NCBI Bookshelf. A service of the National Library of Medicine, National Institutes of Health.
Madame Curie Bioscience Database [Internet]. Austin (TX): Landes Bioscience; 2000-2013.
A number of recent studies have suggested that the rapid evolution of genes involved in sexual reproduction is driven by conflict between the sexes. Such genes include the ones that have a role in mating behavior, postmating gamete interactions, and fertilization (i.e., sex-related genes). However, in many cases an alternative scenario with males coadapting to female-driven changes appears as an equally likely one. Studies on the molecular evolution of sex-related genes have mainly focused on males, with few exceptions. We suggest that the combined analysis of intraspecific polymorphism and interspecies divergence will allow to make predictions on whether sex-related genes evolution is driven by conflict or coadaptation between the sexes. Such approach, made possible by rapid accumulation of DNA sequence information, will benefit from studies designed to identify male and female gene products that interact with each other during mate signaling, fertilization, and postzygotic development.
Introduction
Sexual selection captures our attention due to its effect on sexual dimorphism, evolution of exaggerated and often maladaptive traits, and its implication for the relationship between sexual conflict and fitness. The concept of sexual selection has been extended from its original meaning, which implied the evolution of secondary sexual traits that confer a mating advantage by making males better competitors against other males or more attractive to females. The extension of the concept includes not only the divergent and rapid evolution of the morphology of primary genitalia and clasping traits directly involved in mating,1 but also that of sperm morphology2-4 and of seminal proteins transferred in the ejaculate.5-9 The extension comes simply from the understanding that male-to-male competition (sperm competition10) and/or female choice (cryptic choice11) are still possible even after copulation is over.
In its original form, sexual selection refers to a process leading to the development of extreme male secondary sexual traits despite of their potentially detrimental effect on survival. While natural selection draws our attention to differences in viability, sexual selection focuses on differences in mating success. It is usually entertained that males increase their overall fitness at the expense of survival by increasing their chances to be chosen for mating. Females increase their fitness in an indirect way by choosing males that will provide them with successful male progeny (thus propagating the female's genes). The result is a constant selection of males with the appropriate signals until the male traits become so elaborate or so extreme that the balance between survival and reproductive advantage is upset by the high cost of the trait. Although fitness is presented in terms of separate (male or female) sexes, the end result is an overall increase in fitness at the population level.
However, a side consequence of thinking in terms of distinct sexes is a tendency to appraise fitness of males and females separately. When such dissociation is made, female's fitness is usually considered in terms of her overall reproductive advantage rather than in terms of her viability. In recent years, the idea that the viability aspect of female's fitness could be impaired by the evolution of male traits that increase his mating success has gained support. The result is the concept of conflict, or arm races, between the sexes—a constant struggle in which male traits evolve to extremes to secure mating at the expense of being detrimental to their partners.
While it has become customary to calculate fitness for each sex separately, it is obvious that the population fitness depends on the interaction and coadaptation of both sexes. Separate sexes cannot maximize their fitness indefinitely, and not all reproductive traits are subject to sexual conflict. Sex-specific signals to the members of the conspecific opposite sex, complementarity of genitalic structures, and egg-sperm interactions are just a few examples of traits that would be subject to coadaptation rather than to conflict. Clasping structures, mating frequency, and sperm competition are the obvious examples of conflict traits. However, in many cases it is not clear whether the trait is likely to have evolved under male-female coadaptation or sexual conflict scenario. We propose that such cases could be resolved by analysis of the dynamics of genetic polymorphism and divergence for candidate genes under the contrasting scenarios of sexual conflict vs. sexual coadaptation.
Are Males Conflicting with Females or Coadapting to Them?
The use of the “conflict” or “war” term is not restricted to the field of sexual selection and evolution. It is a widespread and attractive term that is widely applied to political, sociological, and health related issues (the war against cancer, the war on drugs, etc).
