NCBI Bookshelf. A service of the National Library of Medicine, National Institutes of Health.
Madame Curie Bioscience Database [Internet]. Austin (TX): Landes Bioscience; 2000-2013.
The nuclear lamina is a filamentous scaffold structure underneath the inner nuclear membrane and consists of A- and B-type lamins and a number of integral inner nuclear membrane proteins, such as lamin B receptor (LBR), emerin, and various isoforms of lamina-associated polypeptides 1 (LAP1) and LAP2. Lamins, LAP2, emerin and LBR interact with DNA and/or chromosomal proteins, including core histones, BAF, HP1 and HA95, and provide a complex dynamic link between the peripheral lamina and nucleoskeletal structures and chromatin fibers. In addition, components of nuclear pore complexes, such as Nup153 and Tpr, link the nuclear envelope to the nuclear interior. Furthermore, intranuclear complexes of A-type lamins and LAP2α are likely involved in higher order chromatin organization throughout the nucleus. These interactions are tightly regulated in a temporal and spatial manner during the cell cycle and are responsible for the multiple functions of the lamina in dynamic nuclear and chromatin structure organization, in DNA replication, gene transcription, cell cycle progression, and apoptosis.
Introduction
The eukaryotic nucleus contains the chromosomes and is a complex organelle where major cellular processes, such as DNA replication, RNA transcription and processing, and ribosome assembly take place. The function of the nucleus highly depends on its structural organization and the dynamic structural rearrangements occurring in cell differentiation and cell cycle progression.1 Cellular structures and proteins involved in nuclear architecture are not very well understood except for a few major elements. The nuclear envelope (NE) enwraps the DNA and forms the border between the nucleus and cytoplasm. It is composed of inner and outer nuclear membranes that are separated by the perinuclear space and contain nuclear pore complexes mediating nucleo-cytoplasmic transport. The outer nuclear membrane is continuous with the endoplasmic reticulum and is also directly linked to the inner membrane at sites of nuclear pore complexes (Fig. 1). Despite the continuity of membrane structures, protein and lipid composition and functions of inner and outer membranes are clearly different, most likely due to specific interactions of membrane components with nuclear and cytoplasmic components, respectively. Underneath the inner membrane is a meshwork of nuclear-specific intermediate filaments, termed the nuclear lamina, which includes lamins plus a growing number of lamin-associated proteins, which regulate lamin assembly and function.1,2 Most of these lamin-binding proteins have been identified as integral membrane proteins of the inner membrane or are tightly associated with the lipid bilayer.2-4 Biochemically, the nuclear lamina is defined as the peripheral nuclear structure that remains insoluble after extraction of nuclei with non-ionic detergents, salt and nucleases.5,6 However, the lamina is only a subfraction of the detergent-salt-resistant structural framework, which runs throughout the nuclear interior and organizes nuclear space and is often referred to as nucleoskeleton or nuclear matrix.7 As the visualization of this putative nuclear scaffold has always been hampered by the bulky chromatin mass, it is still under debate, whether there exists a chromatin-independent proteinaceous nuclear scaffold or whether intranuclear structures are organized by a complex network of protein-protein, protein-DNA and protein-RNA interactions.8 In any case, the nuclear scaffold is supposed to provide mechanical stability for nuclear structure, to form a platform for most metabolic nuclear processes, and to organize chromatin in a three-dimensional nuclear space and thus regulate gene expression at the chromatin structure level. Except for the peripheral lamins, the components and molecular organization of the nucleoskeleton are not very well understood.
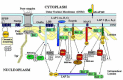
Figure 1
Schematic drawing of the molecular links at the interface between the nuclear envelope and the internal nucleoskeleton/chromatin scaffold. Arrows denote specific interactions of components shown in vitro and/or in vivo. For details see text.
In this review, I summarize the major components and interactions of the lamina and focus on the interface between the peripheral nuclear envelope and the intranuclear scaffold/chromatin. I will also describe cell cycle-dependent dynamics and potential functions of these interactions, particularly focusing on members of the Lamina-Associated Polypeptide 2 (LAP2) family, whose cell cycle-dependent dynamics have been fairly well characterized in the past years.
Major Components of the Peripheral Nuclear Lamina
The core structure of the nuclear lamina is formed by type V intermediate filament proteins, the lamins.9 They assemble to a meshwork of tetragonally organized 10-nm filaments underneath the inner nuclear membrane. The number and complexity of lamins has increased during metazoan evolution. Vertebrates have three lamin genes (LMNA, LMNB1, LMNB2) encoding at least seven distinct isoforms.2 B-type lamins are constitutively expressed in cells throughout development and every cell expresses at least one B-type lamin. A-type lamins, comprising lamin A and its smaller splice variant lamin C are only expressed in later stages of development and in differentiated cells.2
The assembly and attachment of lamins at the membrane involve several mechanisms and are different between A- and B-type lamins. B-type lamins contain a stable C-terminal farnesyl modification, which is important but not sufficient for targeting and anchoring the protein to the membrane.10-13 In contrast, lamin A is only transiently farnesylated due to cleavage of the C-terminal residues containing the farnesyl group during protein maturation, and lamin C is never farnesylated.10 Therefore, B-type lamins are more tightly associated with membrane structures than A-type lamins in mitosis and interphase and are less stably incorporated into the lamina.14,15
Homotypic interactions of lamin subunits,9 hetero-oligomeric interactions of B-type and A-type lamin dimers or oligomers,11 as well as interactions of lamins with integral and peripheral membrane proteins are essential for the proper assembly of the lamina underneath the nuclear membrane. Most of the lamin-binding proteins are tightly bound to lamins and cofractionate with lamins even after detergent-salt extraction of nuclei or of isolated NE fractions.5,6,16-18 Therefore, these proteins are considered as genuine components of the nuclear lamina. Among those are (see Fig. 1):
The Lamin B receptor (LBR, p58) contains eight transmembrane domains19 and was found to interact with B-type lamins in vivo and in vitro.20-22 Since ectopically expressed lamin B1 mutants lacking farnesylation segregate independently of LBR,12 it was suggested that LBR might also bind to the farnesyl residues of B-type lamins. The hydrophobic domain of LBR shares extensive homology with sterol reductases and exhibits C14 reductase activity, suggesting that the protein might have additional functions in sterol metabolism.23,24
Lamina-associated-polypeptide 1 (LAP-1) is a type II integral nuclear membrane protein, containing a nucleoplasmic N-terminus, a single transmembrane spanning region, and a C-terminus located in the luminal space between inner and outer nuclear membrane.25 LAP1 specifically interacts with A-type lamins in vitro6 and its nuclear envelope localization depends on the presence of lamin A in vivo.26 LAP1 exists as three alternatively spliced isoforms, the smallest one, LAP1C, being expressed constitutively, while the larger isoforms, LAP1 A and B, which contain additional domains in the nucleoplasm, are expressed only in differentiated cells like A-type lamins.25
Lamina-associated polypeptide 2 (LAP2) is another family of alternatively spliced lamin binding proteins, comprising up to six mammalian isoforms, LAP2α, β, γ, δ, ϵ, and ζ27,28 (formerly also called thymopoietins) and three Xenopus LAP2 proteins.29,30 Except for LAP2α and LAP2ζ, all mammalian LAP2 isoforms contain a closely related N-terminal nucleoplasmic domain of variable length and share a single transmembrane spanning region passing the inner nuclear membrane, and a short C-terminus located in the luminal space between inner and outer nuclear membrane.31 LAP2β possesses the longest nucleoplasmic N-terminus (223aa), LAP2 e, d, g miss regions of 40, 72, and 109 amino acids respectively due to alternative splicing, but are otherwise identical, and LAP2ζ represents a truncated form of LAP2β, missing 190 amino acids of the nucleoplasmic domain as well as the transmembrane and luminal regions. LAP2α is structurally and functionally different from the other isoforms. It shares only the N-terminal 187 amino acids with all the other LAP2 isoforms but contains a unique C-terminus (506 aa) lacking a transmembrane domain. Unlike LAP2α, LAP2β was found to interact with lamin B in vitro.6 Its lamin B-interaction domain was located within a 72-amino acid long stretch in the nucleoplasmic region (aa 298-370),32 which is also present in the smaller isoforms LAP2ϵ and d, and parts of it in LAP2γ. An interaction of the smaller isoforms with lamin B, however, has not been demonstrated experimentally. LAP2α is unique, as it is located in the nuclear interior33 and binds specifically intranuclear A-type lamins34 (see below). Very little is known about the expression patterns of the various LAP2 isoforms. Northern blot analysis and S1-nuclease protection assays revealed that LAP2 mRNAs are ubiquitously found in many tissues and cells of human, mouse and rat origin.27,28,35 At the protein level, LAP2α and β appear to be the predominantly expressed LAP2 isoforms in mammalian cells,27,33,36 but a recent proteomics analysis aimed at characterizing novel NE proteins clearly revealed also expression of smaller LAP2 isoforms.37 While available data on the mammalian LAP2 isoforms indicate an ubiquitous expression, some of the LAP2 homologues, identified in Xenopus,29,30 showed differential expression during development. One of them was found to be expressed only in somatic cells, but was not detected in oocytes, eggs and in early embryos up to the gastrula stage, while a slightly larger putative LAP2 isoform—which has not been cloned yet—was predominantly expressed in Xenopus eggs and embryos and was downregulated during embryogenesis.29
Emerin and MAN1 are proteins related to LAP2 isoforms. These proteins share a ˜40 amino acid long highly homologous structural motif (LEM domain) in their N-termini17, which consists of a helical turn and two large parallel α-helices connected by a 11 to 12 residue loop.38,39 Emerin is a ubiquitously expressed type II integral membrane protein of the inner nuclear membrane18,40 and has been identified as the gene product that is missing or mutated in patients suffering from X-linked Emery-Dreifuss muscular dystrophy (EDMD).41,42 It binds to both A- and B-type lamins in vitro 43-45 and its nuclear envelope localization is dependent on the expression of lamin A.46,47 MAN1 is a lamina-associated protein detected by the MAN autoimmune serum,48 and by sequence analysis is predicted to contain two transmembrane domains.17 Its interaction with lamins has not been analyzed yet.
