NCBI Bookshelf. A service of the National Library of Medicine, National Institutes of Health.
Lux LJ, Posey RE, Daniels LS, et al. Pharmacokinetic/Pharmacodynamic Measures for Guiding Antibiotic Treatment for Hospital-Acquired Pneumonia [Internet]. Rockville (MD): Agency for Healthcare Research and Quality (US); 2014 Nov. (Comparative Effectiveness Reviews, No. 136.)
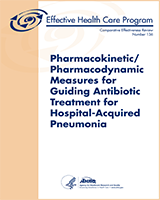
Pharmacokinetic/Pharmacodynamic Measures for Guiding Antibiotic Treatment for Hospital-Acquired Pneumonia [Internet].
Show detailsBackground
Hospital-Acquired Pneumonia: Epidemiology
Hospital-acquired (or nosocomial) pneumonia (HAP) is the second most common hospital-acquired infection. It occurs especially in the elderly, immunocompromised patients, surgical patients, and individuals receiving enteral feeding through a nasogastric tube. The incidence rates for HAP, which can occur in all areas of hospitals, range from 5 to more than 20 per 1,000 admissions.1,2
HAP is the leading cause of hospital-acquired infection in the intensive care unit (ICU).1 Almost one-third of HAP episodes are acquired in ICUs;3 as many as 90 percent of ICU cases may be ventilator associated.3,4 In the ICU setting, HAP accounts for up to 25 percent of all infections and for more than 50 percent of the antibiotics prescribed.1
Guidelines issued in 2005 by the American Thoracic Society (ATS) and the Infectious Diseases Society of America (IDSA) described HAP and two related pneumonias, ventilator-associated pneumonia (VAP) and healthcare-associated pneumonia (HCAP).1 Briefly:
- HAP is a pneumonia that occurs 48 hours or more after admission and was not incubating at the time of admission.
- VAP is a pneumonia that presents more than 48 to 72 hours after endotracheal intubation. It is a severe type of HAP; because of the difficulty in treating it, its prognosis can be poor.
- HCAP is a pneumonia that develops in any patient who meets one or more of several criteria: had been hospitalized in an acute care hospital for 2 or more days within 90 days of the infection; had resided in a nursing home or long-term care facility; had received recent intravenous (IV) antibiotic therapy, chemotherapy, or wound care within the 30 days preceding the current infection; or had attended a hospital or hemodialysis clinic.
Unless we specify otherwise, the term “HAP” includes VAP and HCAP throughout the report. Most biological and clinical principles for HAP and VAP overlap those for HCAP.
HAP is most often caused by bacterial pathogens, and it may be polymicrobial. Staphyloccus aureus—especially methicillin-resistant S. aureus (MRSA)—and aerobic Gram-negative bacilli, including Pseudomonas aeruginosa, Escherichia coli, Klebsiella pneumoniae, and Acinetobacter species, are the common causes of HAP. HAP caused by S. aureus is found with greater frequency in patients with diabetes mellitus, patients with head trauma, and patients hospitalized in ICUs. HAP caused by viral or fungal pathogens is rare in immunocompetent patients.1,5
Because HAP, VAP, and HCAP share similar microbial sources, they are treated similarly. The general approach is to treat broadly for resistant Gram-positive and Gram-negative organisms, then deescalate therapy when the pathologic agent is defined. Clinicians may manage HAP patients in a hospital ward or in an ICU when the illness is more severe. Some patients may require intubation after developing severe HAP; in these cases, clinicians should treat them in ways similar to those used for treating patients with VAP.
HAP is associated with increased morbidity and mortality, longer lengths of inpatient stays, and higher costs of care compared with hospital episodes not complicated by HAP despite advances in antimicrobial therapy, supportive care, and prevention. For example, episodes of HAP that are not associated with ventilator use raise both hospital lengths of stay and costs of care; in one report from Asian countries, they were associated with death rates of between 27 percent and 50 percent.2
Patients who have received mechanical ventilation are at the greatest risk for HAP; intubation increases a patient's HAP risk by 6 to 21 times. Mortality from VAP among patients who have acquired VAP in ICUs can be higher for patients who receive inadequate empirical therapy.6 Additional costs per episode of VAP may be as high as $40,000.7
Hospital-Acquired Infection: Treatment
Appropriate antibiotic therapy significantly improves survival for patients with HAP.8-11 Relevant antibiotics for treating HAP patients include broad-spectrum beta-lactams, vancomycin, and aminoglycosides, among others. Table A lists antibiotic classes and individual agents that clinicians might use to treat HAP; bold items are those used most often.
Table A
Intravenous antibiotics for which PK/PD measures could be used.
Optimal treatment involves choosing the right drug or combination of drugs, the proper dose and route of administration, and the appropriate duration, followed by deescalation to pathogen-directed therapy.1 Subtherapeutic dosing of antibiotics has been associated with poorer clinical outcomes and emergence of antibiotic resistance.12-15
Optimal dosing of antibiotics based on principles of pharmacokinetics and pharmacodynamics (PK/PD) has the potential to improve outcomes and prevent the development of resistance in patients with HAP. PK is the study of the time course of drug absorption, distribution, metabolism, and excretion. The primary goals of clinical PK include enhancing efficacy and decreasing toxicity of an individual patient's drug therapy. PD refers to the relationship between the concentration of the drug at the site of action and the resulting effect. Antibiotic PD relates PK parameters to the ability of an antibiotic to kill or inhibit growth of bacterial pathogens.16 Antibiotics can be classified based on PD characteristics that affect bacterial killing in relation to the minimum inhibitory concentration (MIC) of the organism.