There are clear examples of the harm potentially inflicted by males to females during courtship and mating. At the mating level, it is usually forgotten that such harm is not necessarily beneficial to the male. For example, it has been recently shown that even though sexual selection may favor male bowerbirds that display intensively and therefore appear more attractive, a tradeoff is in place because excessive intensity is perceived as aggressive behavior threatening to females; such potentially harmful performance leads to an end of courtship.12 This is a very important idea in terms of the potential effect of conflict between the sexes because during courtship behaviors should favor communication that will benefit both sexes. In a situation where males were allowed to overcome male-females tradeoffs in terms of access to mating, males with higher sexual activity (access to females) showed a diminished antibacterial immune reaction, suggesting a cost of male sexual activity that is irrelevant to the female's response.13
In a recent paper, Arnqvist and Rowe demonstrated a constant coadaptation between the sexes in water striders, with deviations from such male-female coadaptations leading to rapid evolution.14 In the species analyzed, males have evolved clasping genitalia to grasp females, and females have evolved counteradaptations to resist grasping, suggesting an arm-race between the sexes. However, it is not usually easy to establish whether male-female coevolution results as a consequence of conflict or of mutual benefit between the sexes. In species where there is male-to-male competition, developing of traits that facilitate access to females or improve sperm competitiveness may have side effects deleterious to females.14,15 Such pattern of sexual conflict has been proposed to promote speciation, because polyandrous species show a higher speciation rate.16 Another possible explanation of high speciation rate is coadaptation leading to fine-tuning of male and female reproductive systems, as seems to be the case for beetles where sperm of males from the same population as females outcompetes sperm of male from an allopatric population.17
Among Drosophila species, the evolution of long sperm is correlated with longer sperm storage organs in females.18 Experiments with selection for divergent sperm length or size of female sperm storage organs in D. melanogaster revealed that male — female coadaptation is responsible for such correlations.19 Drosophila females' lifespan is affected due to male seminal fluids transferred in the ejaculate,20 with the effect being more drastic when the male is a strong sperm competitor.15 An interesting observation is that despite the cost of mating, mated females live longer than virgins.15 Similar results have been shown for the Meditaeranean fruit fly Ceratitis capitata where virgin females experience lower mortality than mated females only during the first 20 days after eclosion.21 It appears that there is a compensation to the cost on viability that females have to pay shortly after mating, as mated females live longer than virgins.15,21
Sexual conflict has been implicated in rapid evolution of genes with a role in fertilization, but obviously it does not represent a single driving force behind the phenomenon. In other words, rapid evolution of a gene involved in fertilization does not necessarily imply a sexual conflict. In this chapter we first examine what we have learned from studies on rapid molecular evolution of immune system genes, where there is a clear arm-race between the infective agent and the infected organism. We then explore the pattern of rapid molecular evolution of genes with a role in mate recognition, sperm competition and/or fertilization (i.e., sex-related genes). Finally, we make predictions of what would be commonly observed for genes rapidly evolving under conflict versus those simply involved in a rapid coadaptation between males and females.
Conflict Scenarios: Balancing Selection in Rapidly Evolving Immune System Genes
One of the best-understood examples of adaptive evolution is that of the Major Histocompatibility Complex (MHC) in vertebrates. MHC proteins are expressed on the cell surface where they present small peptides to cytotoxic T lymphocytes or helper T cells. The efficiency of the immune response to battle a wide variety of infections depends on the ability of the MHC system to present a wide variety of peptides that can be recognized as foreign. The resolution of the molecular structure of MHC molecules22,23 was crucial for understanding of the role played by selection in the evolution of these proteins. The peptide binding region of MHC molecules became a logical target for the prediction of an elevated polymorphism that will allow a wide variety of alleles capable of recognizing and presenting multiple antigens. Indeed, the peptide binding region has the highest proportion of nonsynonymous to synonymous polymorphic sites within the protein,24,25 indicative of balancing selection. The level of polymorphism at peptide binding regions apparently depends on the level of variability of the peptides that it binds.26 Therefore, parasites represent the major selective force that drives evolution of the protein-binding region of MHC molecules.
