NCBI Bookshelf. A service of the National Library of Medicine, National Institutes of Health.
Madame Curie Bioscience Database [Internet]. Austin (TX): Landes Bioscience; 2000-2013.
We discuss insights into integrin function obtained through phenotypic and structure function analysis of integrins and associated proteins in the fruitfly, Drosophila melanogaster. Integrins contribute to fly development by mediating both dynamic and stable adhesive interactions. The minimal redundancy of proteins involved and the ease with which they can be manipulated in the context of the whole organism and studied at the cellular level make it an ideal model organism to study the “core” complexes that mediate diverse integrin functions. Such an analysis has revealed a hierarchy of molecular interactions that mediate stable adhesion between two layers of cells through an intervening extracellular matrix.
Introduction
The fruitfly Drosophila melanogaster has proved to be a valuable model organism for the study of integrin biology. Apart from the obvious advantage of being genetically tractable, integrin biology has benefited from the fact that integrins and their associated proteins are well conserved in the fly and encoded by smaller numbers of genes. This makes the analysis of function of a particular type of protein (by removing it from an otherwise normal animal) much easier in the fly than in vertebrate organisms. Thus, it is possible to test what a particular protein is actually required for in the intact organism, which sometimes differs significantly from what it can do when perturbed or overexpressed in cells in culture.
The wealth of knowledge on the mechanisms of development in Drosophila has made it possible to put integrin function within the framework of developmental decisions and events. Of course the relatively simple body plan of Drosophila precludes the analysis of some integrin dependant functions that are of great interest in humans and take place in specialized cell types that flies lack (for example, lymphocyte activation, platelet aggregation and neural crest migration). Nonetheless, many different cell types in Drosophila, including haemocytes (the blood cells of the fly), are migratory and some of these depend on integrins for their migration. We view Drosophila as a means to identify a “core” integrin complex that was present in the metazoan ancestor that gave rise to both flies and humans. By identifying this core complex, we anticipate that we will understand how the basic integrin machinery has become modified during evolution to perform an increased variety of functions.
Here we highlight the insights into integrin function obtained through studies in Drosophila. The reader is also referred to previous reviews.1-3 In our view, we have learned two main lessons. The first is that some integrin-associated molecules are more important than others and that there is a molecular hierarchy among integrin associated proteins. The second is that almost all integrin dependent processes during development involve two groups of cells that interact via integrins and an intervening extracellular matrix (ECM). Thus, rather than thinking of integrins as mediating cell adhesion to a substrate, it is more accurate to think of them as mediating cell-ECM-cell interactions.
Integrins and Associated Proteins: Simplicity Is a Good Thing
The completion of the fly genome sequence allowed a clear tabulation of how many integrin subunits flies have, and how many of the integrin-associated proteins identified in vertebrates are conserved in Drosophila4 (Tables 1, 2, 3, 4, 5, 6). The fly has 5 α integrin subunits (αPS1-5) and 2 β integrin subunits (βPS and βν [beta-nu]). The PS is historical, referring to the fact that the distribution of integrins was found to be position specific rather than cell type specific.5 It remains a useful part of the name, since for example αPS1 is not closely related to vertebrate α1, but instead is equally similar to α3, α6 and α7. In almost all cases there is a single orthologue in flies of each cytoplasmic integrin-associated protein, compared to up to 4 closely related proteins in vertebrates. The ECM proteins that make basement membranes are well conserved, but many other ECM proteins found in vertebrates, notably fibrillar collagens and fibronectin, are not present in the fly.4 Flies also lack cytoplasmic intermediate filaments and the specialised integrin subunit that connects with them, β4. As C. elegans has both fibrillar collagens and cytoplasmic intermediate filaments (although not a β4 integrin subunit), these appear to have been lost in the fly, rather than not present in the common ancestor of flies and humans.
Table 1
Drosophila Integrin genes and their mutant phenotypes.
Table 2
Integrin associated proteins whose loss of function phenotypes includes defects in integrin-mediated adhesion in muscles or the wings.
Table 3
Drosophila integrin associated proteins whose mutant phenotype includes dorsal closure defects, but not defects in strong integrin-mediated adhesion at muscle attachment sites.