To improve the effectiveness of the available antibiotics specifically for HAP, the 2005 ATS/IDSA guidelines recommend considering PK/PD properties when selecting an antibiotic regimen, dosage, and route of administration. The goal of these guidelines is to provide recommendations for the selection of adequate therapy and thereby achieve optimal patient outcomes. This antibiotic dosing logic is based on serum antibiotic concentrations in vitro and in vivo observations. For those reasons, it may not account fully for the heterogeneity of patient populations with HAP, the complex pathologic environment in the infected lung, and the drug concentration achieved at the site of the pneumonia. Current antibiotic dosing strategies also do not directly consider the variety of antibiotic-resistance mechanisms in bacteria that contribute to the persistence of HAP.
Furthermore, measuring PK/PD only in the serum may lead to suboptimal antibiotic concentrations at the site of infection—in this case, the lung. In such cases, the antibiotic may not eradicate resistant organisms; this problem may in turn lead to treatment failure and contribute to emerging antibiotic resistance. Generally speaking, given the unique attributes of the lung that contribute to the challenge of adequately treating patients with HAP, these issues are of special concern for clinicians and others in providing fully successful services for such patients.
Concerns in the United States and abroad about the increasing rates of superinfection (i.e., infection with a new organism) and new resistance patterns in pathogens call for strategies to optimize existing antibiotic treatment options for HAP.17,18 Antibiotic resistance is a growing and significant threat to public health. The incidence rates of drug resistance among many common HAP pathogens have increased dramatically over the past three decades. During the same period, the number of new antibiotics developed has decreased, especially for drugs that target Gram-negative organisms. In addition, treatment of MRSA pneumonia has become more difficult because of the rising incidence of infections caused by isolates with increased MICs to vancomycin (“MIC creep”). To reach proposed pharmacodyamic targets, higher doses of vancomycin are needed, which increases risks of toxicities.19 With fewer antibiotic options, ensuring the appropriate and judicious use of these drugs becomes increasingly important.20,21
Although optimization of antibiotic dosing is important to improve individual patient outcomes with HAP, optimal antimicrobial exposure may also serve to prevent the emergence of resistant populations of organisms. Subtherapeutic concentrations of antibiotics may contribute to the emergence or acceleration of resistance. Consequently, any procedures that can help to guide dosing of antibiotics have important implications, not only for the individual patient being treated, but also for public health concerns.
Scope and Key Questions
Scope of This Review
This review aims to document the impact of contemporary approaches to PK/PD-guided dosing of IV antibiotic therapy on clinical outcomes for patients with HAP. In general, antibiotics are grouped into one of three categories based on their mode of bacterial killing: (1) concentration dependent, (2) time dependent, or (3) a combination of concentration and time dependent. These three modes are expressed as ratios to the MIC of the organisms (Figure A).
- Concentration-dependent antibiotic: peak concentration to MIC (expressed as Cmax/MIC)
- Time-dependent antibiotic: time that the serum concentration is greater than the MIC (expressed as T>MIC)
- Area under the curve (AUC) for the concentration-time curve in relationship to MIC (expressed as AUC/MIC)
Given the PK/PD properties of antibiotics, clinicians can optimize the PD effects of antibiotics by making decisions about dosing strategies. For example, to optimize the PD effect of a concentration-dependent antibiotic, clinicians may choose to increase the dose, resulting in a higher Cmax/MIC ratio.
Populations of interest for this review include adults who have presumed or confirmed HAP, VAP, or HCAP and who are being treated with IV antibiotic treatment. We looked at benefits defined as both intermediate outcomes (clinical response and use of ventilators) and health outcomes (morbidity and mortality); we also examined evidence about adverse events (harms). We examined evidence relating to HAP that begins in the hospital setting (e.g., emergency department, floor, or ICU) and relating to treatment that continues in other settings; we also included studies of patients who acquired HAP in a nursing home setting.
This review is relevant to several dilemmas that clinicians face about how best to select doses and to monitor the use of IV antibiotics for these severely ill patients while taking account of the PD properties of different IV antibiotics, various patient-specific factors, and resistance patterns of the pathogens. Of concern are both presumed benefits and harms of using PK/PD measures for these purposes. The review also attempts to address one specific question concerning the beta-lactam class of antibiotics. Finally, we examine what may be known about how outcomes (benefits or harms) relate to patient populations characterized by sociodemographic or clinical characteristics.
We excluded studies of fungal pneumonia in this review, because fungal infections would involve a different set of populations, interventions, comparators, outcomes, timing of measurement or followup, and settings (PICOTS) from those found in the literature for bacterial infections. Because the report scope was limited to HAP, VAP, or HCAP, we also excluded studies of community-acquired pneumonia and of other pneumonias for which treatment began in a setting other than the hospital (or nursing home). In addition, because of the report's focus on pneumonia, we did not include studies of shock, sepsis, or other infections that did not provide data for HAP patients. Finally, we excluded studies in which serum concentration had been measured without comparing different serum concentration targets; this type of intervention would be considered standard of care and is not a study design that is looking at optimization of PK/PD measures to inform treatment decisions.
Key Questions
We addressed three Key Questions (KQs). The analytic framework used to guide this review can be found in Figure 2 of the full report.
- Key Question 1.
