NCBI Bookshelf. A service of the National Library of Medicine, National Institutes of Health.
Lux LJ, Posey RE, Daniels LS, et al. Pharmacokinetic/Pharmacodynamic Measures for Guiding Antibiotic Treatment for Hospital-Acquired Pneumonia [Internet]. Rockville (MD): Agency for Healthcare Research and Quality (US); 2014 Nov. (Comparative Effectiveness Reviews, No. 136.)
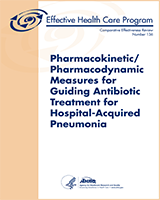
Pharmacokinetic/Pharmacodynamic Measures for Guiding Antibiotic Treatment for Hospital-Acquired Pneumonia [Internet].
Show detailsBackground
Hospital-Acquired Pneumonia: Epidemiology
Hospital-acquired (or nosocomial) pneumonia (HAP) is the second most common hospital-acquired infection. It occurs especially in the elderly, immunocompromised patients, surgical patients, and individuals receiving enteral feeding through a nasogastric tube. The incidence rates for HAP, which can occur in all areas of hospitals, range from 5 to more than 20 per 1,000 admissions.1,2
HAP is the leading cause of hospital-acquired infection in the intensive care unit (ICU).1 Almost one-third of HAP episodes are acquired in ICUs;3 as many as 90 percent of ICU cases may be ventilator associated.3,4 In the ICU setting, HAP accounts for up to 25 percent of all infections and for more than 50 percent of the antibiotics prescribed.1
Guidelines issued in 2005 by the American Thoracic Society (ATS) and the Infectious Diseases Society of America (IDSA) described HAP and two related pneumonias, ventilator-associated pneumonia (VAP) and health-care-associated pneumonia (HCAP).1 Briefly:
- HAP is a pneumonia that occurs 48 hours or more after admission and was not incubating at the time of admission.
- VAP is a pneumonia that presents more than 48 to 72 hours after endotracheal intubation. It is a severe type of HAP; because of the difficulty in treating it, its prognosis can be poor.
- HCAP is a pneumonia that develops in any patient who meets one or more of several criteria: had been hospitalized in an acute care hospital for 2 or more days within 90 days of the infection; had resided in a nursing home or long-term care facility; had received recent intravenous (IV) antibiotic therapy, chemotherapy, or wound care within the past 30 days of the current infection; or had attended a hospital or hemodialysis clinic.
Unless we specify otherwise, the term “HAP” includes VAP and HCAP throughout the report. Most biological and clinical principles for HAP and VAP overlap those for HCAP.
HAP is most often caused by bacterial pathogens, and it may be polymicrobial. Staphyloccus aureus (S. aureus)—especially methicillin-resistant S. aureus (MRSA)—and aerobic Gram-negative bacilli, including Pseudomonas aeruginosa, Escherichia coli, Klebsiella pneumoniae, and Acinetobacter species, are the common causes of HAP. HAP caused by S. aureus is found with greater frequency in patients with diabetes mellitus, patients with head trauma, and patients hospitalized in ICUs. HAP caused by viral or fungal pathogens is rare in immunocompetent patients.1,5
Because HAP, VAP, and HCAP share similar microbial sources, they are treated similarly. The general approach is to treat broadly for resistant Gram-positive and Gram-negative organisms, then de-escalate therapy when the pathologic agent is defined. Clinicians may manage HAP patients in a hospital ward or in an ICU when the illness is more severe. Some patients may require intubation after developing severe HAP; in these cases, clinicians should treat them in ways similar to treating patients with VAP.
HAP is associated with increased morbidity and mortality, longer lengths of inpatient stays, and higher costs of care despite advances in antimicrobial therapy, supportive care, and prevention. For example, episodes of HAP that are not associated with ventilator use raise both hospital lengths of stay and costs of care; in one report from Asian countries, they were associated with death rates of between 27 percent and 50 percent.2
Concerns in the United States and abroad about the increasing rates of superinfection (i.e., infection with a new organism) and multidrug-resistant pathogens call for strategies to optimize existing antibiotic treatment for HAP.6,7 Gram-negative pathogens, such as Pseudomonas aeruginosa and Acinetobacter species, are of particular concern because of increasing rates of resistance and lack of effective antibiotic options for treatment. Pneumonia caused by MRSA is also a concern because of the emergence of strains with decreased susceptibility to vancomycin, reports of poor clinical outcomes, and increased risks of toxicities associated with increasing vancomycin doses.