A visual screen of a GFP-fusion library49 and a proteomics approach37 revealed novel integral membrane proteins termed nurim, with 5 predicted transmembrane domains and only few hydrophilic residues, Unc-84, a protein similar to Drosophila Unc-8450 and a novel protein LUMA with three to four predicted transmembrane domains. Binding of these proteins to lamins has not been analyzed yet.
In addition, a peripheral membrane protein, otefin,51,52 has been identified as a lamina protein in Drosophila.
Lamina Proteins in the Nuclear Interior
Lamins were traditionally regarded as proteins of the nuclear periphery, but with the availability of novel tools and microscopic techniques the concept of intranuclear lamins has recently developed. Since the nuclear membrane forms extensive tubular invaginations projecting deep into the nuclear interior,53 it is often hard to distinguish whether observed internal lamin structures are still associated with the invaginated nuclear membrane or whether they are truly intranuclear, apart from the nuclear membrane. Nevertheless, specific antibodies,34,54 several microscopical preparations techniques,55,56 and the use of expressed green fluorescent protein-(GFP) fusions of lamins,57,58 have revealed intranuclear lamin structures in foci or along filamentous structures or diffusely distributed throughout the nuclear interior. Although B-type lamins may also localize to intranuclear replication sites59 and a minor fraction of GFP-lamin B has been detected in stable intranuclear structures by fluorescence recovery after photobleaching (FRAP) analysis,58 the majority of studies have revealed particularly A-type lamins in the nuclear interior. This observation is consistent with the lack of C-terminal farnesyl modification of mature A-type lamins and the less stable association with the peripheral nuclear membrane and the nuclear lamina as compared with B-type lamins (see above).
Intranuclear A-type lamins may exist in a complex with LAP2α, the only LAP2 isoform not integrated into the membrane. LAP2α is a nucleoskeletal protein, based on its resistance to extraction by detergents and high salt,33 and was found to directly interact with the C-terminal tail region of mature lamins A and C in vitro.34 Furthermore, selective disruption of endogenous lamin A structures upon ectopic expression of dominant-negative lamin mutants in Hela cells caused a relocalization of LAP2α to intranuclear lamin A/C aggregates, but had no effect on lamin B, LAP2β, or NuMa.34 It is still unclear, however, whether lamin A and LAP2α form filaments or other higher order structures of the nuclear scaffold, or whether they exist as smaller complexes involved in the regulation of nuclear processes (see below). It is also not known, whether there is continuity between peripheral and internal nuclear lamin A structures or whether lamin subunits steadily exchange between these two subnuclear compartments.
Several laboratories have reported a transient localization of A-type lamins in the nuclear interior before their assembly into the nuclear lamina. FRAP analyses in GFP-lamin A expressing cells showed that the assembly of lamin A into peripheral nuclear structures is a late event in post-mitotic nuclear reformation,58 leading to accumulation of the majority of lamins A and C in the nuclear interior in G1 phase.34,60 Furthermore, microinjected lamin A and/or lamin C were found to first accumulate in nucleoplasmic foci, before the majority was incorporated into the nuclear lamina.61,62 As non-processed lamin A (missing the farnesyl modification) accumulated in similar intranuclear foci,63,64 transient intranuclear localization of lamin A might be directly linked to its post-translational processing, but the molecular mechanisms remain unclear. Recently, a novel nuclear protein of unknown cellular function, Narf, has been identified by yeast two-hybrid-screens as a direct and specific interaction partner of unprocessed lamin A.65
Farnesylation and C-terminal proteolytic cleavage of A-type lamins during maturation can, however, not be the only reason for their transient accumulation in the nucleus, as intranuclear lamin A found in late stages of post-mitotic nuclear reformation is fully processed, and lamin C, which was also found to accumulate in intranuclear structures,62 is not processed at all. Thus, other modifications such as (de-) phosphorylation,66 or specific interactions with still unknown nuclear proteins might also be required for correct targeting of A-type lamins to peripheral as well as intranuclear structures.
Interactions at the Interface Between the Lamina and the Nuclear Scaffold/Chromatin
The transcriptionally silenced or less active and late replicating domains in higher eukaryotic genomes, referred to as heterochromatin, are dynamically associated with the NE.67-69 This association involves a complex network of specific protein-protein and protein-DNA interactions at the interface of the lamina and the nuclear matrix (Fig. 1). In vitro, A and B-type lamins have been shown to bind directly to matrix/scaffold attachment regions70,71 and to telomeric and heterochromatic DNA sequences,72,73 but the physiological relevance of these associations is not clear. However, photo-crosslinking experiments in Drosophila cells revealed specific association of interphase lamins with DNA in vivo.74 Lamins can also interact with and assemble around chromatin, and this is mediated by the lamin rod domain75 and/or the C-terminal tail domain that binds to core histones.76,77
In addition to lamins, many lamin-binding proteins were shown to interact with DNA and/or chromosomal protein (Fig. 1). LBR interacts directly with DNA,2278, and with human HP1-type chromodomain proteins,79,80 which function as chromatin modifiers and regulators of gene expression and have been implicated in position effect variegation and heterochromatin organization.81,82 In line with these findings, microinjected HP1 has been shown to localize transiently at the nuclear periphery in a deacetylation-dependent manner, before it translocates to intranuclear sites.83 The association of HP1 with the nuclear envelope may be mediated by direct binding to LBR, but recent studies revealed a complex of LBR, HP1 and histones H3/H4, in which histones bind to both LBR and HP1 and mediate the LBR-HP1 interaction.84 As the interaction of histones with HP1 was found to be affected by methylation of histone at lysine residue 9,85,86 this could also provide a regulatory mechanisms for chromatin docking at the cellular periphery during cell proliferation and cell differentiation. In cross-linking studies LBR was also found to associate with chromatin-associated HA95, a nuclear protein with high homology to the nuclear A-kinase anchoring protein AKAP95.87
LAP2 proteins contain several chromatin and/or DNA binding domains, which are either common to all or unique to some isoforms (Fig. 1). The constant N-terminal region, common to all LAP2 proteins, contains the LEM domain (amino acids 111-152), which was found by yeast two hybrid assay88 and by biochemical studies89 to interact with the chromosomal protein Barrier-to-Autointegration Factor (BAF), a protein that was first identified for its role in retroviral DNA integration.90,91 Further studies revealed that BAF is a 89-residue protein that is highly conserved in multicellular eukaryotes92 and binds double-stranded DNA non-specifically forming nucleoprotein complexes (dodecamers) between DNA molecules.93 Since the LEM domain is also present in emerin and MAN1,17 it can be expected that all these proteins interact with chromatin-associated BAF,94 but this has not been experimentally tested yet.
Moreover, the N-terminal 50 residues of the LAP2 constant region were found by structural studies to contain a LEM-like motif that may bind DNA.39 In accordance with these findings an N-terminal 85-residue LAP2 fragment was found to associate with chromosomes in vitro.32
In addition to the N-terminal chromatin binding domains, common to all LAP2 proteins, in vitro studies revealed a DNA binding region in the LAP2β-specific C-terminus95, and a region in LAP2α's unique C-terminus was found to mediate chromosome association of LAP2α (the chromosomal binding partner is still unknown). Several studies have indicated that the interaction of the LAP2 isoforms with chromatin, mediated by the different domains, is regulated in a very complex and interdependent manner. For example, LAP2α's unique C-terminal chromatin binding domain was found both essential and sufficient for interaction of the protein with chromosomes during post-mitotic nuclear assembly, while the N-terminal LEM-like and LEM domains were not required at this stage.96 Furthermore, while LAP2 N-terminal fragments containing both the LEM-like and LEM motif did not interact with chromosomes when overexpressed in cells, and monomeric recombinant fragments did not bind to chromosomesin vitro,96 GST fusion proteins of the same fragments, which form oligomeres, interacted with chromosomes.96,32 This suggests that protein oligomerization, achieved by GST in the recombinant fragments or by C-terminal domains downstream of the LEM domain in full-length proteins, is required for tight interactions between the LEM domain and BAF and/or between the LEM-like motif and DNA. This hypothesis is further supported by recent in vitro binding studies showing that various Xenopus LAP2β-like isoforms, which are identical in their N-terminal part and contain the LEM domain, but differ slightly in their C-terminal regions, varied 9-fold in their affinities for BAF. Thus, the C-terminal unique regions in LAP2 isoforms may regulate the activity of the N-terminal LEM domain.89 Aside from their diverse interactions with DNA and BAF, LAP2β has also been identified by cross-linking experiments to associate with HA95, similar to LBR.87
It is not clear to what extent lamins and lamin binding proteins contribute to heterochromatin anchorage at the periphery. Considering the ˜ ten-fold larger abundance of lamins as compared to most lamin binding proteins, it can be assumed that lamins may have a major role in chromatin association. In line with this observation, it has been shown that the expression of a lamin mutant missing major parts of the rod domain caused relocalization of endogenous lamins and lamin-binding proteins to discrete patches at the nuclear envelope, not overlapping with patches of mutant protein. Despite the redistribution of lamin-binding proteins and pore complexes to patches of endogenous lamins, the position of chromatin was unchanged,97 suggesting that lamins rather than lamin-binding proteins anchor chromatin at the periphery. However, since overexpression of lamin mutants caused major changes in nuclear shape and arrested cell growth, these effects may have been caused by the unphysiological conditions.
The large diversity of interactions of different lamin-binding proteins with DNA and with different chromosomal proteins argues for an important role of lamin-binding proteins in chromatin organization and anchorage at the NE. These interactions might be important for a “more specialized”, cell stage-specific regulation of the chromatin-NE link during cell differentiation and/or cell cycle progression.