For people with hospital-acquired pneumonia, how does using PK/PD measures to inform decisions about dosing or monitoring antibiotic treatment affect:
- Clinical response or mechanical ventilation?
- Morbidity or mortality?
- Rates of antibiotic-related adverse events?
- Key Question 2.
For people with hospital-acquired pneumonia, how does using prolonged or continuous infusions compared with bolus infusions for beta-lactams affect:
- Clinical response or mechanical ventilation?
- Morbidity or mortality?
- Rates of antibiotic-related adverse events?
- Key Question 3.
For people with hospital-acquired pneumonia, does the evidence for clinical response, mechanical ventilation, morbidity, mortality, or antibiotic-related adverse events differ for subgroups defined by age, sex, race, ethnicity, renal dysfunction or need for dialysis, severity of illness, microorganism, or susceptibility patterns when examining the use of PK/PD measures to inform decisions about dosing and monitoring antibiotic treatment or when comparing prolonged or continuous infusions versus bolus infusions for beta-lactams?
Methods
Our protocol for this review was registered with PROSPERO (CRD42013005309).
Literature Search Strategy
Search Strategy
We searched MEDLINE® (via PubMed), the Cochrane Library, and the International Pharmaceutical Abstracts for English-language and human-only studies from January 1, 2004, through May 15, 2013; we later updated the searches through June 7, 2014. We used either medical subject headings (MeSH) or major headings as search terms when available or key words when appropriate, focusing on terms to describe the relevant population and interventions of interest. We reviewed our search strategy with Technical Expert Panel members and incorporated their input into our search strategies. An experienced information scientist, our Evidence-based Practice Center (EPC) librarian, ran the searches; another EPC librarian peer-reviewed the searches.
We manually searched reference lists of pertinent reviews and included trials, and searched background articles on this topic to identify any relevant citations that our searches might have missed. We searched for relevant unpublished studies using ClinicalTrials.gov and the World Health Organization's International Clinical Trials Registry Platform.
Inclusion and Exclusion Criteria
We developed eligibility (inclusion and exclusion) criteria with respect to PICOTS, study designs, and durations for each KQ. Our review focused on adults (age 18 years and older) who have presumed or confirmed HAP, VAP, or HCAP and are being treated with IV antibiotics. For KQ 1, we required studies to assess an intervention focused on using PK/PD measures to inform decisions: serum concentration, volume of distribution, protein binding, time above MIC, and ratio of AUC to MIC. For KQ 2, we required studies to compare prolonged or continuous infusions with bolus infusions for beta-lactams. (As noted above, the clinical concern for this review is the lung and specifically pneumonia, so studies about other types of infections or infections in other organ systems are excluded.)
For KQs 1 and 3, eligible comparators included: no use of PK/PD measures, different targets of PK/PD measures, or usual care (e.g., physician discretion or judgment, local epidemiology of bacteria and resistance). For KQs 2 and 3, the eligible comparator was bolus dosing. We required that at least one of our specified outcomes be measured and reported: intermediate outcomes (clinical response, occurrence or duration of mechanical ventilation); health outcomes (mortality, reinfection, relapse, superinfection); and antibiotic adverse events (organ toxicity, hematologic effects, Clostridium difficile infection, antibiotic resistance). No limits were placed on timing of the measurement or followup. HAP had to have begun in a health care setting (e.g., skilled nursing facility) and be treated in the hospital (e.g., emergency department, floor, or ICU).
For both intermediate and health outcomes, randomized controlled trials (RCTs), nonrandomized controlled trials, and prospective cohort studies were eligible. For adverse effects data, case-control and retrospective cohort studies were also eligible.
Study Selection
Two trained members of the research team independently reviewed all titles and abstracts for eligibility against our eligibility criteria. Studies marked for possible inclusion by either reviewer underwent a full-text review. Studies whose titles and abstracts lacked adequate information to determine inclusion or exclusion underwent a full-text review.
Two trained members of the research team independently reviewed each full-text article for inclusion or exclusion based on the eligibility criteria described above. If both reviewers agreed that a study did not meet the eligibility criteria, we excluded it. If the reviewers disagreed, they resolved conflicts by discussion and consensus or by consulting a third senior member of the review team.
Data Extraction
For studies meeting inclusion criteria, we extracted important information into evidence tables. For this purpose, we designed and used structured data-extraction forms that included characteristics of study populations, settings, interventions, comparators, study designs, methods, and results. Trained reviewers recorded relevant data from the studies; a second member of the team reviewed all data abstractions for completeness and accuracy.
Risk-of-Bias Assessment of Individual Studies
To assess the risk of bias (i.e., the internal validity) of studies, we applied predefined criteria based on the Agency for Healthcare Research and Quality (AHRQ) “Methods Guide for Effectiveness and Comparative Effectiveness Reviews” (Methods Guide).22 This approach uses questions to assess selection bias, confounding, performance bias, detection bias, and attrition bias—that is, it addresses issues of adequacy of randomization, allocation concealment, similarity of groups at baseline, masking, attrition, whether intention-to-treat analysis was used, method of handling dropouts and missing data, validity and reliability of outcome measures, and treatment fidelity.
Two independent reviewers assessed risk of bias for each study, assigning a rating of low, medium, or high risk of bias. Disagreements between the two reviewers were resolved by discussion and consensus or by consulting a third member of the team.
Data Synthesis
We did not find multiple studies for any comparison of interest that reported similar outcomes; for that reason, we could not consider quantitative synthesis (i.e., meta-analysis) of data from included studies. All analyses in this review are, therefore, qualitative. We synthesized data from the included studies in tabular and narrative format. Synthesized evidence was organized by KQ.