Patients who have received mechanical ventilation are at the greatest risk for HAP; intubation increases a patient's HAP risk by 6 to 21 times. Mortality from VAP among patients who have acquired VAP in ICUs can be higher for patients who receive inadequate empirical therapy.8 Additional costs per episode of VAP may be as high as $40,000.9
Beyond mechanical ventilation, numerous other factors may increase a patient's risk for HAP.10-21 These variables include:
- Age >60 years
- Chronic lung disease
- Presence of various underlying illness
- Depressed consciousness
- Aspiration
- Use of acid-suppressing medications
- Use of paralytic agents
- Previous antibiotic exposure, particularly to third-generation cephalosporins
- Mechanical ventilation for acute respiratory distress syndrome
- Reintubation or prolonged intubation
- Frequent ventilator circuit changes
- Chest surgery
- Transport from the ICU for diagnostic or therapeutic procedures
- Presence of an intracranial pressure monitor or nasogastric tube
- Hospitalization during the fall or winter season
HAP Infection: Treatment
Appropriate antibiotic therapy significantly improves survival for patients with HAP.22-25 Relevant antibiotics for treating HAP patients include broad-spectrum beta-lactams, vancomycin, and aminoglycosides, among others. Table 1 lists antibiotic classes and individual agents that clinicians might use to treat HAP; bold items are those used most often.
Table 1
Intravenous antibiotics for which PK/PD measures could be used.
Optimal treatment involves choosing the right drug or combination of drugs, the proper dose and route of administration, and the appropriate duration, followed by de-escalation to pathogen-directed therapy once culture and susceptibility results are known.1 Subtherapeutic dosing of antibiotics has been associated with poorer clinical outcomes and emergence of antibiotic resistance.26-29
Optimal dosing of antibiotics based on principles of pharmacokinetics and pharmacodynamics (PK/PD) has the potential to improve outcomes and prevent the development of resistance in patients with HAP. PK is the study of the time course of drug absorption, distribution, metabolism, and excretion. The primary goals of clinical PK include enhancing efficacy and decreasing toxicity of an individual patient's drug therapy. PD refers to the relationship between the concentration of the drug at the site of action and the resulting effect. Antibiotic PD relates PK parameters to the ability of an antibiotic to kill or inhibit growth of bacterial pathogens.30
To improve the effectiveness of the available antibiotics specifically for HAP, the 2005 ATS/IDSA guidelines recommended considering PK/PD properties when selecting an antibiotic regimen, dosage, and route of administration. The goal of these guidelines is to provide recommendations for the selection of adequate therapy and thereby achieve optimal patient outcomes. This antibiotic dosing logic is based on serum antibiotic concentrations in healthy volunteers in in vitro and in vivo observations. For those reasons, it may not account fully for the heterogeneity of patient populations with HAP, the complex pathologic environment in the infected lung, and the drug concentration achieved at the site of the pneumonia. Current antibiotic dosing strategies also do not directly consider the variety of antibiotic resistance mechanisms in bacteria that contribute to the persistence of HAP.
Furthermore, measuring PK/PD only in the serum may lead to suboptimal antibiotic concentrations at the site of infection—in this case, the lung. In such cases, the antibiotic may not eradicate resistant organisms; this problem may in turn lead to treatment failure and contribute to emerging antibiotic resistance. Generally speaking, given the unique attributes of the lung that contribute to the challenge of adequately treating patients with HAP, these issues are of special concern for clinicians and others in providing fully successful services for such patients.
Categorizing antibiotics according to their PD parameters (time-dependent or concentration-dependent) is based on data relating antibiotic activity to serum drug concentrations rather than to concentrations at the site of the infection (such as the lung). Furthermore, susceptibility interpretive criteria and breakpoint determinations (MIC data) are based on established PK/PD concepts, which have been derived from serum drug concentrations. Often, dosing choices are based on assumptions that the concentration of the antibiotic at the site of infection is equal to the concentration observed in the serum.