Two recently described nuclear pore complex (NPC)-associated proteins might also link the peripheral lamina to the internal nucleoskeleton and mediate chromatin anchorage and organization. Tpr (translocated promoter region) is a constitutive component of filaments extending from the nuclear pore basket structure 100–350 nm into the nucleus98 in extrachromosomal channel networks.99 Apart from being involved in mRNA transport,100,101 yeast Tpr homologues Mlp1 and Mlp2 have been shown to be involved in transcriptionally repressing telomeric genes by tethering telomere-binding factor yKu70 to the perinuclear region.102 The second candidate for a NPC-associated matrix protein is Nup153, which is a constituent of the nucleoplasmic pore basket103-105 and has been implicated in nuclear import and export.106-109 Highly sophisticated FRAP analysis and life cell imaging nicely showed that the nuclear lamina and NPC form a stable elastic network responsible for positioning NPCs in the membrane.110 Since Nup153 interacts directly with lamin LIII in Xenopus oocytes,111 and NPCs assembled in the absence of Nup153 lack anchorage within the NE,112 Nup153 may be important for linking the NPC to the lamina. Strikingly however, Nup153 fluorescence recovered much faster than those of other NPC proteins,110 indicating that Nup153 undergoes a rapid exchange between intranuclear and NPC-associated pools. In yeast, overexpression of Nup153 caused the formation of intranuclear membrane lamellae structures,113 suggesting that Nup153 might have additional nuclear binding partners. Zinc-finger motifs in Nup153 may mediate DNA interaction114 and early association of Nup153 with chromosomes after mitosis in a membrane-independent manner110,115,116also suggests that the protein may interact with chromatin.
Potential Functions of Lamina Proteins in Interphase
The molecular and cellular functions of lamins and lamin complexes remain unclear, although functional disruption of lamins in Drosophila117 and C. elegans118 revealed that they are essential for viability. In mice targeted disruption of A-type lamins caused muscular dystrophy, loss of adipose tissue, and early death,47 while mutations in the human lamin A gene were linked to inherited forms of muscular and lipodystrophies.41,119-121
Similar to cytoplasmic intermediate filament networks, the nuclear lamina has been suggested to serve as the structural backbone for the nucleus defining nuclear shape.2,11,119,120 Consistent with this function, nuclei assembled in vitro under lamin-depleted conditions were rather fragile122,123 and nuclei of lamin A knockout mice showed a irregular shape.47
The complex interactions of lamins and lamin-binding proteins with DNA and with chromatin-associated proteins (histones, HP1, HA95, and BAF) at the nuclear periphery (lamina including membrane proteins) and in the nuclear interior (A-type lamins and LAP2α) suggest functions of these proteins in higher order chromatin organization by providing specific chromatin docking sites at the NE and by structurally organizing chromatin fibers in the 3-dimensional nuclear space. Since higher order chromatin organization is ultimately linked to control of gene expression, lamina proteins might also be involved in this process. In line with this hypothesis, highly silenced human chromosome 18 occupies more peripheral territories in the nucleus as compared to highly active chromosome 19.124 Furthermore, the lamina protein LBR is found in a complex with HP1 (see above), a protein involved in position effect variegation and control of gene expression.81,82
In addition, components of the NE may directly influence transcription by interacting with transcription factors and/or chromatin remodeling complexes. A novel integral membrane protein of the inner membrane (Ring Finger Binding protein, RFBP), which resembles a type IV phospholipid pump, has recently been identified125 and has been shown to directly interact with RUSH proteins, SWI/SNF transcription factors that model chromatin. Thus, association of chromatin with the NE may regulate transcriptional access directly119. Furthermore, several findings have indicated a direct interaction of lamina proteins with E2F transcriptional complexes, which regulate G1-S phase progression in cell cycle by activating transcription of S-phase specific genes.126 The membrane-bound LAP2β was found by yeast two-hybrid analysis to bind mouse germ cell less (mGCL),127 which in turn interacts with E2F-associated DP and regulates the cell cycle.128 As overexpressed LAP2β reduced E2F-dependent reporter activity,127 it is likely that LAP2β might negatively regulate E2F activity by tethering the transcription complex to the nuclear periphery, a mechanism known also for other transcription factors.2 In addition, lamins A/C associate directly with the hypophosphorylated, active form of retinoblastoma protein (pRb),129 which binds E2F and represses transcription of S-phase-specific genes.130,131 LAP2α, which has been identified as a direct binding partner of A-type lamins34 might be another functional component of such a complex.
Lamina proteins might also be involved in DNA replication. Nuclei assembled in the absence of lamin B3 in Xenopus in vitro nuclear assembly reactions were not able to replicate their DNA,123,132 but addition of lamin B3 partially restored the phenotype.133 Similarly, lamin mutants causing nuclear lamina disassembly were shown to inhibit DNA replication,134-136 and lamin mutants causing a dramatic reorganization of the lamina and lobulated nuclei interfered with DNA replication and cell growth.97 Interestingly, ectopic expression of lamin-binding LAP2β fragments in mammalian cells inhibited progression into S-phase,137 while LAP2β mutants added to Xenopus in vitro nuclear assembly reactions influenced DNA replication positively.30 Thus LAP2β might also be involved in DNA-replication either directly or indirectly by affecting lamina assembly.
Lamina proteins might also be involved in controlling apoptosis. In C. elegans, for instance, CED-4, a cell death activator, is translocated from mitochondria to the nuclear envelope before caspase activation,138 suggesting that the lamina provides an attachment site for the apoptotic signaling machinery.2 Lamins, LAP2α and LAP2β are early targets of apoptosis139-141 and expression of uncleavable lamin mutants was shown to delay apoptosis for several hours.141 Furthermore, inhibition of lamin B assembly at the nuclear envelope upon preventing its postmitotic dephosphorylation induced apoptosis in human cells,142 suggesting that mislocalized lamins actively trigger apoptosis.143
Interestingly, the Drosophila lamin Dmo was recently found to be required for a cytoplasmic function in polarized cells, the outgrowth of cytoplasmic extensions from terminal cells of the tracheal system.144 The molecular mechanisms however remain obscure.
These diverse functions of lamina/matrix proteins may explain how mutations in lamina proteins cause different inheritable human diseases affecting heart and skeletal muscle as well as adipose tissue (laminopathies).41,119,121 It is conceivable that a disturbance of any of the above-described functions of lamins and lamin-binding proteins can contribute to the disease to different degrees. Mutations in emerin and lamin A do not only affect these two proteins but may have significant impact on other proteins tightly linked to lamin A structures. Thus, elucidating the function of any potential lamin A binding partner may provide important clues as to the molecular mechanisms of the disease. Therefore, in view of the recently reported interaction of LAP2α with the C-terminal region of A-type lamins34 containing many lipodystrophy and EDMD-specific mutations,145 it is intriguing to speculate that these mutations may effect LAP2α-lamin A interactions and interfere with LAP2α/lamin A functions. As such mutations would predominantly affect structures in the nuclear interior, the disease phenotype would be different from those caused by mutations in emerin or in lamin A, affecting mostly lamin A-emerin interactions and functions at the nuclear envelope.
Dynamics and Functions of Lamina-Chromatin Interactions During Mitosis
Multicellular eukaryotes reversibly disassemble the nuclear lamina, NPCs and the nucleoskeleton during mitosis, and the nuclear membrane merges into the endoplasmic reticulum.146 This process is driven by phosphorylation of lamins, LBR, LAP1 and peripheral as well as intranuclear LAP2 proteins mostly involving mitotic cyclin-dependent kinases (cdk), although other kinases may also play a role.10,31,66Mitotic A-type lamins are distributed in the cytoplasm probably as dimers or tetramers, while B-type lamins remain associated with membranes due to their C-terminal farnesyl modification, and probably also due to their interaction with LBR.21 LAP2β6 and LAP2α33 dissociate from lamins and chromosomes in a mitotic phosphorylation-dependent manner. There are reported discrepancies depending on the cell systems used as to whether nuclear membrane proteins (LAP2β, LBR and emerin) and ER proteins segregate into distinct membrane structures during mitosis or whether both disperse throughout the endoplasmic reticulum.147
Nuclear reassembly requires phosphatase activity and, at least for B-type lamins, has been shown to involve phosphatase PP1.148 PP1 is targeted to the NE by the membrane-integrated A-kinase anchoring protein AKAP149, and this process was shown to correlate with the assembly of B-type lamins.149 Inhibition of PP1 association with AKAP149 by a peptide containing the PP1 binding domain of AKAP149 resulted in lack of assembly of B-type lamins and apoptosis.142 Interestingly, however, assembly of A-type lamin was not effected by the peptide in HeLa cells, supporting other studies which show different pathways of assembly of A- and B-type lamins after mitosis.34,58
Numerous studies have shown that the assembly of the NE and nuclear structure after cell division requires targeting and assembly of lamins and lamin binding proteins to chromosomes in a temporally and spatially regulated manner (Fig. 2). LAP2α appears to be the first protein among the lamina components to associate with chromosomes in anaphase, clearly before LAP2β-containing membranes accumulate and enclose the decondensing chromosomes.96 Other membrane proteins, including LBR and emerin, accumulate at the chromosomal surface at the same time as LAP2β.115,116,150,151Interestingly, initial association of LBR with chromosomes was shown to occur primarily at the peripheral chromosomal regions115, while LAP2β, emerin and lamins were enriched at more central regions of chromosomes closest to the spindle poles and the midbody.6,58,115,151,152This suggested that association of LBR and LAP2β with chromosomes involves different mechanisms. Although a subfraction of B-type lamins might be targeted to the chromosomal surfaces by binding to LAP2β and or LBR and or histones, the bulk of lamin B assembly occurred after accumulation of the membrane proteins at the chromosomal surface.6,58 There are clear differences in the assembly of B-type and A-type lamins. While lamin B1 assembled around chromosomes at anaphase-telophase transition following accumulation of membranes, A-type lamins were targeted to intranuclear sites much later when an intact continuous nuclear envelope had already formed.58 Lamin A translocation to the nuclear interior might involve interaction with LAP2α34 (see below).