Strength of Evidence of the Body of Evidence
We graded the strength of evidence based on the guidance established for the EPC program.23 Developed to grade the overall strength of a body of evidence, this approach incorporates four required domains: risk of bias (including study design and aggregate quality), consistency, directness, and precision of the evidence.
Two reviewers independently assessed each domain for each key outcome and resolved differences by consensus. The overall grade was based on a qualitative decision taking into account the ratings for the four required domains. Reviewers can assign one of four strength-of-evidence grades: high, medium, low, or insufficient. For the last, evidence either is unavailable or does not permit estimation of an effect.
We graded the strength of evidence for the following outcomes: clinical response, mechanical ventilation, treatment failure, mortality, superinfection, and antibiotic-related adverse effects.
Applicability
We assessed the applicability of both individual studies and the body of evidence following guidance from the AHRQ Methods Guide.24 For individual studies, we examined factors that may limit applicability based on the PICOTS framework. Some factors identified a priori that could limit the applicability of evidence for this review included the following: severity of illness, whether studies enrolled patients with chronic lung diseases, and settings.
Results
Results of Literature Searches
From an unduplicated pool of 2,134 possible articles, we excluded 1,894 at the title and abstract review stage and another 240 at the full-text review stage (Figure B). We included 10 studies reported in 11 published articles. Of these, one study pertained to KQ 1; nine pertained to KQ 2. We identified no studies addressing KQ 3, on subgroups.
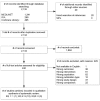
Figure B
Disposition of articles about using PK/PD measures in hospital-acquired pneumonia. IPA=International Pharmaceutical Abstracts; KQ = Key Question; PK/PD = pharmacokinetic/pharmacodynamic; SIP = Scientific Information Packet
Seven studies were RCTs.25-32 Two were prospective cohort studies,33,34 and one was a retrospective cohort study.35 All seven RCTs addressed KQ 2. One prospective cohort study pertained to KQ 133 and the other to KQ234; the retrospective cohort study addressed KQ 2. We rated five the trials and one cohort study as medium risk of bias and two trials and two cohort studies as high risk of bias.
Key Question 1. PK/PD Measures for Dosing or Monitoring
Evidence was insufficient for clinical response, mechanical ventilation, treatment failure, and mortality (Table B). The evidence base was a single prospective cohort study that we rated as high risk of bias for multiple reasons, including high risk of measurement bias and confounding. Further, methods were not clearly described. Investigators reported significantly improved outcomes with PK/PD in terms of cure and mortality, but both measures were problematic.33 Whether the data reported were based on clinical or microbiologic success data (or both) was unclear, and mortality was combined with “leaving against medical advice.”
Table B
Strength of evidence for using PK/PD measures to influence dosing or monitoring.
Key Question 2. Prolonged or Continuous Infusions
For KQ 2 (Table C), we graded evidence as insufficient for all outcomes. We had no more than one study for any included outcome, and this small number of studies had small numbers of patients. These problems generally resulted in unknown consistency and imprecision. Evidence is insufficient to draw conclusions about the effect of continuous infusions compared with the effect of intermittent infusions on outcomes related to clinical response, mechanical ventilation, morbidity, or mortality. The evidence for these outcomes consisted of one small trial.26,28 Evidence is also insufficient to draw conclusions about the effect of continuous infusions versus intermittent infusions on the rates of antibiotic-related adverse events.25-29,35
Table C
Strength of evidence for comparisons of continuous and intermittent infusion.
Key Question 3. Subgroup Analyses
We found no studies meeting inclusion criteria. Consequently, evidence was insufficient.
Discussion
Key Findings and Strength of Evidence
Comparative evidence is scarce on use of PK/PD measures in dosing or monitoring. Similarly, little evidence is available on use of PK/PD strategies in adult patients with HAP who are being treated with IV antibiotics.
The strength of evidence is insufficient to conclude whether using measures to inform decisions about dosing or monitoring IV antibiotic treatment (KQ 1) improves either intermediate or health outcomes. We found only a single prospective cohort study (which we rated as high risk of bias) that used PK/PD measures to study the impact of different antibiotic dosing on clinical responses, such as time on mechanical ventilation, treatment failure, and mortality.
Evidence is also insufficient to draw conclusions about the effect of continuous infusions of beta-lactam antibiotics compared with the effect of intermittent infusions on outcomes related to clinical response, mechanical ventilation, morbidity, mortality, or rates of antibiotic-related adverse events (KQ 2). Pertinent studies found no significant differences in clinical response, duration of mechanical ventilation, superinfection, rates of antibiotic-related adverse events, or infusion-related adverse effects.
We determined that very little research has focused on the use of PK/PD measures in dosing or monitoring adult patients with HAP being treated with IV antibiotics. This dearth of studies suggests that the research conducted to date has been conducted in in vitro and animal studies. In what little is published relating to different PK/PD strategies, investigators have studied mixed populations, including patients with a variety of conditions (e.g., sepsis, bacteremia, community-acquired pneumonia, HAP) without reporting outcomes for patients with HAP (including VAP and HCAP), separately. Our review focused solely on HAP and explicitly omitted community-acquired pneumonia.