On the other side of the barricades, there are parasites that try to escape recognition by the immune system. For example, in Plasmodium falciparum, an unusually high proportion of nonsynonymous substitutions was found in the region of the circumsporozoite protein (CSP) which provides the peptides recognized by the MHC molecules.27 An additional intriguing twist to the arm-race scenario in the case of malaria is introduced by cross-reactivity between the epitopes of Plasmodium falciparum and the cytotoxic T-lymphocytes (CTL) epitopes, which results in selective pressure on human leukocyte proteins.28 During HIV infection, the host antibody response can act as a strong selective agent targeting surface proteins of the virus. Sequence analyses of a series of hypervariable regions in these proteins suggest that amino acid polymorphisms are favored by positive selection, but that different lineages of the virus show different responses depending on the nature of the host environment.29 Again, an arm-race between infectious agents and the host leads to the rapid accumulation of polymorphisms that can help escape recognition by the host immune system.30,31
Other recognition systems, such as self-incompatibility genes in plants, and pheromone-receptor recognition in fungi, also show evidence for balancing selection by preservation of multiple alleles.32-34 The common pattern is high polymorphism within species combined with rapid divergence between species.35
External Fertilization: How Are Marine Invertebrate Sperm Surface Proteins Different from Immune System Genes?
In sea urchins, the sperm surface protein bindin mediates binding to the egg. The protein is not only highly divergent between species but also highly polymorphic within species.36-38 The high polymorphism within species could suggest a situation of sexual conflict with male bindin proteins being in a state of male-to-male competition for the eggs. In a sexual conflict scenario where males control the results of fertilization, the bindin proteins for which females have not yet coevolved proper defense would be more successful. However, Palumbi39 showed that sea urchins could be grouped into different clades according to the sequence of their bindin alleles, and that males are more efficient at fertilizing eggs of the same genotype, suggesting female cryptic choice scenario as opposed to sexual conflict. Male-to-female coadaptation within the population, where different bindin alleles work better with akin partners, apparently have maintained high polymorphism and the system seems to be female driven.
In another marine invertebrate, abalone, both the sperm surface protein (lysin) and its receptor on the egg (VERL) have been identified. Swanson and Vacquier40 have shown that the lysin-binding motif of VERL is a large glycoprotein with 22 tandem repeats which shows rapid divergence, but no sign of positive selection. They demonstrated that concerted evolution of the VERL repeats leads to species-specific phylogenetic grouping, and proposed that redundancy of its tandem repeat structure reduces selective pressures on the receptor. The spread of accumulated mutations within VERL by unequal crossing-over and gene conversion imposes new selective pressures on lysin, so that species-specific fertilization evolves as a consequence of males having to adapt to a rapidly neutrally evolving sperm receptor on the egg surface.40,41
The examples coming from studies on marine invertebrates with an external fertilization system show a situation in which female sperm receptors are not only essential for proper fertilization, but also are the source of selective pressure on male sperm proteins that are constantly adapting to the female-driven changes. The marine invertebrate system clearly shows the need for a proper understanding of who are the key players in sperm-egg interactions during fertilization, before we can make predictions as to whether rapid evolution of male reproductive proteins driven by positive selection is a consequence of sexual conflict or of male-to-female coadaptation.
Internal Fertilization: Lessons from Drosophila
Drosophila males produce secretions in a pair of accessory glands (called also paragonia) and transfer these secretions in their ejaculate. The paragonia represent a component of the male's internal genitalia. The potential effect of accessory gland proteins on the female physiology and behavior has been suggested in different experimental settings. Accessory gland proteins transferred during copulation have been found to be responsible for triggering a series of postcopulatory female responses, such as increased ovulation and delayed remating.42
Several studies also suggested that accessory gland secretions are capable of affecting female's longevity after mating. When Drosophila males lacking the accessory gland secretions are compared to intact males, only the males that transfer accessory gland secretions during copulation are able to reduce average longevity of female.20 The specific factors that are responsible for this effect remain elusive. However, the most likely candidate is Acp62F, an accessory gland protein capable of entering the haemolymph of females after mating,43 and the only accessory gland product proven to be toxic to females and males when ectopically expressed.44
Why would males transfer protein(s) that inflict a damage to females by reducing their viability? One likely possibility is that the transfer of such proteins provides a benefit in terms of male reproductive ability. We know that there is a significant and negative correlation between sperm competitiveness and female viability shortly after mating,15 and it appears that mating provides a long-term survival advantage for females.15,21 An antagonistic arm race between the sexes may start as a consequence of a male toxicity, with females evolving responses to escape the harmful effect of male's ejaculate and with males readjusting to the new female environment. Specific mechanisms on the female side could include a rapid evolution of female receptors to escape effects of the male proteins or, perhaps, developing of female reproductive gland secretions that can neutralize the male's accessory gland secretions. A likely scenario is the one in which males have evolved mechanisms that harm females after mating, because it provides an immediate advantage in terms of male-to-male competition. Such situation is tolerated, because females acquired a mating-induced long-term survival advantage. Therefore, the connection between competitiveness of male sperm and cost of mating in terms of female longevity seems to be more complex than a simple toxic effect inflicted by seminal fluids.