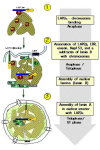
Figure 2
Sequence of protein targeting to chromosomal surfaces during nuclear reassembly following chromatid separation, and responsible interactions. For details see text.
More recently, the dynamics of the NPC-associated proteins Nup153 and Tpr during mitosis have been tested. Interestingly, Nup153, but not Tpr, was recruited to chromosomes at the same time as LAP2β and LBR,110,115,116 before accumulation of lamin B.58 As this interaction is membrane-independent, it seems to be the first step of NPC assembly.
The mechanisms regulating the sequential association of lamina proteins with chromatin are not very well understood. It is particularly intriguing that LAP2α assembles prior to LAP2β, although both proteins contain the LEM and LEM-like motifs in their N-terminal constant regions, known to bind BAF and/or DNA (see above). Several recent observations might help to explain this phenomenon. It was shown that the association of LAP2α with chromosomes at early stages of assembly requires the a-specific C-terminal domain, which is absent in LAP2β.96 The N-terminal BAF-binding domain, present in both LAP2α and b, did not interact with BAF at this stage of nuclear assembly, probably due to post-translational modification of LAP2 and/or BAF or due to an inhibitory effect of the C-terminal regions of LAP2.89 Therefore, we favor the following model for the initial stages of assembly (Fig. 2). In phase 1 LAP2α associates with chromosomes via its C-terminus, not involving the N-terminal LEM and LEM-like domains. This association triggers conformational changes on the chromosomal surface and/or induces post-translational modification of BAF or other chromosomal proteins and allows binding of the LEM domain to BAF in phase 2. This mediates cross-linking of chromosomal territories by chromosome bound LAP2α, and targeting of LAP2β, and emerin, and thus membrane structures to the chromosomal surface (phase 3).
Despite the fairly detailed analysis of kinetics of chromosome association of various lamina proteins, their specific roles in nuclear assembly is still not clear. The reported contributions of lamins to NE assembly has been controversial.153 While immunodepletion of lamins from in vitro Xenopus nuclear assembly extracts have indicated the formation of a NE in the absence of lamins,122,123 other studies using Drosophila, mammalian and Xenopus extracts showed that immunoadsorbtion of lamins inhibited NE assembly.154-156 As these different results were most likely caused by the different efficiencies in depleting and/or de-activating lamins by antibodies, Lopez-Soler et al have recently used a peptide containing the C-terminal domain of Xenopuslamin B3 in nuclear assembly reactions and found that the peptide inhibits nuclear lamin polymerization and also nuclear membrane assembly around chromatin.157 Thus Xenopus lamin is likely to have important functions in NE assembly.
LAP2β has originally been implicated in targeting membranes to chromosomes6, but this has not been demonstrated directly. Microinjection of LAP2β's nucleoplasmic domain into mitotic cells did not inhibit nuclear membrane targeting and assembly but affected nuclear growth during G1-S phase progression.137 The same fragment had similar effects, when added to Xenopus in vitro nuclear assembly reactions.30 However, all these studies were performed in a background of endogenous LAP2 proteins. Interestingly, an N-terminal LAP2β fragment containing the BAF-binding region was able to inhibit nuclear membrane binding in the Xenopus assembly system,30 but overexpression of the same fragment in mammalian cells had no detectable effect on cell cycle progression.96 This suggests that different molecular mechanisms of nuclear assembly may exist in early embryonic versus somatic cell divisions. Apart from LAP2β, LBR has also been suggested to be involved in membrane-chromosome interactions in in vitro binding studies using LBR-immunodepleted membrane fractions.158,159
The early accumulation of LAP2α around decondensing chromosomes suggested a function of the protein in providing a scaffold for chromatin organization. Considering the existence of at least two chromatin interaction domains in LAP2α, the protein is ideally suited to cross-link chromosomal regions and help to structurally organize chromatin in post-mitotic nuclei. Observations showing that overexpression of a C-terminal chromatin-binding fragment of LAP2α was toxic for the cells and that addition of the same fragment to mammalian in vitro nuclear assembly extracts inhibited NE formation96 (Vlcek, Korbei and Foisner, submitted), supported this hypothesis, but the mechanisms remain obscure.
Conclusions and Future Prospects
The recent discovery of novel interaction partners for lamins and lamina-associated proteins have changed our view of how these proteins function in the cell nucleus. While former studies revealed mainly structural function, novel findings point towards important functions also in controlling DNA replication, gene transcription and cell cycle progression in a more direct fashion. Nevertheless, I believe that this is just the tip of the iceberg, and more interactions of lamin and lamin-binding proteins, which may be regulated in a development-, differentiation- or cell cycle-specific manner, will be identified in the future. This will give us a much clearer picture of the specific involvement of lamina proteins in these processes and will also allow to completely unravel the molecular mechanisms behind the lamin-related human diseases (laminopathies).
Acknowledgements
Work in the author's laboratory was supported by grants from the Austrian Science Research Fund (FWF) No. P13374 to RF.
References
- 1.
- Gotzmann J, Foisner R. Lamins and lamin-binding proteins in functional chromatin organization. Crit Rev Eukaryot Gene Expr. 1999;9:257–265. [PubMed: 10651242]
- 2.
- Cohen M, Lee KK, Wilson KL, Gruenbaum Y. Transcriptional repression, apoptosis, human disease and the functional evolution of the nuclear lamina. Trends Biochem Sci. 2001;26:41–47. [PubMed: 11165516]
- 3.
- Foisner R. Inner nuclear membrane proteins and the nuclear lamina. J Cell Sci. 2001;114:3791–3792. [PubMed: 11719545]
- 4.
- Gerace L, Foisner R. Integral membrane proteins and dynamic organization of the nuclear envelope. Trends Cell Biol. 1994;4:127–131. [PubMed: 14731735]
- 5.
- Senior A, Gerace L. Integral membrane proteins specific to the inner nuclear membrane and associated with the nuclear lamina. J Cell Biol. 1988;107:2029–2036. [PMC free article: PMC2115672] [PubMed: 3058715]
- 6.
- Foisner R, Gerace L. Integral membrane proteins of the nuclear envelope interact with lamins and chromosomes, and binding is modulated by mitotic phosphorylation. Cell. 1993; 73:1267–1279. [PubMed: 8324822]
- 7.
- Nickerson J. Experimental observations of a nuclear matrix. J Cell Sci. 2001;114:463–474. [PubMed: 11171316]
- 8.
- Pederson T. Half a century of the “nuclear matrix” Mol Biol Cell. 2000;11:799–805. [PMC free article: PMC14811] [PubMed: 10712500]
- 9.
- Stuurman N, Heins S, Aebi U. Nuclear lamins: their structure, assembly, and interactions. J Struct Biol. 1998; 122:42–66. [PubMed: 9724605]
- 10.
- Moir RD, Spann TP, Goldman RD. The dynamic properties and possible functions of nuclear lamins. Int Rev Cytol. 1995:141–182. [PubMed: 8557486]
- 11.
- Hutchison CJ, Alvarez-Reyes M, Vaughan OA. Lamins in disease: why do ubiquitously expressed nuclear envelope proteins give rise to tissue-specific disease phenotypes? J Cell Sci. 2001;114:9–19. [PubMed: 11112685]
- 12.
- Mical TI, Monteiro MJ. The role of sequences unique to nuclear intermediate filaments in the targeting and assembly of human lamin B: evidence for lack of interaction of lamin B with its putative receptor. J Cell Sci. 1998; 111:3471–3485. [PubMed: 9811562]
- 13.
- Hennekes H, Nigg EA. The role of isoprenylation in membrane attachment of nuclear lamins. A single point mutation prevents proteolytic cleavage of the lamin A precursor and confers membrane binding properties. J Cell Sci. 1994; 107:1019–1029. [PubMed: 8056827]
- 14.
- Izumi M, Vaughan OA, Hutchison CJ, Gilbert DM. Head and/or CaaX domain deletions of lamin proteins disrupt preformed lamin A and C but not lamin B structure in mammalian cells. Mol Biol Cell. 2000;11:4323–4337. [PMC free article: PMC15075] [PubMed: 11102526]
- 15.
- Gerace L, Burke B. Functional organization of the nuclear envelope. Annu Rev Cell Biol. 1988;4:335–374. [PubMed: 2461721]
- 16.
- Worman HJ, Yuan J, Blobel G, Georgatos SD. A lamin B receptor in the nuclear envelope. Proc Natl Acad Sci U S A. 1988;85:8531–8534. [PMC free article: PMC282492] [PubMed: 2847165]
- 17.
- Lin F, Blake DL, Callebaut I, Skerjanc IS, Holmer L, McBurney MW. et al. MAN1, an inner nuclear membrane protein that shares the LEM domain with lamina-associated polypeptide 2 and emerin. J Biol Chem. 2000; 275:4840–4847. [PubMed: 10671519]
- 18.
- Manilal S, Nguyen TM, Sewry CA, Morris GE. The Emery-Dreifuss muscular dystrophy protein, emerin, is a nuclear membrane protein. Hum Mol Genet. 1996;5:801–808. [PubMed: 8776595]
- 19.
- Worman HJ, Evans CD, Blobel G. The lamin B receptor of the nuclear envelope inner membrane: a polytopic protein with eight potential transmembrane domains. J Cell Biol. 1990; 111:1535–1542. [PMC free article: PMC2116249] [PubMed: 2170422]
- 20.
- Simos G, Georgatos SD. The inner nuclear membrane protein p58 associates in vivo with a p58 kinase and the nuclear lamins. EMBO J. 1992;11:4027–4036. [PMC free article: PMC556913] [PubMed: 1327755]
- 21.
- Meier J, Georgatos SD. Type B lamins remain associated with the integral nuclear envelope protein p58 during mitosis: implications for nuclear reassembly. EMBO J. 1994;13:1888–1898. [PMC free article: PMC395030] [PubMed: 8168487]
- 22.