Table 4
Drosophila integrin associated proteins whose reported mutant phenotypes do not have any overlap with integrin mutant phenotypes.
Table 5
Drosophila integrin associated proteins for which mutations do not yet exist.
Table 6
Integrin associated proteins for which no orthologues exist in the fly.
Genetics of Integrins and Integrin-Associated Proteins
Mutations have been isolated within many but not all of the genes encoding Drosophila integrins and their associated proteins. There are three sources of these mutations.
The first source is from mutations that were identified because they caused a phenotype of interest and were kept within the fly community. Subsequent molecular characterisation of these mutant genes revealed that some encode integrins or integrin-associated proteins. For example, the first reported allele in an integrin gene was described in 1918 as a mutation that caused blisters in the wings.6 The gene was therefore named inflated and was subsequently found to encode the integrin αPS2 subunit. Other genes of this type are myospheroid (βPS),7 wing blister (one of the two laminin α subunits)8 and blistery (tensin).9
Based on the hypothesis that the wing blister phenotype, which results in the separation of the two normally closely apposed wing epithelial layers, would be diagnostic of mutations in genes encoding integrins and their associated proteins, new genetic screens were performed. Some of these were designed to isolate mutations specifically in the αPS1 subunit gene,10 while other more exhaustive screens were aimed at identifying the majority of genes in the genome that can cause this phenotype when mutated).11,12 These screens made use of a newly developed genetic trick, mitotic recombination using FLP-FRT, to screen mosaic flies that were mostly heterozygous for the newly generated mutations, but had clones of cells within the wing that were homozygous mutant. In this way genes needed for adhesion in the wing that are also required at earlier stages of development, such as the development of the embryo, could still be recovered. All of the genes recovered in these screens have not been characterised yet, but this approach has so far yielded mutations in three genes encoding integrin-associated proteins: a spectraplakin cytoskeletal linker (short stop, previously known as kakapo,13,14), which is an orthologue of vertebrate ACF7/MACF1 and BPAG1; the cytoskeletal linker talin (rhea,15); and the LIM domain adaptor protein PINCH (steamer duck,16). Although the hope was that these screens would identify proteins essential for integrin function that had not been previously identified, there is yet to be an example of this.
The third source is from reverse genetic approaches aimed at isolating mutations in particular genes of interest. A tool that has greatly aided these efforts is the generation of large collections of insertions of modified transposable elements and the mapping of their site of insertion.17 The transposon may or may not inactivate the gene when it inserts nearby (the P-element transposon, which is the basis for the majority of these lines, preferentially inserts close to the promoter). However, the ability to induce the transposon to jump out has been used very effectively to cause the deletion of sequences adjacent to the site of insertion (in approximately 2% of the cases). In the ideal case, the generated deletion will remove enough of the gene of interest to generate a molecular null, while not affecting the adjacent genes. Mutations in the genes encoding the integrin ECM ligand tiggrin,44 the integrin βν subunit,19 focal adhesion kinase (FAK)20 and definitive null mutations in the gene encoding tensin21,22 were recovered this way. A mutation in integrin-linked-kinase (ILK) was identified within a collection of lethal mutations that had been generated by more standard classical genetic methods.23
Some of the sophisticated molecular genetic tools available in Drosophila that have been applied to the study of integrins include (i) insertion of single copies of modified genes into the genome, allowing the rigorous testing of the importance of functional domains within proteins; (ii) the elimination of the maternal contribution of a gene, enabling the complete removal of a gene product from the developing embryo, and (iii) advanced techniques for generating mosaic animals that contain mutant cells within an otherwise normal animal, facilitating a direct comparison of wild type and mutant cells side by side in the intact animal (as is achieved in the mouse with the Cre/loxP system). These tools have made it feasible to study the precise roles and requirements of particular proteins in the context of the whole organism.
We will first describe the genetics of integrins and their associated proteins and then go on to describe what these mutations have told us about the role of integrins in development and the mechanisms of integrin function.