Arm-Race vs. Sex-Specific Coadaptation: Test of Hypotheses
Female Response: Are There Female Counterparts for Male Proteins?
Very little is known about the pattern of molecular evolution of female reproductive proteins. Civetta and Singh45 showed that proteins expressed in Drosophila ovary tissue are as highly divergent between species as are male reproductive proteins. More recently, it has been shown that zona pellucida proteins in mammalian eggs display signs of rapid divergence shaped by positive selection.46
How helpful is the information on the evolutionary patterns of female reproductive tissue proteins in deciding between the conflict versus the coadaptation scenarios? While the conflict scenario is male driven, the lack of conflict implies that females are choosing and males are coadapting to the new requirements introduced by females. It is possible to test conflict vs. coadaptation by examining the combined pattern of molecular evolution of male and female proteins that interact at various stages of gamete recognition and fertilization. Under the coadaptation hypothesis, the expectation is that female proteins will show a higher proportion of differences between populations than the male counterparts, at least in early stages of differentiation between populations. However, the pattern might not hold in a case where the female component (e.g., sperm receptor, reproductive gland proteins) is duplicated and therefore there is no one-to-one relationship between the male and the female counterparts, as in the case of the lysin/VERL system.40,47
A conflict scenario will predict males driving divergence between populations and therefore male proteins having higher levels of polymorphism. A major limitation to the test is the lack of identified female reproductive proteins that interact with well-characterized male counterparts such as the accessory gland proteins in Drosophila. A general picture of the situation in Drosophila can be inferred from our study on protein divergence between pairs of species from the virilis group.45 This is an interesting group, since it offers pairs of species with different levels of postmating prezygotic isolation, from the pairs capable of producing viable and fertile offspring to the pairs producing no viable/fertile progeny. Comparisons between species belonging to the virilis phylad, where flies are capable of interspecific hybridization yielding fertile progeny, show that the level of protein divergence found in ovaries is higher than that in testes for some species pairs. This observation suggests that females are driving the direction of evolution of male traits, implying the coadaptation scenario. However, larger samples and a more detailed analysis of reproductive isolation in the virilis group species are needed for a convincing claim.
Another more recent study in mammals analyzed the pattern of molecular evolution of three zona pellucida proteins that are either known or suspected to play a key role during sperm recognition and binding. All three proteins showed signs of positive selection, and specific residues that may be involved in species-specific gamete interactions were identified.46 However, to formally test whether such pattern of positive selection is male (conflict) or female (cryptic choice) driven, we need a simultaneous analysis of the pattern of evolution of the interacting sperm proteins, including the amount and pattern of divergence and phylogenetic clustering of male and female alleles (fig. 1).
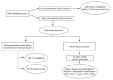
Figure 1
Predictive patterns of genetic polymorphism and interspecific divergence for genes evolving under sexual conflict or sexual coadaptation.
Male Reproductive Proteins: Patterns of Polymorphism and Divergence
Given the current scarcity of data on molecular evolution of female reproductive genes, assumptions on the mode of evolution of sex-related genes are often based on analysis of just the male reproductive genes. While this approach lacks the meticulousness of the aforementioned simultaneous analysis of the male and female counterparts, it is valid for discriminating between the two possible rationales for the rapid divergence of reproductive genes, i.e., between the conflict and the coadaptation scenarios. If conflict between the sexes is in place, the expectation for the patterns of molecular evolution should be similar to that of a parasite-host situation, where the alleles of female genes which confer resistance to male harmful proteins are beneficial. We expect that such balancing selection would promote an elevated proportion of replacement polymorphisms and would result in coexistence of multiple ancient alleles (fig. 1). The effect of selection could be localized at specific sites or regions within the genes, or could be more widespread as a consequence of a hitchhiking effect (selective sweep). The strength of the sweep depends on the level of intragenic recombination. The expectation in terms of the molecular evolutionary pattern between species depends on the intensity of selection, but it is possible that we might see polymorphic sites shared between species, as well as fixed differences.