- Ye Q, Worman HJ. Primary structure analysis and lamin B and DNA binding of human LBR, an integral protein of the nuclear envelope inner membrane. J Biol Chem. 1994; 269:11306–11311. [PubMed: 8157662]
- 23.
- Holmer L, Pezhman A, Worman HJ. The human lamin B receptor/sterol reductase multigene family. Genomics. 1998; 54:469–476. [PubMed: 9878250]
- 24.
- Silve S, Dupuy PH, Ferrara P, Loison G. Human lamin B receptor exhibits sterol C14-reductase activity in Saccharomyces cerevisiae. Biochim Biophys Acta. 1998; 1392:233–244. [PubMed: 9630650]
- 25.
- Martin L, Crimaudo C, Gerace L. cDNA cloning and characterization of lamina-associated polypeptide 1C (LAP1C), an integral protein of the inner nuclear membrane. J Biol Chem. 1995;270:8822–8828. [PubMed: 7721789]
- 26.
- Powell L, Burke B. Internuclear exchange of an inner nuclear membrane protein (p55) in heterokaryons: in vivo evidence for the interaction of p55 with the nuclear lamina. J Cell Biol. 1990;111:2225–2234. [PMC free article: PMC2116408] [PubMed: 2277058]
- 27.
- Harris CA, Andryuk PJ, Cline S, Chan HK, Natarajan A, Siekierka JJ, Goldstein G. Three distinct human thymopoietins are derived from alternatively spliced mRNAs. Proc Natl Acad Sci U S A. 1994;91:6283–6287. [PMC free article: PMC44185] [PubMed: 7517549]
- 28.
- Berger R, Theodor L, Shoham J, Gokkel E, Brok-Simoni F, Avraham KB, Copeland NG, Jenkins NA, Rechavi G, Simon AJ. The characterization and localization of the mouse thymopoietin/lamina-associated polypeptide 2 gene and its alternatively spliced products. Genome Res. 1996;6:361–370. [PubMed: 8743987]
- 29.
- Lang C, Paulin-Levasseur M, Gajewski A, Alsheimer M, Benavente R, Krohne G. Molecular characterization and developmentally regulated expression of Xenopus lamina-associated polypeptide 2 (XLAP2). J Cell Sci. 1999; 112:749–759. [PubMed: 9973608]
- 30.
- Gant TM, Harris CA, Wilson KL. Roles of LAP2 proteins in nuclear assembly and DNA replication: Truncated LAP2β proteins alter lamina assembly, envelope formation, nuclear size, and DNA replication efficiency in Xenopus laevis extracts. J Cell Biol. 1999;144:1083–1096. [PMC free article: PMC2150574] [PubMed: 10087255]
- 31.
- Dechat T, Vlcek S, Foisner R. Review: Lamina-associated polypeptide 2 isoforms and related proteins in cell cycle-dependent nuclear structure dynamics. J Struct Biol. 2000;129:335–345. [PubMed: 10806084]
- 32.
- Furukawa K, Fritze CE, Gerace L. The major nuclear envelope targeting domain of LAP2 coincides with its lamin binding region but is distinct from its chromatin interaction domain. J Biol Chem. 1998;273:4213–4219. [PubMed: 9461618]
- 33.
- Dechat T, Gotzmann J, Stockinger A, Harris CA, Talle MA, Siekierka JJ, Foisner R. Detergent-salt resistance of LAP2α in interphase nuclei and phosphorylation-dependent association with chromosomes early in nuclear assembly implies functions in nuclear structure dynamics. EMBO J. 1998;17:4887–4902. [PMC free article: PMC1170818] [PubMed: 9707448]
- 34.
- Dechat T, Korbei B, Vaughan OA, Vlcek S, Hutchison CJ, Foisner R. Lamina-associated polypeptide 2alpha binds intranuclear A-type lamins. J Cell Sci. 2000;113 Pt 19:3473–3484. [PubMed: 10984438]
- 35.
- Theodor L, Shoham J, Berger R, Gokkel E, Trachtenbrot L, Simon AJ, Brok-Simon F, Nir U, Ilan E, Zevin-Sonkin D, Friedman E, Rechavi G. Ubiquitous expression of a cloned murine thymopoietin cDNA. Acta Haematol. 1997;97:153–163. [PubMed: 9066710]
- 36.
- Ishijima Y, Toda T, Matsushita H, Yoshida M, Kimura N. Expression of thymopoietin beta/lamina-associated polypeptide 2 (TP beta/LAP2) and its family proteins as revealed by specific antibody induced against recombinant human thymopoietin. Biochem Biophys Res Commun. 1996;226:431–438. [PubMed: 8806652]
- 37.
- Dreger M, Bengtsson L, Schoneberg T, Otto H, Hucho F. Nuclear envelope proteomics: Novel integral membrane proteins of the inner nuclear membrane. Proc Natl Acad Sci U S A. 2001; 98:11943–11948. [PMC free article: PMC59747] [PubMed: 11593002]
- 38.
- Laguri C, Gilquin B, Wolff N, Romi-Lebrun R, Courchay K, Callebaut I, Worman HJ, Zinn-Justin S. Structural characterization of the LEM motif common to three human inner nuclear membrane proteins. Structure (Camb). 2001;9:503–511. [PubMed: 11435115]
- 39.
- Cai M, Huang Y, Ghirlando R, Wilson KL, Craigie R, Clore GM. Solution structure of the constant region of nuclear envelope protein LAP2 reveals two LEM-domain structures: one binds BAF and the other binds DNA. EMBO J. 2001;20:4399–4407. [PMC free article: PMC125263] [PubMed: 11500367]
- 40.
- Ostlund C, Ellenberg J, Hallberg E, Lippincott-Schwartz J, Worman HJ. Intracellular trafficking of emerin, the Emery-Dreifuss muscular dystrophy protein. J Cell Sci. 1999; 112:1709–1719. [PubMed: 10318763]
- 41.
- Morris GE, Manilal S. Heart to heart: from nuclear proteins to Emery-Dreifuss muscular dystrophy. Hum Mol Genet. 1999;8:1847–1851. [PubMed: 10469836]
- 42.
- Nagano A, Koga R, Ogawa M, Kurano Y, Kawada J, Okada R, Hayashi YK, Tsukuhara T, Arahata K. Emerin deficiency at the nuclear membrane in patients with Emery-Dreyfuss muscular dystrophy. Nature Genet. 1996;12:254–259. [PubMed: 8589715]
- 43.
- Sakaki M, Koike H, Takahashi N, Sasagawa N, Tomioka S, Arahata K, Ishiura S. Interaction between emerin and nuclear lamins. J Biochem (Tokyo). 2001;129:321–327. [PubMed: 11173535]
- 44.
- Fairley EA, Kendrick-Jones J, Ellis JA. The Emery-Dreifuss muscular dystrophy phenotype arises from aberrant targeting and binding of emerin at the inner nuclear membrane. J Cell Sci. 1999;112:2571–2582. [PubMed: 10393813]
- 45.
- Clements L, Manilal S, Love DR, Morris GE. Direct interaction between emerin and lamin A. Biochem Biophys Res Commun. 2000;267:709–714. [PubMed: 10673356]
- 46.
- Vaughan OA, Alvarez-Reyes M, Bridger JM, Broers LV, Ramaekers FCS, Wehnert M, Morris G, Whitfield WGF, Hutchison CJ. Both emerin and lamin C depend on lamin A for localization at the nuclear envelope. J Cell Sci. 2001; 114:2577–2590. [PubMed: 11683386]
- 47.
- Sullivan T, Escalante-Alcalde D, Bhatt H, Anver M, Bhat N, Nagashima K, Stewart CL, Burke B. Loss of A-type lamin expression compromises nuclear envelope integrity leading to muscular dystrophy. J Cell Biol. 1999;147:913–920. [PMC free article: PMC2169344] [PubMed: 10579712]
- 48.
- Paulin-Levasseur M, Blake DL, Julien M, Rouleau L. The MAN antigens are non-lamin constituents of the nuclear lamina in vertebrate cells. Chromosoma. 1996;104:367–379. [PubMed: 8575249]
- 49.
- Rolls MM, Stein PA, Taylor SS, Ha E, McKeon F, Rapoport TA. A visual screen of a GFP-fusion library identifies a new type of nuclear envelope membrane protein. J Cell Biol. 1999; 146:29–44. [PMC free article: PMC2199743] [PubMed: 10402458]
- 50.
- Malone CJ, Fixsen WD, Horvitz HR, Han M. UNC-84 localizes to the nuclear envelope and is required for nuclear migration and anchoring during C. elegans development. Development. 1999;126:3171–3181. [PubMed: 10375507]
- 51.
- Padan R, Nainudel-Epszteyn S, Goitein R, Fainsod A, Gruenbaum Y. Isolation and characterization of the Drosophila nuclear envelope otefin cDNA. J Biol Chem. 1990;265:7808–7813. [PubMed: 2186029]
- 52.
- Goldberg M, Lu H, Stuurman N, Ashery-Padan R, Weiss AM, Yu J, Bhattacharyya D, Fisher PA, Gruenbaum Y, Wolfner MF. Interactions among Drosophila nuclear envelope proteins lamin, otefin, and YA. Mol Cell Biol. 1998; 18:4315–4323. [PMC free article: PMC109015] [PubMed: 9632815]
- 53.
- Fricker M, Hollinshead M, White N, Vaux D. Interphase nuclei of many mammalian cell types contain deep, dynamic, tubular membrane-bound invaginations of the nuclear envelope. J Cell Biol. 1997;136:531–544. [PMC free article: PMC2134289] [PubMed: 9024685]
- 54.
- Jagatheesan G, Thanumalayan S, Muralikrishna B, Rangaraj N, Karande AA, Parnaik VK. Colocalization of intranuclear lamin foci with RNA splicing factors. J Cell Sci. 1999;112:4651–4661. [PubMed: 10574713]
- 55.
- Hozak P, Sasseville AM, Raymond Y, Cook PR. Lamin proteins form an internal nucleoskeleton as well as a peripheral lamina in human cells. J Cell Sci. 1995;108:635–644. [PubMed: 7769007]
- 56.