A few studies have reported on drug penetration into the lung, generally measured as alveolar concentrations or epithelial lining fluid (ELF) concentrations. However, PD relationships and specific dosing inferences from these data have not been established. For many antibiotics, drug concentrations achieved within the lung are likely not to be equal to drug concentrations easily measured in the serum. Lodise et al. determined ELF concentrations of vancomycin in healthy patients;31 they found that vancomycin penetrates ELF at approximately 50 percent of plasma levels, with a high level of variability among their measurements. In contrast, studies evaluating the penetration of linezolid into the lung have shown that linezolid achieves concentrations within the lung that are equal to or higher than concurrent concentrations in the serum.32,33 Differences in chemical properties of drugs and differences in patient charachteristics such as lung inflammation also influence the penetration of drugs into the lung.34
Concerns in the United States and abroad about the increasing rates of superinfection and new resistance patterns in pathogens call for strategies to optimize existing antibiotic treatment options for HAP.6,7 Antibiotic resistance is a growing and significant threat to public health. The incidence rates of drug resistance among many common HAP pathogens have increased dramatically over the past 3 decades. During the same period of time, the number of new antibiotics has decreased, especially for drugs that target Gram-negative organisms. In addition, treatment of MRSA pneumonia has become more difficult because of rising incidence of infections caused by isolates with increased minimal inhibitory concentrations (MICs) to vancomycin (“MIC creep”). To reach proposed pharmacodyamic targets, higher doses of vancomycin are needed, which increases risks of toxicities.35 With fewer antibiotic options, ensuring the appropriate and judicious use of these drugs becomes increasingly important.36,37
Although optimization of antibiotic dosing is important to improve individual patient outcomes with HAP, optimal antimicrobial exposure may also serve to prevent the emergence of resistant populations of organisms. Subtherapeutic concentrations of antibiotics may contribute to the emergence or acceleration of resistance. Consequently, the use of PK/PD measures to guide dosing of antibiotics has important implications, not only for the individual patient being treated, but also for public health concerns. The correlation between the emergence of resistance and clinical outcomes is not fully understood, but we believe that the emergence of resistance is an important patient and societal concern. Its usefulness as a surrogate marker for clinical outcomes, however, requires further study.
Use of Pharmacokinetic and Pharmacodynamic Measures for Dosing and Monitoring of Antibiotics
This review aims to document the impact of contemporary approaches to PK/PD-guided dosing of IV antibiotic therapy on clinical outcomes for patients with HAP. In general, antibiotics are grouped into one of three categories based on their mode of bacterial killing: (1) concentration dependent, (2) time dependent, or (3) a combination of concentration and time dependent. These three modes are expressed as ratios to the MIC of the organisms (Figure 1).
- Concentration-dependent antibiotic: Peak concentration to MIC (expressed as Cmax/MIC)
- Time-dependent antibiotic: Time that the serum concentration is greater than the MIC (expressed as T>MIC)
- Area under the concentration-time curve (AUC) to MIC (expressed as AUC/MIC)
Given the PK/PD properties of antibiotics, clinicians can optimize the PD effects of antibiotics by making decisions about dosing strategies. For example, to optimize the PD effect of a concentration-dependent antibiotic, clinicians may choose to increase the dose, resulting in a higher Cmax/MIC ratio.
The traditional method of aminoglycoside dosing has been to divide the total daily dose into two or three equal doses. Based on PD evidence revealing concentration-dependent action, however, many clinicians have adopted the practice of administering aminoglycosides using an extended-interval dosing scheme; doing so enables them to take advantage of the concentration-dependent effects of the drug. A target of Cmax/MIC>10 has been proposed. This target is based on retrospective clinical data, including data in patients with HAP, correlating clinical response with specific Cmax/MIC targets.38,39 To achieve this target, the total aminoglycoside daily dose is administered as a single bolus infusion (i.e., a relatively large dose of medication administered into a vein in a short period) over 30 to 60 minutes instead of the traditional divided doses.