An alternative scenario is the one of sex-specific coadaptation, where male and female genes involved in reproduction are under purifying selection within species, showing low polymorphism (in particular, nonsynonymous polymorphism). In regions of low recombination, the removal of deleterious mutant alleles will strongly reduce polymorphism according to the background selection model.48 If adaptive selective pressures in different species differentially fix nonsynonymous variants in these genes, an elevated proportion of nosynonymous substitutions is expected between species. Such situation is anticipated if adaptive differentiation at sex-related genes is driving speciation. The prediction is therefore one of low polymorphism, in particular nonsynonymous polymorphism, within species but elevated proportion of nonsynonymous changes between species (fig. 1).
Conclusion
We expect natural selection to optimize sex-related traits with respect to their effects on reproduction and fitness. However, sexual inequality created by differences in allocation of resources, and competition within or between the sexes, can lead to evolution of exaggerated and often maladaptive traits. The concept of sexual selection has been extended to include both pre copulation and post-copulation interactions between males and females or between their gametes, respectively. The traits covered under this broad definition of sexual selection35 encompass those that have evolved by sexual coadaptation as well as by sexual conflict. In this chapter we compared the evolutionary dynamics of the genes involved in pathogen/host interactions and of the sexual reproduction genes, and made predictions on the patterns of genetic polymorphism and divergence for genes experiencing sexual coadaptation vs. sexual conflict. The predictions provide a tool to deduce reasons for the rapid evolution of individual sex-related genes. This approach will utilize rapidly accumulating whole-genome DNA sequence data, thus providing a wealth of information on the evolutionary dynamics of sex-related genes.
References
- 1.
- Eberhard WG. Sexual selection and animal genitalia. Cambridge: Harvard University Press. 1985
- 2.
- Pitnick S, Markow TA. Male gametic strategies: Sperm size, testes size, and the allocation of ejaculate among successive mates by the sperm-limited fly Drosophila pachea and its relatives. Am Nat. 1994;143:785–819.
- 3.
- Joly D, Bressac C, Lachaise D. Disentangling giant sperm. Nature. 1995;377:202. [PubMed: 7675105]
- 4.
- Karr TL, Pitnick S. The ins and outs of fertilization. Nature. 1996;379:405–406. [PubMed: 8559245]
- 5.
- Coulthart MB, Singh RS. High level of divergence of male-reproductive-tract proteins, between Drosophila melanogaster and its sibling species, D. simulans. Mol Biol Evol. 1988;5:182–191. [PubMed: 3130539]
- 6.
- Aguadé M, Miyashita N, Langley CH. Polymorphism and divergence in the Mst26A male accessory gland gene region in Drosophila. Genetics. 1992;132:755–770. [PMC free article: PMC1205212] [PubMed: 1361475]
- 7.
- Tsaur S-C, Wu C-I. Positive selection and the molecular evolution of a gene of male reproduction, Acp26Aa of Drosophila. Mol Biol Evol. 1997;14:544–549. [PubMed: 9159932]
- 8.
- Aguadé M. Different forces drive the evolution of the Acp26Aa and Acp26Ab accessory gland genes in the Drosophila melanogaster species complex. Genetics. 1998;150:1079–1089. [PMC free article: PMC1460384] [PubMed: 9799260]
- 9.
- Aguadé M. Positive selection drives the evolution of the Acp29AB accessory gland protein in Drosophila. Genetics. 1999;152:543–551. [PMC free article: PMC1460617] [PubMed: 10353898]
- 10.
- Parker GA. Sperm competition and its evolutionary consequences in insects. Biological Reviews. 1970;45:525–567.
- 11.
- Eberhard WG. Female control: Sexual selection by cryptic female choice Princeton: PrincetonUniversity Press,1996.
- 12.
- Patricelli GL, Uy JAC, Walsh G. et al. Male displays adjusted to female's response. Nature. 2002;415:279–280. [PubMed: 11796996]
- 13.
- McKean KA, Nunney L. Increased sexual activity reduces male immune function in Drosophila melanogaster. Proc Natl Acad Sci USA. 2001;98:7904–7909. [PMC free article: PMC35441] [PubMed: 11416162]
- 14.
- Arnqvist G, Rowe L. Antagonistic coevolution between the sexes in a group of insects. Nature. 2002;415:787–789. [PubMed: 11845208]
- 15.
