332O23 from chromosome 14, complete sequenceMus musculus BAC clone RP23-332O23 from chromosome 14, complete sequencegi|77020526|gb|AC166828.3||gnl|wugs 3-332O23Nucleotide
Your browsing activity is empty.
Activity recording is turned off.
See more...