- Neri LM, Raymond Y, Giordano A, Capitani S, Martelli AM. Lamin A is part of the internal nucleoskeleton of human erythroleukemia cells. J Cell Physiol. 1999;178:284–295. [PubMed: 9989774]
- 57.
- Broers JL, Machiels BM, van Eys GJ, Kuijpers HJ, Manders EM, van Driel R, Ramaekers FC. Dynamics of the nuclear lamina as monitored by GFP-tagged A-type lamins. J Cell Sci. 1999;112:3463–3475. [PubMed: 10504295]
- 58.
- Moir RD, Yoon M, Khuon S, Goldman RD. Nuclear lamins A and B1: different pathways of assembly during nuclear envelope formation in living cells. J Cell Biol. 2000; 151:1155–1168. [PMC free article: PMC2190592] [PubMed: 11121432]
- 59.
- Moir RD, Montag-Lowy M, Goldman RD. Dynamic properties of nuclear lamins: lamin B is associated with sites of DNA replication. J Cell Biol. 1994;125:1201–1212. [PMC free article: PMC2290916] [PubMed: 7911470]
- 60.
- Bridger JM, Kill IR, O'Farrell M, Hutchison CJ. Internal lamin structures within G1 nuclei of human dermal fibroblasts. J Cell Sci. 1993;104:297–306. [PubMed: 8505362]
- 61.
- Goldman AE, Moir RD, Montag-Lowy M, Stewart M, Goldman RD. Pathway of incorporation of microinjected lamin A into the nuclear envelope. J Cell Biol. 1992;119:725–735. [PMC free article: PMC2289687] [PubMed: 1429833]
- 62.
- Pugh GE, Coates PJ, Lane EB, Raymond Y, Quinlan RA. Distinct nuclear assembly pathways for lamins A and C lead to their increase during quiescence in Swiss 3T3 cells. J Cell Sci. 1997;110:2483–2493. [PubMed: 9410886]
- 63.
- Lutz RJ, Trujillo MA, Denham KS, Wenger L, Sinensky M. Nucleoplasmic localization of prelamin A: implications for prenylation- dependent lamin A assembly into the nuclear lamina [published erratum appears in Proc Natl Acad Sci U S A 1992 Jun 15;89(12):5699] Proc Natl Acad Sci U S A. 1992; 89:3000–3004. [PMC free article: PMC48791] [PubMed: 1557405]
- 64.
- Sasseville AM, Raymond Y. Lamin A precursor is localized to intranuclear foci. J Cell Sci. 1995;108:273–285. [PubMed: 7738105]
- 65.
- Barton RM, Worman HJ. Prenylated prelamin A interacts with Narf, a novel nuclear protein. J Biol Chem. 1999; 274:30008–30018. [PubMed: 10514485]
- 66.
- Foisner R. Dynamic organisation of intermediate filaments and associated proteins during the cell cycle. Bioessays. 1997;19:297–305. [PubMed: 9136627]
- 67.
- Hari KL, Cook KR, Karpen GH. The Drosophila Su(var)2-10 locus regulates chromosome structure and function and encodes a member of the PIAS protein family. Genes Dev. 2001;15:1334–1348. [PMC free article: PMC312712] [PubMed: 11390354]
- 68.
- Li G, Sudlow G, Belmont AS. Interphase cell cycle dynamics of a late-replicating, heterochromatic homogeneously staining region: precise choreography of condensation/decondensation and nuclear positioning. J Cell Biol. 1998;140:975–989. [PMC free article: PMC2132695] [PubMed: 9490713]
- 69.
- Belmont AS, Zhai Y, Thilenius A. Lamin B distribution and association with peripheral chromatin revealed by optical sectioning and electron microscopy tomography. J Cell Biol. 1993;123:1671–1685. [PMC free article: PMC2290888] [PubMed: 8276889]
- 70.
- Luderus ME, de Graaf A, Mattia E, den Blaauwen JL, Grande MA, de Jong L, van Driel R. Binding of matrix attachment regions to lamin B1. Cell. 1992;70:949–959. [PubMed: 1525831]
- 71.
- Zhao K, Harel A, Stuurman N, Guedalia D, Gruenbaum Y. Binding of matrix attachment regions to nuclear lamin is mediated by the rod domain and depends on the lamin polymerization state. FEBS Lett. 1996;380:161–164. [PubMed: 8603728]
- 72.
- Shoeman RL, Traub P. The in vitro DNA-binding properties of purified nuclear lamin proteins and vimentin. J Biol Chem. 1990;265:9055–9061. [PubMed: 2345165]
- 73.
- Baricheva EA, Berrios M, Bogachev SS, Borisevich IV, Lapik ER, Sharakhov IV, Stuurman N, Fisher PA. DNA from Drosophila melanogaster beta-heterochromatin binds specifically to nuclear lamins in vitro and the nuclear envelope in situ. Gene. 1996;171:171–176. [PubMed: 8666268]
- 74.
- Rzepecki R, Bogachev SS, Kokoza E, Stuurman N, Fisher PA. In vivo association of lamins with nucleic acids in Drosophila melanogaster. J Cell Sci. 1998;111:121–129. [PubMed: 9394018]
- 75.
- Glass CA, Glass JR, Taniura H, Hasel KW, Blevitt JM, Gerace L. The alpha-helical rod domain of human lamins A and C contains a chromatin binding site. EMBO J. 1993;12:4413–4424. [PMC free article: PMC413739] [PubMed: 8223451]
- 76.
- Taniura H, Glass C, Gerace L. A chromatin binding site in the tail domain of nuclear lamins that interacts with core histones. J Cell Biol. 1995;131:33–44. [PMC free article: PMC2120604] [PubMed: 7559784]
- 77.
- Goldberg M, Harel A, Brandeis M, Rechsteiner T, Richmond TJ, Weiss AM, Gruenbaum Y. The tail domain of lamin Dm0 binds histones H2A and H2B. Proc Natl Acad Sci U S A. 1999; 96:2852–2857. [PMC free article: PMC15858] [PubMed: 10077600]
- 78.
- Duband-Goulet I, Courvalin JC. Inner nuclear membrane protein LBR preferentially interacts with DNA secondary structures and nucleosomal linker. Biochemistry. 2000; 39:6483–6488. [PubMed: 10828963]
- 79.
- Ye Q, Callebaut I, Pezhman A, Courvalin JC, Worman HJ. Domain-specific interactions of human HP1-type chromodomain proteins and inner nuclear membrane protein LBR. J Biol Chem. 1997;272:14983–14989. [PubMed: 9169472]
- 80.
- Ye Q, Worman HJ. Interaction between an integral protein of the nuclear envelope inner membrane and human chromodomain proteins homologous to Drosophila HP1. J Biol Chem. 1996;271:14653–14656. [PubMed: 8663349]
- 81.
- Cavalli G, Paro R. Chromo-domain proteins: linking chromatin structure to epigenetic regulation. Curr Opin Cell Biol. 1998;10:354–360. [PubMed: 9640536]
- 82.
- Jones DO, Cowell IG, Singh PB. Mammalian chromodomain proteins: their role in genome organisation and expression. Bioessays. 2000;22:124–137. [PubMed: 10655032]
- 83.
- Kourmouli N, Dialynas G, Petraki C, Pyrpasopoulou A, Singh PB, Georgatos SD, Theodoropoulos PA. Binding of heterochromatin protein 1 to the nuclear envelope is regulated by a soluble form of tubulin. J Biol Chem. 2001; 276:13007–13014. [PubMed: 11278332]
- 84.
- Polioudaki H, Kourmouli N, Drosou V, Bakou A, Theodoropoulos PA, Singh PB, Giannakouros T, Georgatos SD. Histones H3/H4 form a tight complex with the inner nuclear membrane protein LBR and heterochromatin protein 1. EMBO Rep. 2001;2:920–925. [PMC free article: PMC1084077] [PubMed: 11571267]
- 85.
- Bannister AJ, Zegerman P, Partridge JF, Miska EA, Thomas JO, Allshire RC, Kouzarides T. Selective recognition of methylated lysine 9 on histone H3 by the HP1 chromo domain. Nature. 2001;410:120–124. [PubMed: 11242054]
- 86.
- Lachner M, O'Carroll D, Rea S, Mechtler K, Jenuwein T. Methylation of histone H3 lysine 9 creates a binding site for HP1 proteins. Nature. 2001;410:116–120. [PubMed: 11242053]
- 87.
- Martins SB, Eide T, Steen RL, Jahnsen T, Skalhegg BS, Collas P. HA95 is a protein of the chromatin and nuclear matrix regulating nuclear envelope dynamics. J Cell Sci. 2000; 113 Pt 21:3703–3713. [PubMed: 11034899]
- 88.
- Furukawa K. LAP2 binding protein 1 (L2BP1/BAF) is a candidate mediator of LAP2-chromatin interaction. J Cell Sci. 1999;112:2485–2492. [PubMed: 10393804]
- 89.
- Shumaker DK, Lee KK, Tanhehco YC, Craigie R, Wilson KL. LAP2 binds to BAF-DNA complexes: requirement for the LEM domain and modulation by variable regions. EMBO J. 2001; 20:1754–1764. [PMC free article: PMC145505] [PubMed: 11285238]
- 90.
- Chen H, Engelman A. The barrier-to-autointegration protein is a host factor for HIV type 1 integration. Proc Natl Acad Sci U S A. 1998;95:15270–15274. [PMC free article: PMC28032] [PubMed: 9860958]
- 91.
- Lee MS, Craigie R. A previously unidentified host protein protects retroviral DNA from autointegration. Proc Natl Acad Sci USA. 1998;95:1528–1533. [PMC free article: PMC19075] [PubMed: 9465049]
- 92.
- Cai M, Huang Y, Zheng R, Wei SQ, Ghirlando R, Lee MS, Craigie R, Gronenborn AM, Clore GM. Solution structure of the cellular factor BAF responsible for protecting retroviral DNA from autointegration. Nat Struct Biol. 1998;5:903–909. [PubMed: 9783751]
- 93.