For time-dependent antibiotics such as beta-lactams, strategies of prolonged or continuous infusions have been employed to optimize the T>MIC ratio. The standard administration method for IV beta-lactam antibiotics is intermittent bolus dosing. PD data have shown, however, that administration of beta-lactam antibiotics by prolonged infusions produces a higher T>MIC ratio than does intermittent dosing. A target T>MIC of at least 50 to 70 percent of the dosing interval has been proposed based on studies in animal infection models.40-43 The use of prolonged or continuous infusions of beta-lactam antibiotics, instead of intermittent bolus dosing, should increase the percentage of time that antibiotic concentrations are above the MIC in the serum; this may correlate with efficacy, especially for organisms with high MICs.
For antibiotics in which the AUC/MIC ratio is the predictor of efficacy, such as vancomycin, clinicians can use concentration monitoring to achieve a specific AUC/MIC target to optimize dosing. Vancomycin monitoring guidelines were published in 2009 by the Society of Infectious Diseases Pharmacists, the American Society of Hospital Pharmacists, and the IDSA.44 These guidelines recommend a target AUC/MIC ratio of 400 for optimal efficacy for vancomycin. Because serum trough concentration monitoring (to determine the minimum concentration of a drug in the serum at the end of a dosing interval) is more practical than AUC monitoring in clinical settings, a goal trough concentration of 15 mg/L to 20 mg/L is recommended for the treatment of HAP caused by MRSA with an MIC≤1 mg/L. For more resistant organisms with an MIC>1 mg/L, the target AUC/MIC of 400 becomes more difficult with standard dosing. The recommendations from this guideline were based on PK analyses and retrospective, observational studies, including one retrospective investigation of patients with pneumonia caused by S. aureus.45 The clinical benefit of various vancomycin targets remains a subject of controversy.
PD targets become more difficult to achieve as the MIC for an organism increases and the organism becomes more resistant. As the prevalence of antibiotic-resistant bacteria continues to rise, particularly among critically ill patients, choosing the optimal antibiotic dosing regimen is important to increase the likelihood of clinical success. The optimal dosing regimen will achieve the appropriate PD target without increasing the risk of concentration-related toxicities. For drugs with a narrow therapeutic index (i.e., ones with little difference between toxic and subtherapeutic concentrations), such as vancomycin and the aminoglycosides, the risk of toxicities is often a dose-limiting factor.
The probability of attaining the PD target changes not only with the organism MIC but also with variations in patient-specific factors. The efficacy of an antibiotic depends on its ability to reach the site of infection in sufficient concentrations to inhibit bacterial activity.46 Optimizing PK/PD can increase the likelihood of obtaining adequate concentrations of the appropriate drug and enhancing outcomes for patients with HAP. However, in critically ill patients, alterations in fluid distribution, homeostasis, hemodynamic state, microcirculation, and organ function are common. These factors are essential to understanding and choosing an effective therapeutic regimen, and they can affect both PK and PD properties.46,47
A recent multicenter study demonstrated significant variability in antibiotic trough concentrations in critically ill patients who were receiving continuous renal replacement therapy; the intensity of continuous renal replacement therapy had not predicted such variability.48 This observation suggested that desirable clinical results cannot reliably be achieved with empiric dosing. Current recommended dosing strategies for HAP tend to be based on animal or in vitro models or on data from patients who are not critically ill. Today's guidance about HAP treatments typically does not account for these factors. This problem puts critically ill patients at risk of treatment failure, adverse effects from drug toxicity, antibiotic resistance, and death.
In their consensus document on controversial issues for treating critically ill patients with HAP, Franzetti et al. recommended using PK/PD parameters, particularly trough serum concentration monitoring for vancomycin.49 They based their guidance on evidence that optimizing PK/PD parameters may prevent treatment failure and resistance; it may also reduce nephrotoxicity (severe negative effects on the kidneys) in patients who are receiving aggressive dosing, concurrent nephrotoxic drugs, or prolonged courses of therapy and in patients with unstable renal function.
Scope and Key Questions
Scope of This Review
The main objective of this report is to document and present the findings from a systematic review of the evidence concerning use of PK/PD methods for treating HAP infections. We are not addressing community-acquired pneumonia or HAP in children or adolescents; we are also not addressing PK/PD applications for conditions other than pneumonia or organ systems other than the lungs. This focus responds to the major concerns of clinical groups that nominated the topic and the substantial challenges of successfully applying PK/PD methods to pneumonia.