Integrin Genes
As mentioned, the fly has a small repertoire of integrin subunits (5α and 2β).3,4 The βPS subunit appears to be the orthologue of vertebrate β1, but this cannot be deduced on the basis of sequence conservation alone, since βPS has similar levels of sequence conservation with more than one vertebrate β subunit (fig. 1). A very comprehensive analysis of the embryonic mutant phenotype caused by the absence of βPS was performed by Ted Wright in 1960, and impressively, he predicted that the gene product would have a role in the formation of the ECM. He named the gene myospheroid (mys) based on the most prominent aspect of the phenotype, the rounding up of the muscles.7
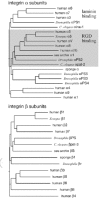
Figure 1
Phylogenetic tree of selected integrin α and β subunits.
We suspect that βPS forms heterodimers with all five α subunits, but this has only been demonstrated for αPS1-3. The sequence similarity of the αPS1 subunit with the vertebrate α3, α6 and α7 extends to ligand preference, as αPS1βPS binds to laminin.24 The gene encoding αPS1 was named multiple edematous wings (mew),10 due to the wing blister phenotype. The αPS2 subunit is equally similar to vertebrate α5, α8, αV and αIIb, and αPS2βPS shares with these integrins the ability to bind ligands containing the tripeptide sequence RGD.25 The three remaining integrin subunits, αPS3-5, are very closely related but do not have a clear orthologue in vertebrates (all 5 α subunits lack an I domain). The αPS3βPS heterodimer binds to both forms of laminin.26,27 The αPS3-5 subunits appear to be the result of recent duplication events, because the Drosophila pseudoobscura genome contains only one, in addititon to αPS1 and αPS2 (our observations). In Drosophila melanogaster, the transcription units encoding αPS3 and αPS4 are adjacent, suggesting that they could be controlled by the same cis-regulatory elements, which may explain some of the illogical aspects of the molecular genetics of this locus. The locus is named scab because of the scab in the embryonic epidermis that results from a failure in dorsal closure,28 and also volado (forgetful), because of defects in short term memory.29 Mutations in scab clearly affect αPS3, so the αPS3 gene is often thought of as equivalent to the scab gene. However, it is not clear whether these mutations also affect αPS4, so some caution should be exercised in interpreting the data on scab mutants until this is cleared up. Mutations in the gene encoding αPS5 have yet to be described.
The second β subunit, βυ, was identified by sequence homology, but is not an obvious orthologue of any vertebrate β subunit (fig. 1), and was found to be only expressed at high levels in the endoderm of the developing gut.30 The recent generation of null mutations in the gene encoding βν has shown that it is not essential for viability nor fertility.19 However, the loss of both βν and βPS caused more severe phenotypes, compared to the loss of βPS alone, but only in the developing gut, the tissue in which βυ is expressed. Thus, in all tissues tested besides the gut, mutations in the βPS subunit alone generate an effectively null integrin phenotype. Using a cell biological and genetic approach, it was shown that βυ forms heterodimers with αPS3. Thus, we have good evidence for the existence of four integrins in flies: αPS1βPS, αPS2βPS, αPS3βPS and αPS3βυ. Based on the high level of sequence similarity between αPS3-5, it seems likely that both βPS and βν can form heterodimers with αPS4 and αPS5. We suspect that αPS1 and αPS2 do not form heterodimers with βν, but such heterodimers have not been absolutely ruled out.
Since integrins must form a heterodimer in the endoplasmic reticulum to become transported to the cell surface, a fly that lacks the two β subunits should have no integrin function at all. Thus, by removing both maternal and zygotic contribution of these subunits, embryos that completely lack integrin function have been generated for the first time;19 a comparable experiment has yet to be done in worm or mouse. Similarly, it is now possible to produce clones of cells in the adult that completely lack integrin function. Surprisingly, certain processes such as the regulation of proliferation, that vertebrate studies have suggested are integrin dependent, occurred normally in the complete absence of integrins in the fly.
As one might expect, the loss of individual α subunits causes subsets of the phenotype of the complete loss of integrins. For some developmental events the loss of a single α subunit produces a phenotype that is just as strong but does not perturb other developmental processes mediated by other integrin heterodimers. In other cases there seems to be redundancy between the different α subunits, most commonly between αPS1 and αPS3, so that the loss of each individual subunit produces a mild phenotype and only the double mutant is as strong as the absence of the β subunits (see below for examples). The phenotypes fit with the expression patterns of the different α subunits. Thus, the analysis of the distribution and consequences of the loss of function of integrin subunits has shown that different integrin heterodimers have functions that are restricted to particular tissues, as elaborated below.