- Zheng R, Ghirlando R, Lee MS, Mizuuchi K, Krause M, Craigie R. Barrier-to-autointegration factor (BAF) bridges DNA in a discrete, higher-order nucleoprotein complex. Proc Natl Acad Sci U S A. 2000;97:8997–9002. [PMC free article: PMC16810] [PubMed: 10908652]
- 94.
- Wolff N, Gilquin B, Courchay K, Callebaut I, Worman HJ, Zinn-Justin S. Structural analysis of emerin, an inner nuclear membrane protein mutated in X-linked Emery-Dreifuss muscular dystrophy. FEBS Lett. 2001;501:171–176. [PubMed: 11470279]
- 95.
- Furukawa K, Glass C, Kondo T. Characterization of the chromatin binding activity of lamina-associated polypeptide (LAP) 2. Biochem Biophys Res Commun. 1997;238:240–246. [PubMed: 9299486]
- 96.
- Vlcek S, Just H, Dechat T, Foisner R. Functional diversity of LAP2α and LAP2β in postmitotic chromosome association is caused by an alpha-specific nuclear targeting domain. EMBO J. 1999;18:6370–6384. [PMC free article: PMC1171700] [PubMed: 10562549]
- 97.
- Schirmer EC, Guan T, Gerace L. Involvement of the lamin rod domain in heterotypic lamin interactions important for nuclear organization. J Cell Biol. 2001;153:479–489. [PMC free article: PMC2190570] [PubMed: 11331300]
- 98.
- Cordes VC, Reidenbach S, Rackwitz HR, Franke WW. Identification of protein p270/Tpr as a constitutive component of the nuclear pore complex-attached intranuclear filaments. J Cell Biol. 1997;136:515–529. [PMC free article: PMC2134304] [PubMed: 9024684]
- 99.
- Zimowska G, Aris JP, Paddy MR. A Drosophila Tpr protein homolog is localized both in the extrachromosomal channel network and to nuclear pore complexes. J Cell Sci. 1997;110:927–944. [PubMed: 9152019]
- 100.
- Bangs P, Burke B, Powers C, Craig R, Purohit A, Doxsey S. Functional analysis of Tpr: identification of nuclear pore complex association and nuclear localization domains and a role in mRNA export. J Cell Biol. 1998;143:1801–1812. [PMC free article: PMC2175216] [PubMed: 9864356]
- 101.
- Strambio-de-Castillia C, Blobel G, Rout MP. Proteins connecting the nuclear pore complex with the nuclear interior. J Cell Biol. 1999;144:839–855. [PMC free article: PMC2148185] [PubMed: 10085285]
- 102.
- Galy V, Olivo-Marin JC, Scherthan H, Doye V, Rascalou N, Nehrbass U. Nuclear pore complexes in the organization of silent telomeric chromatin [see comments] Nature. 2000; 403:108–112. [PubMed: 10638763]
- 103.
- Stoffler D, Fahrenkrog B, Aebi U. The nuclear pore complex: from molecular architecture to functional dynamics. Curr Opin Cell Biol. 1999;11:391–401. [PubMed: 10395558]
- 104.
- Allen TD, Cronshaw JM, Bagley S, Kiseleva E, Goldberg MW. The nuclear pore complex: mediator of translocation between nucleus and cytoplasm. J Cell Sci. 2000; 113:1651–1659. [PubMed: 10769196]
- 105.
- Cordes VC, Reidenbach S, Kohler A, Stuurman N, van Driel R, Franke WW. Intranuclear filaments containing a nuclear pore complex protein. J Cell Biol. 1993;123:1333–1344. [PMC free article: PMC2290899] [PubMed: 8253834]
- 106.
- Shah S, Forbes DJ. Separate nuclear import pathways converge on the nucleoporin Nup153 and can be dissected with dominant-negative inhibitors. Curr Biol. 1998;8:1376–1386. [PubMed: 9889100]
- 107.
- Bastos R, Lin A, Enarson M, Burke B. Targeting and function in mRNA export of nuclear pore complex protein Nup 153. J Cell Biol. 1996;134:1141–1156. [PMC free article: PMC2120979] [PubMed: 8794857]
- 108.
- Moroianu J, Blobel G, Radu A. RanGTP-mediated nuclear export of karyopherin alpha involves its interaction with the nucleoporin Nup 153. Proc Natl Acad Sci U S A. 1997; 94:9699–9704. [PMC free article: PMC23253] [PubMed: 9275187]
- 109.
- Nakielny S, Shaikh S, Burke B, Dreyfuss G. Nup153 is an M9-containing mobile nucleoporin with a novel Ran-binding domain. EMBO J. 1999;18:1982–1995. [PMC free article: PMC1171283] [PubMed: 10202161]
- 110.
- Daigle N, Beaudouin J, Hartnell L, Imreh G, Hallberg E, Lippincott-Schwartz J, Ellenberg J. Nuclear pore complexes form immobile networks and have a very low turnover in live mammalian cells. J Cell Biol. 2001;154:71–84. [PMC free article: PMC2196857] [PubMed: 11448991]
- 111.
- Smythe C, Jenkins HE, Hutchison CJ. Incorporation of the nuclear pore basket protein Nup153 into nuclear pore structures is dependent upon lamina assembly: evidence from cell-free extracts of Xenopus eggs. EMBO J. 2000; 19:3918–3931. [PMC free article: PMC306609] [PubMed: 10921874]
- 112.
- Walther TC, Fornerod M, Pickersgill H, Goldberg M, Allen TD, Mattaj IW. The nucleoporin Nup153 is required for nuclear pore basket formation, nuclear pore complex anchoring and import of a subset of nuclear proteins. EMBO J. 2001; 20:5703–5714. [PMC free article: PMC125666] [PubMed: 11598013]
- 113.
- Marelli M, Lusk CP, Chan H, Aitchison JD, Wozniak RW. A link between the synthesis of nucleoporins and the biogenesis of the nuclear envelope. J Cell Biol. 2001; 153:709–724. [PMC free article: PMC2192385] [PubMed: 11352933]
- 114.
- Sukegawa J, Blobel G. A nuclear pore complex protein that contains zinc finger motifs, binds DNA, and faces the nucleoplasm. Cell. 1993;72:29–38. [PubMed: 8422679]
- 115.
- Haraguchi T, Koujin T, Hayakawa T, Kaneda T, Tsutsumi C, Imamoto N, Akazawa C, Sukegawa J, Yoneda Y, Hiraoka Y. Live fluorescence imaging reveals early recruitment of emerin, LBR, RanBP2, and Nup153 to reforming functional nuclear envelopes. J Cell Sci. 2000;113:779–794. [PubMed: 10671368]
- 116.
- Bodoor K, Shaikh S, Salina D, Raharjo WH, Bastos R, Lohka M, Burke B. Sequential recruitment of NPC proteins to the nuclear periphery at the end of mitosis. J Cell Sci. 1999; 112:2253–2264. [PubMed: 10362555]
- 117.
- Lenz-Bohme B, Wismar J, Fuchs S, Reifegerste R, Buchner E, Betz H. et al. Insertional mutation of the Drosophila nuclear lamin Dm0 gene results in defective nuclear envelopes, clustering of nuclear pore complexes, and accumulation of annulate lamellae. J Cell Biol. 1997; 137:1001–1016. [PMC free article: PMC2136230] [PubMed: 9166402]
- 118.
- Liu J, Ben-Shahar TR, Riemer D, Treinin M, Spann P, Weber K, Fire A, Gruenbaum Y. Essential roles for Caenorhabditis elegans lamin gene in nuclear organization, cell cycle progression, and spatial organization of nuclear pore complexes. Mol Biol Cell. 2000;11:3937–3947. [PMC free article: PMC15048] [PubMed: 11071918]
- 119.
- Wilson KL, Zastrow MS, Lee KK. Lamins and disease: insights into nuclear infrastructure. Cell. 2001;104:647–650. [PubMed: 11257219]
- 120.
- Wilson KL. The nuclear envelope, muscular dystrophy and gene expression. Trends Cell Biol. 2000;10:125–129. [PubMed: 10740265]
- 121.
- Nagano A, Arahata K. Nuclear envelope proteins and associated diseases. Curr Opin Neurol. 2000;13:533–539. [PubMed: 11073359]
- 122.
- Meier J, Campbell KH, Ford CC, Stick R, Hutchison CJ. The role of lamin LIII in nuclear assembly and DNA replication, in cell- free extracts of Xenopus eggs. J Cell Sci. 1991;98:271–279. [PubMed: 2055960]
- 123.
- Newport JW, Wilson KL, Dunphy WG. A lamin-independent pathway for nuclear envelope assembly. J Cell Biol. 1990;111:2247–2259. [PMC free article: PMC2116431] [PubMed: 2277059]
- 124.
- Croft JA, Bridger JM, Boyle S, Perry P, Teague P, Bickmore WA. Differences in the localization and morphology of chromosomes in the human nucleus. J Cell Biol. 1999; 145:1119–1131. [PMC free article: PMC2133153] [PubMed: 10366586]
- 125.
- Mansharamani M, Hewetson A, Chilton BS. Cloning and characterization of an atypical Type IV P-type ATPase that binds to the RING motif of RUSH transcription factors. J Biol Chem. 2001;276:3641–3649. [PubMed: 11058586]
- 126.
- Lavia P, Jansen-Durr P. E2F target genes and cell-cycle checkpoint control. BioEssays. 1999;21:221–230. [PubMed: 10333731]
- 127.
- Nili E, Cojocaru GS, Kalma Y, Ginsberg D, Copeland NG, Gilbert DJ, Jenkins NA, Berger R, Shaklai S, Amariglio N, Brok-Simoni F, Simon AJ, Rechavi G. Nuclear membrane protein LAP2β mediates transcriptional repression alone and together with its binding partner GCL (germ-cell-less). J Cell Sci. 2001;114:3297–3307. [PubMed: 11591818]
- 128.