As presented in thoroughly in Methods, we focus our analysis on detailed specifications for populations, interventions, comparators, outcomes, timing of measurement or followup, and settings (PICOTS). Briefly, populations include adults who have presumed or confirmed HAP, VAP, or HCAP and who are being treated with IV antibiotic treatment. We look at benefits defined for both intermediate outcomes (clinical response; use of ventilators) and health outcomes (morbidity and mortality); we also examine evidence about adverse events (harms). We examine evidence relating to HAP that begins in the hospital setting (e.g., emergency department, floor, or ICU) and relating to treatment that continues in other settings; we also include studies of patients who have acquired HAP in a nursing home setting.
This review is relevant to several dilemmas that clinicians face about how best to select doses and to monitor the use of IV antibiotics for these severely ill patients while taking account of the PD properties of different IV antibiotics, various patient-specific factors, and resistance patterns of the pathogens. Of concern are both presumed benefits and harms of using PK/PD measures for these purposes. We also attempt to address one very specific question concerning the beta-lactam class of antibiotics. Finally, we examine what may be known about how outcomes (benefits or harms) relate to patient populations characterized by sociodemographic or clinical characteristics.
Key Questions
We address three Key Questions (KQs). Figure 2 presents the analytic framework used to guide this review. The KQs and subquestions are noted in relationship to the direct or indirect linkages depicted in the figure.
- Key Question 1.
For people with hospital-acquired pneumonia, how does using PK/PD measures to inform decisions about dosing or monitoring antibiotic treatment affect:
- clinical response or mechanical ventilation?
- morbidity or mortality?
- rates of antibiotic-related adverse events?
- Key Question 2.
For people with hospital-acquired pneumonia, how does using prolonged or continuous infusions compared with bolus infusions for beta-lactams affect:
- clinical response or mechanical ventilation?
- morbidity or mortality?
- rates of antibiotic-related adverse events?
- Key Question 3.
For people with hospital-acquired pneumonia, does the evidence for clinical response, mechanical ventilation, morbidity, mortality, or antibiotic-related adverse events differ for subgroups defined by age, sex, race, ethnicity, renal dysfunction or need for dialysis, severity of illness, microorganism, or susceptibility patterns, when examining the use of PK/PD measures to inform decisions about dosing and monitoring antibiotic treatment or when comparing prolonged or continuous infusions versus bolus infusions for beta-lactams?
Organization of This Report
The remainder of the review describes our methods in detail and presents the results of our synthesis of the literature with summary tables and the strength of evidence grades for major comparisons and outcomes. The discussion section offers our conclusions, summarizes our findings, and provides other information relevant to interpreting this work for clinical practice and future research. References, a list of acronyms and abbreviations, and a glossary of terms follow the Discussion section.
Appendix A contains the exact search strings we used in our literature searches. Appendix B presents the risk of bias assessments of individual studies in this review. Studies excluded at the stage of reviewing full-text articles with reasons for exclusion are presented in Appendix C. Evidence tables appear in Appendix D.
- Introduction - Pharmacokinetic/Pharmacodynamic Measures for Guiding Antibiotic T...Introduction - Pharmacokinetic/Pharmacodynamic Measures for Guiding Antibiotic Treatment for Hospital-Acquired Pneumonia
- Preface - Effectiveness of Early Diagnosis, Prevention, and Treatment of Clostri...Preface - Effectiveness of Early Diagnosis, Prevention, and Treatment of Clostridium difficile Infection
- Acronyms and Abbreviations - Self-Measured Blood Pressure Monitoring: Comparativ...Acronyms and Abbreviations - Self-Measured Blood Pressure Monitoring: Comparative Effectiveness
- Streptococcus agalactiae strain DK-NI-013, whole genome shotgun sequenceStreptococcus agalactiae strain DK-NI-013, whole genome shotgun sequencegi|942540123|ref|NZ_CHDT01000002.1|Nucleotide
- References - The Role of Immunotherapy in the Treatment of AsthmaReferences - The Role of Immunotherapy in the Treatment of Asthma
Your browsing activity is empty.
Activity recording is turned off.
See more...