- Civetta A, Clark AG. Correlated effects of sperm competition and post-mating female mortality. Proc Natl Acad Sci USA. 2000;97:13162–13165. [PMC free article: PMC27195] [PubMed: 11078508]
- 16.
- Arnqvist G, Edvardsson M, Friberg U. et al. Sexual conflict promotes speciation in insects. Proc Natl Acad Sci USA. 2000;97:10460–10464. [PMC free article: PMC27046] [PubMed: 10984538]
- 17.
- Brown DV, Eady PE. The functional incompatibility between the fertilization systems of two allopatric populations of Callosobruchus maculatus (Coleoptera: Bruchidae). Evolution. 2001;55:2257–2262. [PubMed: 11794785]
- 18.
- Pitnick S, Markow TA, Spicer GS. Evolution of multiple kinds of female sperm-storage organs in Drosophila. Evolution. 1999;53:1804–1822. [PubMed: 28565462]
- 19.
- Miller GT, Pitnick S. Sperm-female coevolution in Drosophila. Science. 2002;298:1230–1233. [PubMed: 12424377]
- 20.
- Chapman T, Liddle LF, Kalb JM. et al. Cost of mating in Drosophila melanogaster females is mediated by male sccessory gland products. Nature. 1995;373:241–244. [PubMed: 7816137]
- 21.
- Carey JR, Liedo P, Harshman L. et al. A mortality cost of virginity at older ages in female Mediterranean fruit flies. Exp Gerontol. 2002;37:507–512. [PubMed: 11830353]
- 22.
- Lawlor DA, Zemmour J, Enis PD. et al. Evolution of class-I MHC genes and proteins: From natural selection to thymic selection. Annu Rev Immunol. 1990;8:23–63. [PubMed: 2188663]
- 23.
- Jardetzky TS, Brown JH, Gorga JC. et al. Three-dimensional structure of a human class II histocompatibility molecule complexed with superantigen. Nature. 1994;368:711–718. [PubMed: 8152483]
- 24.
- Hughes AL, Nei M. Pattern of nucleotide substitution at major histocompatibility complex class I loci reveals overdominant selection. Nature. 1988;335:167–70. [PubMed: 3412472]
- 25.
- Hughes AL, Hughes MK, Howell CY. et al. Natural selection at the class II major histocompatibility complex loci of mammals. Philos Trans R Soc Lond B Biol Sci. 1994;346:359–367. [PubMed: 7708830]
- 26.
- Hughes AL, Hughes MK. Natural selection on the peptide-binding regions of major histocompatibility complex molecules. Immunogenetics. 1995;42:233–243. [PubMed: 7672817]
- 27.
- Hughes AL, Hughes MK. Natural selection on Plasmodium surface proteins. Mol Biochem Parasitol. 1995;71:99–113. [PubMed: 7630387]
- 28.
- Gilbert SC, Plebanski M, Gupta S. et al. Association of malaria parasite population structure, HLA, and immunological antagonism. Science. 1998;279:1173–1177. [PubMed: 9469800]
- 29.
- Seibert SA, Howell CY, Hughes MK. et al. Natural selection on the gag, pol, and env genes of human immunodeficiency virus 1 (HIV-1). Mol Biol Evol. 1995;12:803–813. [PubMed: 7476126]
- 30.
- Klenerman P, Rowland-Jones S, McAdam S. et al. Cytotoxic T-cell activity antagonized by naturally occurring HIV-1 Gag variants. Nature. 1994;369:403–407. [PubMed: 7515165]
- 31.
- Price DA, Goulder PJR, Klenerman P. et al. Positive selection of HIV-1 cytotoxic T lymphocyte escape variants during primary infection. Proc Natl Acad Sci USA. 1997;94:1890–1895. [PMC free article: PMC20013] [PubMed: 9050875]
- 32.
- Uyenoyama MK. Genealogical structure among alleles regulating self-incompatibility in natural populations of flowering plants. Genetics. 1997;147:1389–1400. [PMC free article: PMC1208260] [PubMed: 9383079]
- 33.
- Saupe SJ, Glass NL. Allelic specificity at the het-c heterokaryon incompatibility locus of Neurospora crassa is determined by a highly variable domain. Genetics. 1997;146:1299–1309. [PMC free article: PMC1208076] [PubMed: 9258675]
- 34.