Many national and international organizations have recognized the growing global problem of antibiotic resistance and have made efforts to raise public awareness and coordinate actions to address problems related to resistance. For example, the U.S. National Institutes of Health has issued new funding opportunities to encourage new antibiotic developments, and the Centers for Disease Control and Prevention has launched the Get Smart Campaign to encourage the judicious use of antibiotics. Strategies often employed include infection control and prevention techniques such as hand-washing, development of rapid point-of-care diagnostic tests to diagnose infection more rapidly and accurately, public policies to support development and approval of new drugs to treat resistant infections, and implementation of coordinated efforts to optimize antibiotic use through practices referred to as antibiotic stewardship.
Antibiotic stewardship programs have several goals. Among them are improving appropriate use of antibiotics by promoting antibiotic use only when indicated and selecting optimal antimicrobial drug regimens to improve clinical outcomes. Minimizing toxicity and other adverse events, including limiting the emergence of antibiotic-resistant strains of bacteria, is a related goal. Such programs often focus on streamlining antimicrobial therapy, deescalating or targeting antibiotics based on microbiological data, minimizing excessive durations of antibiotic courses, and optimizing antibiotic doses.
The IDSA, the Society for Healthcare Epidemiology of America, and the Pediatric Infectious Diseases Society have all made recommendations to the Centers for Medicare & Medicaid Services to require antibiotic stewardship programs in all acute care hospitals in the United States.36 Pharmacodynamic dose optimization has been suggested as a strategy for antibiotic stewardship programs to employ to improve antibiotic use.37 In fact, the IDSA guidelines for developing an institutional program to enhance antimicrobial stewardship refer to PK and PD considerations as important parts of antimicrobial stewardship.38
Given the dearth of findings in this review, the evidence base provides little guidance for either clinical or policy decisionmaking. We comment here on two key issues that warrant attention by health professionals, policymakers, and society at large; we offer specific recommendations about filling these research gaps below.
First, as antimicrobial resistance becomes a global problem, appropriate use of antibiotics is of paramount importance. Appropriate use encompasses optimal dosing strategies that are cost effective, can improve patient outcomes, and combat further development of resistance. These matters are relevant to clinicians, hospital administrators, insurers, patients, and public-sector agencies. With respect specifically to PK/PD approaches, of particular interest are exposure-response relationships of antibiotics, antibiotic use in “real-world” clinical settings (all types of hospitals and ICUs), and a broad range of patient-centered outcomes (clinical response, morbidity, mortality, and adverse events) as well as costs of care.
Second, almost a decade ago, ATS redefined dosing guidelines based on PK/PD principles and clinical trial efficacy data.1 Nevertheless, the effectiveness of the dosing strategies described in these guidelines remains unclear. Clinicians and policymakers alike would benefit from updated information that will point to more effective strategies for using current therapies than are now widely available.
In summary, despite the theoretical advantages of optimizing IV antibiotic dosing using PK/PD principles in patients with HAP, major gaps in the available evidence preclude our drawing conclusions or examining clinical or policy implications. The near absence of strong evidence, particularly related to clinical applications, has severely limited the broad adoption of PK/PD dosing optimization in the clinical arena. Below we address the gaps in evidence that might point to additional needed research and to the methods shortcomings in the studies that we were able to use.
Applicability
Based on the guidelines from the AHRQ Methods Guide, we found no robust studies addressing the applicability of PK/PD in relation to our PICOTS structure. Studies instead evaluated the measurement of absolute rather than relative benefits and harms, addressed heterogeneous treatment effects, and included diverse patient populations.
Research Gaps
First, whether use of PK/PD measures for informing dosing decisions for patients with HAP influences clinical outcomes remains unknown, largely because of both the absence of studies and the questionable quality of many of those studies (leading to imprecise findings). As noted, half of the included studies were rated as high risk of bias because of numerous problems with their design or conduct. Moreover, the available study populations were sufficiently diverse that they cannot be expected to produce “consistent” findings (and in fact did not).
Second, two key topics were not addressed in most investigations: (1) use of targeted and monitored antibiotic concentrations to tailor antibiotic doses of individual patients and (2) broad applications of PK/PD concepts such as using extended or prolonged infusions of time-dependent antibiotics. Although several studies have reported PK endpoints and findings from Monte Carlo simulated datasets, few in vivo studies have been designed to evaluate clinical endpoints. Such endpoints might include the types of intermediate outcomes we sought—such as immediate clinical response or days on a ventilator—or preferably, patient-centered health outcomes, especially disease or death. In this review, only one RCT evaluated clinical outcomes in patients with HAP receiving continuous versus intermittent ceftazidime infusions.28
Third, the effect of optimizing antibiotic dosing based on PK/PD principles for patients with HAP who fall into various clinical or sociodemographic subgroups is not known. Specifically, pharmacokinetic variability based on patient-specific factors such as critical illness, body weight, renal function, or age may influence the magnitude of the effect of PK/PD dose optimization (assuming an effect exists). The gaps in understanding the links among patient-specific factors, organism MIC, antibiotic dose, and clinical outcomes reflect the difficulty in isolating these variables and establishing cause-effect relationships. Elevated organism MICs, and thus antibiotic regimen and dosing choices, may be correlated with disease severity without having a causal effect. Furthermore, unmeasured organism factors such as virulence determinants, which may be associated with elevated MICs, may play a role in patient outcomes. These potential confounding variables should be considered when drawing conclusions about the effects of antibiotic dose optimization on patient outcomes.39-41
Finally, optimizing PK in dosing strategies in the clinical setting may delay the development of antimicrobial resistance. Resistant organisms are a persistent and increasing problem, with MRSA infections now accounting for more deaths than AIDS in the United States. Resistance among Gram-negative organisms is particularly concerning because of the scarcity of new drugs in development with activity against these pathogens. A possible contributor to this emerging resistance is today's approach to antibiotic dosing, which is based on the assumptions outlined above for PK/PD. Because present dosing recommendations are based largely on PK/PD studies in healthy volunteers, the recommendations may lead to suboptimal clinical outcomes in patients with HAP (or VAP or HCAP). Furthermore, subtherapeutic concentrations of antibiotics may further contribute to the survival and growth of resistant organisms.