- de la Luna S, Allen KE, Mason SL, La Thangue NB. Integration of a growth-suppressing BTB/POZ domain protein with the DP component of the E2F transcription factor. EMBO J. 1999;18:212–228. [PMC free article: PMC1171116] [PubMed: 9878064]
- 129.
- Mancini MA, Shan B, Nickerson JA, Penman S, Lee WH. The retinoblastoma gene product is a cell cycle-dependent, nuclear matrix-associated protein. Proc Natl Acad Sci USA. 1994;91:418–422. [PMC free article: PMC42959] [PubMed: 8278403]
- 130.
- Dyson N. The regulation of E2F by pRB-family proteins. Genes Dev. 1998;12:2245–2262. [PubMed: 9694791]
- 131.
- Kaelin WG Jr. Functions of the retinoblastoma protein. Bioessays. 1999;21:950–958. [PubMed: 10517868]
- 132.
- Jenkins H, Hölman T, Lyon C, Lane B, Stick R, Hutchison C. Nuclei that lack a lamina accumulate karyophilic proteins and assemble a nuclear matrix. J Cell Sci. 1993; 106:275–285. [PubMed: 7903671]
- 133.
- Goldberg M, Jenkins H, Allen T, Whitfield WG, Hutchison CJ. Xenopus lamin B3 has a direct role in the assembly of a replication competent nucleus: evidence from cell-free egg extracts. J Cell Sci. 1995;108:3451–3461. [PubMed: 8586657]
- 134.
- Spann TP, Moir RD, Goldman AE, Stick R, Goldman RD. Disruption of nuclear lamin organization alters the distribution of replication factors and inhibits DNA synthesis. J Cell Biol. 1997;136:1201–1212. [PMC free article: PMC2132512] [PubMed: 9087437]
- 135.
- Moir RD, Spann TP, Herrmann H, Goldman RD. Disruption of nuclear lamin organization blocks the elongation phase of DNA replication. J Cell Biol. 2000;149:1179–1192. [PMC free article: PMC2175110] [PubMed: 10851016]
- 136.
- Ellis DJ, Jenkins H, Whitfield WG, Hutchison CJ. GST-lamin fusion proteins act as dominant negative mutants in Xenopus egg extract and reveal the function of the lamina in DNA replication. J Cell Sci. 1997;110:2507–2518. [PubMed: 9372440]
- 137.
- Yang L, Guan T, Gerace L. Lamin-binding fragment of LAP2 inhibits increase in nuclear volume during the cell cycle and progression into S phase. J Cell Biol. 1997;139:1077–1087. [PMC free article: PMC2140217] [PubMed: 9382857]
- 138.
- Chen F, Hersh BM, Conradt B, Zhou Z, Riemer D, Gruenbaum Y. et al. Translocation of C. elegans CED-4 to nuclear membranes during programmed cell death. Science. 2000; 287:1485–1489. [PubMed: 10688797]
- 139.
- Buendia B, Santa-Maria A, Courvalin JC. Caspase-dependent proteolysis of integral and peripheral proteins of nuclear membranes and nuclear pore complex proteins during apoptosis. J Cell Sci. 1999;112:1743–1753. [PubMed: 10318766]
- 140.
- Gotzmann J, Vlcek S, Foisner R. Caspase-mediated cleavage of the chromosome-binding domain of lamina-associated polypeptide 2 alpha. J Cell Sci. 2000;113 Pt 21:3769–3780. [PubMed: 11034905]
- 141.
- Rao L, Perez D, White E. Lamin proteolysis facilitates nuclear events during apoptosis. J Cell Biol. 1996; 135:1441–1455. [PMC free article: PMC2133948] [PubMed: 8978814]
- 142.
- Steen RL, Collas P. Mistargeting of B-type lamins at the end of mitosis: implications on cell survival and regulation of lamins A/C expression. J Cell Biol. 2001; 153:621–626. [PMC free article: PMC2190567] [PubMed: 11331311]
- 143.
- Burke B. Lamins and apoptosis: a two-way street? J Cell Biol. 2001;153:F5–7. [PMC free article: PMC2190563] [PubMed: 11331313]
- 144.
- Guillemin K, Williams T, Krasnow MA. A nuclear lamin is required for cytoplasmic organization and egg polarity in Drosophila. Nat Cell Biol. 2001;3:848–851. [PubMed: 11533666]
- 145.
- Bonne G, Mercuri E, Muchir A, Urtizberea A, Becane HM, Recan D, Merlini L, Wehnert M, Boor R, Reuner U, Vorgerd M, Wicklein EM, Eymard B, Duboc D, Penisson-Besnier I, Cuisset JM, Ferrer X, Desguerre I, Lacombe D, Bushby K, Pollitt C, Toniolo D, Fardeau M, Schwartz K, Muntoni F. Clinical and molecular genetic spectrum of autosomal dominant Emery-Dreifuss muscular dystrophy due to mutations of the lamin A/C gene. Ann Neurol. 2000; 48:170–180. [PubMed: 10939567]
- 146.
- Gant TM, Wilson KL. Nuclear assembly. Annu Rev Cell Dev Biol. 1997;13:669–695. [PubMed: 9442884]
- 147.
- Collas I, Courvalin JC. Sorting nuclear membrane proteins at mitosis. Trends Cell Biol. 2000;10:5–8. [PubMed: 10603470]
- 148.
- Thompson LJ, Bollen M, Fields AP. Identification of protein phosphatase 1 as a mitotic lamin phosphatase. J Biol Chem. 1997;272:29693–29697. [PubMed: 9368037]
- 149.
- Steen RL, Martins SB, Tasken K, Collas P. Recruitment of protein phosphatase 1 to the nuclear envelope by A-kinase anchoring protein AKAP149 is a prerequisite for nuclear lamina assembly. J Cell Biol. 2000;150:1251–1262. [PMC free article: PMC2150688] [PubMed: 10995432]
- 150.
- Ellenberg J, Siggia ED, Moreira JE, Smith CL, Presley JF, Worman HJ, Lippincott-Schwartz J. Nuclear membrane dynamics and reassembly in living cells: targeting of an inner nuclear membrane protein in interphase and mitosis. J Cell Biol. 1997;138:1193–1206. [PMC free article: PMC2132565] [PubMed: 9298976]
- 151.
- Dabauvalle MC, Muller E, Ewald A, Kress W, Krohne G, Muller CR. Distribution of emerin during the cell cycle. Eur J Cell Biol. 1999;78:749–756. [PubMed: 10569247]
- 152.
- Buendia B, Courvalin JC. Domain-specific disassembly and reassembly of nuclear membranes during mitosis. Exp Cell Res. 1997;230:133–144. [PubMed: 9013715]
- 153.
- Lourim D, Krohne G. Lamin-dependent nuclear envelope reassembly following mitosis. Trends Cell Biol. 1994;4:324–318. [PubMed: 14731468]
- 154.
- Dabauvalle MC, Loos K, Merkert H, Scheer U. Spontaneous assembly of pore complex-containing membranes (“annulate lamellae”) in Xenopus egg extract in the absence of chromatin. J Cell Biol. 1991; 112:1073–1082. [PMC free article: PMC2288888] [PubMed: 1825658]
- 155.
- Ulitzur N, Harel A, Feinstein N, Gruenbaum Y. Lamin activity is essential for nuclear envelope assembly in a Drosophila embryo cell-free extract. J Cell Biol. 1992; 119:17–25. [PMC free article: PMC2289631] [PubMed: 1527167]
- 156.
- Burke B, Gerace L. A cell free system to study reassembly of the nuclear envelope at the end of mitosis. Cell. 1986;44:639–652. [PubMed: 3948244]
- 157.
- Lopez-Soler RI, Moir RD, Spann TP, Stick R, Goldman RD. A role for nuclear lamins in nuclear envelope assembly. J Cell Biol. 2001;154:61–70. [PMC free article: PMC2196852] [PubMed: 11448990]
- 158.
- Pyrpasopoulou A, Meier J, Maison C, Simos G, Georgatos SD. The lamin B receptor (LBR) provides essential chromatin docking sites at the nuclear envelope. EMBO J. 1996; 15:7108–7119. [PMC free article: PMC452536] [PubMed: 9003786]
- 159.
- Collas P, Courvalin J-C, Poccia D. Targeting of membranes to sea urchin sperm chromatin is mediated by an LBR-like integral membrane protein. J Cell Biol. 1996; 135:1715–1725. [PMC free article: PMC2133942] [PubMed: 8991085]
- Introduction
- Major Components of the Peripheral Nuclear Lamina
- Lamina Proteins in the Nuclear Interior
- Interactions at the Interface Between the Lamina and the Nuclear Scaffold/Chromatin
- Potential Functions of Lamina Proteins in Interphase
- Dynamics and Functions of Lamina-Chromatin Interactions During Mitosis
- Conclusions and Future Prospects
- Acknowledgements
- References
- Dynamic Connections of Nuclear Envelope Proteins to Chromatin and the Nuclear Ma...Dynamic Connections of Nuclear Envelope Proteins to Chromatin and the Nuclear Matrix - Madame Curie Bioscience Database
- Protein Kinase A - Madame Curie Bioscience DatabaseProtein Kinase A - Madame Curie Bioscience Database
- Opioid Receptors on Peripheral Sensory Neurons - Madame Curie Bioscience Databas...Opioid Receptors on Peripheral Sensory Neurons - Madame Curie Bioscience Database
- Nucleotide Exchange Factors for Hsp70 Molecular Chaperones - Madame Curie Biosci...Nucleotide Exchange Factors for Hsp70 Molecular Chaperones - Madame Curie Bioscience Database
- Molecular Biology of Methylenetetrahydrofolate Reductase (MTHFR) and Overview of...Molecular Biology of Methylenetetrahydrofolate Reductase (MTHFR) and Overview of Mutations/Polymorphisms - Madame Curie Bioscience Database
Your browsing activity is empty.
Activity recording is turned off.
See more...