- Vaillancourt LJ, Raudaskowski M, Spetch CA. et al. Multiple genes encoding pheromones and a pheromone receptor define the Bβ1 mating-type specificity in Schizophyllum commune. Genetics. 1997;146:541–551. [PMC free article: PMC1207996] [PubMed: 9178005]
- 35.
- Civetta A, Singh RS. Broad-sense sexual selection, sex gene pool evolution, and speciation. Genome. 1999;42:1033–1041. [PubMed: 10659767]
- 36.
- Metz EC, Palumbi SR. Positive selection and sequence rearrangements generate extensive polymorphism in the gamete recognition protein bindin. Mol Biol Evol. 1996;13:397–406. [PubMed: 8587504]
- 37.
- Metz EC, Gomez-Gutierrez G, Vacquier VD. Mitochondrial DNA and bindin gene sequence evolution among allopatric species of the sea urchin genus Arbacia. Mol Biol Evol. 1998;15:185–195. [PubMed: 9491615]
- 38.
- Metz EC, Robles-Sikisaka R, Vacquier VD. Nonsynonymous substitution in abalone sperm fertilization genes exceeds substitution in introns and mitochondrial DNA. Proc Natl Acad Sci USA. 1998;95:10676–10681. [PMC free article: PMC27954] [PubMed: 9724763]
- 39.
- Palumbi SR. All males are not created equal: Fertility differences depend on gamete recognition polymorphisms in sea urchins. Proc Natl Acad Sci USA. 1999;96:12632–12637. [PMC free article: PMC23023] [PubMed: 10535974]
- 40.
- Swanson WJ, Vacquier VD. Concerted evolution in an egg receptor for a rapidly evolving abalone sperm protein. Science. 1998;281:710–712. [PubMed: 9685267]
- 41.
- Swanson WJ, Vacquier VD. The rapid evolution of reproductive proteins. Nat Rev Genet. 2002;3:137–144. [PubMed: 11836507]
- 42.
- Wolfner MF. The gifts that keep on giving: Physiological functions and evolutionary dynamics of male seminal proteins in Drosophila. Heredity. 2002;88:85–93. [PubMed: 11932766]
- 43.
- Lung O, Wolfner MF. Identification and characterization of the major Drosophila melanogaster mating plug protein. Insect Biochem Mol Biol. 1999;29:1043–1052. [PubMed: 11267893]
- 44.
- Lung O, Tram U, Finnerty CM. et al. The Drosophila melanogaster seminal fluid protein Acp62F is a protease inhibitor that is toxic upon ectopic expression. Genetics. 2002;160:211–224. [PMC free article: PMC1461949] [PubMed: 11805057]
- 45.
- Civetta A, Singh RS. High divergence of reproductive tract proteins and their association with postzygotic reproductive isolation in Drosophila melanogaster and Drosophila virilis group species. J Mol Evol. 1995;41:1085–1095. [PubMed: 8587107]
- 46.
- Swanson WJ, Yang Z, Wolfner MF. et al. Positive Darwinian selection drives the evolution of several female reproductive proteins in mammals. Proc Natl Acad Sci USA. 2001;98:2509–2514. [PMC free article: PMC30168] [PubMed: 11226269]
- 47.
- Nei M, Zhang J. Molecular origin of species. Science. 1998;282:1428–1429. [PubMed: 9867649]
- 48.
- Chalesworth B, Morgan MT, Charlesworth D. The effect of deleterious mutations on neutral molecular variation. Genetics. 1993;134:1289–1303. [PMC free article: PMC1205596] [PubMed: 8375663]
- Introduction
- Are Males Conflicting with Females or Coadapting to Them?
- Conflict Scenarios: Balancing Selection in Rapidly Evolving Immune System Genes
- External Fertilization: How Are Marine Invertebrate Sperm Surface Proteins Different from Immune System Genes?
- Internal Fertilization: Lessons from Drosophila
- Arm-Race vs. Sex-Specific Coadaptation: Test of Hypotheses
- Conclusion
- References
- Rapid Evolution of Sex-related Genes: Sexual Conflict or Sex-specific Adaptation...Rapid Evolution of Sex-related Genes: Sexual Conflict or Sex-specific Adaptations? - Madame Curie Bioscience Database
Your browsing activity is empty.
Activity recording is turned off.
See more...