Future investigations could be conducted in large-scale blinded prospective designs intended to compare different PK/PD strategies in patients with HAP. The two primary goals of such investigations are (1) to document the impact of different dosing strategies on meaningful clinical and patient-centered endpoints, such as survival in different patient populations, and (2) to determine their effects on the development of antibiotic resistance in bacteria. In addition, such studies can provide important data on other outcomes of interest to both clinicians and patients; these include ventilator days, rates of relapse, rates of reinfection, mortality risk, and timeliness of laboratory results in terms of being clinically useful in managing treatment. Measuring microbiological outcomes such as eradication of bacteria, microbiologic relapse, decrease in colony counts of culture, and development of antibiotic resistance can also yield information useful for developing dosing guidelines and recommendations. For certain patient-centered outcomes, such as clinical response and treatment failure not otherwise explained, clearly identifying how the investigators defined those outcomes (e.g., clinician judgment of patient signs and symptoms, laboratory values, quality of life assessed through patient self-reports, or mortality as measured at specific points in time) will improve interpretation of the findings. We believe research teams should be precise in delineating their conceptualization of all such outcomes.
Although antibiotic resistance clearly can arise during or from antibiotic treatment, less is known about the relationships among drug dosage, PK/PD optimization, and the development of resistance. Evaluating either the development or the prevention of resistance is a difficult research endeavor. Nevertheless, investigators can institute several approaches such as monitoring resistance trends in individual patients or tracking changes in hospital or local susceptibility patterns over time. Metrics for evaluating the development of resistance should be tested and validated in relationship to meaningful clinical and ultimate health outcomes. Researchers mounting PK/PD studies would then have more reliable and valid ways to examine this very important public health concern.
Conclusions
In the setting of increasing antimicrobial resistance worldwide and limited new antibiotics in the pipeline, optimizing dosing with PK/PD strategies could serve as an important antimicrobial stewardship tool to improve the use of currently available antibiotics. While PK/PD dosing strategies are supported by concept, the lack of prospective patient outcome data leaves clinicians with little guidance on how to best apply these principles to patient care. This review highlights the significant need for additional research to illuminate the role of antibiotic PK/PD dose optimization for the treatment of HAP.
References
- 1.
- American Thoracic Society. Infectious Diseases Society of America. Guidelines for the management of adults with hospital-acquired, ventilator-associated, and healthcare-associated pneumonia. Am J Respir Crit Care Med. 2005 Feb 15;171(4):388–416. Epub: 2005/02/09. [PubMed: 15699079]
- 2.
- Chawla R. Epidemiology, etiology, and diagnosis of hospital-acquired pneumonia and ventilator-associated pneumonia in Asian countries. Am J Infect Control. 2008 May;36(4 Suppl):S93–100. Epub: 2008/05/28. [PubMed: 18468551]
- 3.
- Vincent JL, Rello J, Marshall J, et al. International study of the prevalence and outcomes of infection in intensive care units. JAMA. 2009 Dec 2;302(21):2323–9. Epub: 2009/12/03. [PubMed: 19952319]
- 4.
- Forel JM, Voillet F, Pulina D, et al. Ventilator-associated pneumonia and ICU mortality in severe ARDS patients ventilated according to a lung-protective strategy. Crit Care. 2012;16(2):R65. Epub: 2012/04/25. [PMC free article: PMC3681394] [PubMed: 22524447]
- 5.
- Valles J, Peredo R, Burgueno MJ, et al. Efficacy of single-dose antibiotic against early-onset pneumonia in comatose patients who are ventilated. Chest. 2013 May;143(5):1219–25. Epub: 2013/05/30. [PubMed: 23715136]
- 6.
- Agrafiotis M, Siempos II, Ntaidou TK, et al. Attributable mortality of ventilator-associated pneumonia: a meta-analysis. Int J Tuberc Lung Dis. 2011 Sep;15(9):1154–63. i–v. Epub: 2011/06/15. [PubMed: 21669028]
- 7.
- Timsit JF, Zahar JR, Chevret S. Attributable mortality of ventilator-associated pneumonia. Curr Opin Crit Care. 2011 Oct;17(5):464–71. Epub: 2011/08/17. [PubMed: 21844801]
- 8.
- Seymann GB, Di Francesco L, Sharpe B, et al. The HCAP gap: differences between self-reported practice patterns and published guidelines for health care-associated pneumonia. Clin Infect Dis. 2009 Dec 15;49(12):1868–74. Epub: 2009/11/17. [PubMed: 19911940]
- 9.
- Zilberberg MD, Shorr AF, Micek ST, et al. Antimicrobial therapy escalation and hospital mortality among patients with health-care-associated pneumonia: a single-center experience. Chest. 2008 Nov;134(5):963–8. Epub: 2008/07/22. [PubMed: 18641103]
- 10.
- Kollef MH, Sherman G, Ward S, et al. Inadequate antimicrobial treatment of infections: a risk factor for hospital mortality among critically ill patients. Chest. 1999 Feb;115(2):462–74. Epub: 1999/02/23. [PubMed: 10027448]
- 11.
- Iregui M, Ward S, Sherman G, et al. Clinical importance of delays in the initiation of appropriate antibiotic treatment for ventilator-associated pneumonia. Chest. 2002 Jul;122(1):262–8. Epub: 2002/07/13. [PubMed: 12114368]
- 12.
- McKinnon PS, Paladino JA, Schentag JJ. Evaluation of area under the inhibitory curve (AUIC) and time above the minimum inhibitory concentration (T>MIC) as predictors of outcome for cefepime and ceftazidime in serious bacterial infections. Int J Antimicrob Agents. 2008 Apr;31(4):345–51. Epub: 2008/03/04. [PubMed: 18313273]
- 13.
- Olofsson SK, Cars O. Optimizing drug exposure to minimize selection of antibiotic resistance. Clin Infect Dis. 2007 Sep 1;45 Suppl 2:S129–36. Epub: 2007/08/19. [PubMed: 17683017]
- 14.
- Olofsson SK, Marcusson LL, Stromback A, et al. Dose-related selection of fluoroquinolone-resistant Escherichia coli. J Antimicrob Chemother. 2007 Oct;60(4):795–801. Epub: 2007/07/20. [PubMed: 17635875]
- 15.
- Roberts JA, Kruger P, Paterson DL, et al. Antibiotic resistance--what's dosing got to do with it? Crit Care Med. 2008 Aug;36(8):2433–40. Epub: 2008/07/04. [PubMed: 18596628]
- 16.
- Drusano GL. Antimicrobial pharmacodynamics: critical interactions of ‘bug and drug’ Nat Rev Microbiol. 2004 Apr;2(4):289–300. Epub: 2004/03/20. [PubMed: 15031728]
- 17.
- Chung DR, Song JH, Kim SH, et al. High prevalence of multidrug-resistant nonfermenters in hospital-acquired pneumonia in Asia. Am J Respir Crit Care Med. 2011 Dec 15;184(12):1409–17. Epub: 2011/09/17. [PubMed: 21920919]
- 18.
- Rello J, Ulldemolins M, Lisboa T, et al. Determinants of prescription and choice of empirical therapy for hospital-acquired and ventilator-associated pneumonia. Eur Respir J. 2011 Jun;37(6):1332–9. Epub: 2010/09/18. [PubMed: 20847075]
- 19.
- Rybak M, Lomaestro B, Rotschafer JC, et al. Therapeutic monitoring of vancomycin in adult patients: a consensus review of the American Society of Health-System Pharmacists, the Infectious Diseases Society of America, and the Society of Infectious Diseases Pharmacists. Am J Health Syst Pharm. 2009 Jan 1;66(1):82–98. Epub: 2008/12/25. [PubMed: 19106348]
- 20.
- Peterson LR. Bad bugs, no drugs: no ESCAPE revisited. Clin Infect Dis. 2009 Sep 15;49(6):992–3. Epub: 2009/08/22. [PubMed: 19694542]
- 21.
- Centers for Disease Control and Prevention. Antibiotic Resistance Threats in the United States, 2013. Atlanta, GA: CDC; 2013. [September 16, 2013]. www
.cdc.gov/drugresistance /threat-report-2013. - 22.
- Viswanathan M, Ansari MT, Berkman ND, et al. Assessing the Risk of Bias of Individual Studies in Systematic Reviews of Health Care Interventions Methods Guide for Effectiveness and Comparative Effectiveness Reviews. Agency for Healthcare Research and Quality; Rockville MD: 2008. AHRQ Pub. No. 12-EHC047-EF.
- 23.
- Owens DK, Lohr KN, Atkins D, et al. AHRQ series paper 5: grading the strength of a body of evidence when comparing medical interventions--Agency for Healthcare Research and Quality and the effective health-care program. J Clin Epidemiol. 2010 May;63(5):513–23. Epub: 2009/07/15. [PubMed: 19595577]
- 24.
- Atkins D, Chang SM, Gartlehner G, et al. Assessing applicability when comparing medical interventions: AHRQ and the Effective Health Care Program. J Clin Epidemiol. 2011 Nov;64(11):1198–207. Epub: 2011/04/06. [PubMed: 21463926]
- 25.
- Nicolau DP, Lacy MK, McNabb J, et al. Pharmacokinetics of continuous and intermittent ceftazidime in intensive care unit patients with nosocomial pneumonia. Infect Dis Clin Pract. 1999;8(1):45–9.
- 26.
- McNabb JJ, Nightingale CH, Quintiliani R, et al. Cost-effectiveness of ceftazidime by continuous infusion versus intermittent infusion for nosocomial pneumonia. Pharmacotherapy. 2001 May;21(5):549–55. Epub: 2001/05/15. [PubMed: 11349744]
- 27.
- Sakka SG, Glauner AK, Bulitta JB, et al. Population pharmacokinetics and pharmacodynamics of continuous versus short-term infusion of imipenem-cilastatin in critically ill patients in a randomized, controlled trial. Antimicrob Agents Chemother. 2007 Sep;51(9):3304–10. Epub: 2007/07/11. [PMC free article: PMC2043189] [PubMed: 17620371]
- 28.
- Nicolau DP, McNabb J, Lacy MK, et al. Continuous versus intermittent administration of ceftazidime in intensive care unit patients with nosocomial pneumonia. Int J Antimicrob Agents. 2001 Jun;17(6):497–504. Epub: 2001/06/09. [PubMed: 11397621]
- 29.
- Nicolau DP, McNabb J, Lacy MK, et al. Pharmacokinetics and pharmacodynamics of continuous and intermittent ceftazidime during the treatment of nosocomial pneumonia. Clin Drug Invest. 1999(2):133–9.
- 30.
- Hanes SD, Wood GC, Herring V, et al. Intermittent and continuous ceftazidime infusion for critically ill trauma patients. Am J Surg. 2000 Jun;179(6):436–40. Epub: 2000/09/27. [PubMed: 11004326]
- 31.
- Jaruratanasirikul S, Wongpoowarak W, Kositpantawong N, et al. Pharmacodynamics of doripenem in critically ill patients with ventilator-associated Gram-negative bacilli pneumonia. Int J Antimicrob Agents. 2012 Nov;40(5):434–9. Epub: 2012/09/11. [PubMed: 22959555]
- 32.
- Wang D. Experience with extended-infusion meropenem in the management of ventilator-associated pneumonia due to multidrugresistant Acinetobacter baumannii. Int J Antimicrob Agents. 2009;33:290–1. [PubMed: 19091523]
- 33.
- Scaglione F, Esposito S, Leone S, et al. Feedback dose alteration significantly affects probability of pathogen eradication in nosocomial pneumonia. Eur Respir J. 2009 Aug;34(2):394–400. Epub: 2009/02/14. [PubMed: 19213786]
- 34.
- Fahimi F, Ghafari S, Jamaati H, et al. Continuous versus intermittent administration of piperacillin-tazobactam in intensive care unit patients with ventilator-associated pneumonia. Indian J Crit Care Med. 2012 Jul;16(3):141–7. [PMC free article: PMC3506071] [PubMed: 23188954]
- 35.
- Lorente L, Jimenez A, Martin MM, et al. Clinical cure of ventilator-associated pneumonia treated with piperacillin/tazobactam administered by continuous or intermittent infusion. Int J Antimicrob Agents. 2009 May;33(5):464–8. Epub: 2009/01/20. [PubMed: 19150225]
- 36.
- Society for Healthcare Epidemiology of America; Infectious Diseases Society of America; Pediatric Infectious Diseases Society. Policy statement on antimicrobial stewardship by the Society for Healthcare Epidemiology of America (SHEA), the Infectious Diseases Society of America (IDSA), and the Pediatric Infectious Diseases Society (PIDS). Infect Control Hosp Epidemiol. 2012 Apr;33(4):322–7. Epub: 2012/03/16. [PubMed: 22418625]
- 37.
- Goff DA, Nicolau DP. When pharmacodynamics trump costs: an antimicrobial stewardship program's approach to selecting optimal antimicrobial agents. Clin Ther. 2013 Jun;35(6):766–71. Epub: 2013/06/26. [PubMed: 23795574]
- 38.
- Dellit TH, Owens RC, McGowan JE Jr, et al. Infectious Diseases Society of America and the Society for Healthcare Epidemiology of America guidelines for developing an institutional program to enhance antimicrobial stewardship. Clin Infect Dis. 2007 Jan 15;44(2):159–77. Epub: 2006/12/19. [PubMed: 17173212]
- 39.
- Holland TL, Fowler VG Jr. Vancomycin minimum inhibitory concentration and outcome in patients with Staphylococcus aureus bacteremia: pearl or pellet? J Infect Dis. 2011 Aug 1;204(3):329–31. Epub: 2011/07/12. [PubMed: 21742827]
- 40.
- Holmes NE, Turnidge JD, Munckhof WJ, et al. Antibiotic choice may not explain poorer outcomes in patients with Staphylococcus aureus bacteremia and high vancomycin minimum inhibitory concentrations. J Infect Dis. 2011 Aug 1;204(3):340–7. Epub: 2011/07/12. [PubMed: 21742831]
- 41.
- Holmes NE, Turnidge JD, Munckhof WJ, et al. Vancomycin minimum inhibitory concentration, host comorbidities and mortality in Staphylococcus aureus bacteraemia. Clin Microbiol Infect. 2013 Dec;19(12):1163–8. Epub: 2013/02/28. [PubMed: 23441652]
- Executive Summary - Pharmacokinetic/Pharmacodynamic Measures for Guiding Antibio...Executive Summary - Pharmacokinetic/Pharmacodynamic Measures for Guiding Antibiotic Treatment for Hospital-Acquired Pneumonia
- Preface - Comparison of Characteristics of Nursing Homes and Other Residential L...Preface - Comparison of Characteristics of Nursing Homes and Other Residential Long-Term Care Settings for People With Dementia
- Study Results - Venous Thromboembolism Prophylaxis in Major Orthopedic Surgery: ...Study Results - Venous Thromboembolism Prophylaxis in Major Orthopedic Surgery: Systematic Review Update
- Placebo-Controlled Meta-Analyses - Comparative Effectiveness and Safety of Oral ...Placebo-Controlled Meta-Analyses - Comparative Effectiveness and Safety of Oral Diabetes Medications for Adults With Type 2 Diabetes
- Analytic Framework - Improving Cultural Competence to Reduce Health DisparitiesAnalytic Framework - Improving Cultural Competence to Reduce Health Disparities
Your browsing activity is empty.
Activity recording is turned off.
See more...