With a genetically tractable organism it can be valuable to generate a large variety of mutations in a given locus with the goal of identifying specific residues within the protein that have key functions. The use of a variety of genetic screening approaches to isolate mutant alleles in the gene encoding the αPS2 subunit gene identified distinct subfunctions of this protein that operated in different developmental events.31 An extensive analysis of strong mutations in the βPS subunit did not prove so informative, as the majority were frameshifts or stop codons,32 but an elegant genetic approach designed to identify mutants that only partially impaired βPS function produced a large variety of mutations of interest.33 Particularly surprising is the finding that mutations in some very highly conserved residues caused relatively weak phenotypes.
Genes Encoding Integrin-Associated Proteins
At this point in time, we can divide the genes encoding integrin-associated proteins into the following three groups: (i) those for which mutations exist and the mutant phenotypes are wholly or partially similar to those produced by mutations in the genes encoding integrins, suggesting that they function with integrins (Tables 2, 3), (ii) those for which mutations exist, but the phenotypes are distinct from integrin gene mutations, suggesting that they are not essential for integrin function (Table 4), and (iii) those for which mutations have yet to be isolated (Table 5). Some proteins associated with integrins in vertebrates do not have orthologues in the fly (Table 6).
Extracellular Matrix and Integrin Ligands
One of the complexities of integrins and their ligands relates to the question of “who comes first?”. Does the ECM get assembled first and then recruit and cluster integrins, or do the integrins lead to the assembly of the ECM.34 There is evidence to suggest both scenarios occur.
So far only three integrin ligands have been identified in Drosophila. Two of these are laminins. In flies there are two laminin α subunit genes, 1 β subunit and 1 γ subunit encoded in the genome. The α subunit gene encoded by the lamininA locus is most similar to vertebrate α3 and α5 laminin subunits, and contributes to basement membranes throughout the animal.35-37 The second α subunit is encoded by the wing blister gene, is equally similar to vertebrate α1 and α2, and has a much more restricted distribution.38 We shall refer to the two trimers formed by these two α subunits as lamininA and lamininW. LamininA has been shown to be a substrate for both αPS1βPS and αPS3βPS,24,27 but in general the distribution of the laminin A containing basement membranes is not altered in embryos lacking integrins.39 Recently, an extracellular matrix containing lamininA was identified that requires integrins for its assembly, which lies between two extraembryonic cell layers in the embryo, the amnioserosa and yolk cell,40 and is important for integrin function in the process of dorsal closure. This ECM does not seem to be a full basement membrane, as it is not visible as such by electron microscopy,89 and it lacks collagen IV.40 The Wing blister α subunit contains an RGD site, and a fragment containing this site is an effective ligand for αPS2βPS and αPS3βPS.27,42 Mutations in these two laminins cause phenotypes that mimic certain aspects of the integrin phenotypes, but they certainly do not completely recapitulate the integrin mutant phenotype, indicating that there must be other ligands. Other components of the basement membrane are also conserved in Drosophila, including collagen IV, perlecan, and nidogen/entactin, but the mutants available do not produce clear integrin-like phenotypes.43
The novel ECM protein Tiggrin highlights the potential problem in identifying the missing integrin ligands in Drosophila. It is a novel secreted protein, containing an RGD site,18 but so far a similar protein cannot be found in organisms other than Drosophila species, not even in other insects such as the mosquito(our observations). This suggests that completely novel proteins can adopt a function in the ECM, and therefore that predicting other ECM proteins from the genome sequence may prove difficult. Tiggrin was isolated from the media conditioned by the Drosophila Kc cell line, and functions as a ligand for αPS2βPS.18,44 The distribution of Tiggrin in the embryo is highly restricted, where it is present in stripes at the points where the muscles attach to the epidermis-the major site of integrin adhesion in the embryo (fig. 2). These muscle attachment sites contain the largest ECM in the fly, called the tendon matrix, which consists of an aggregate 1-2 μm across, containing fibrils of unknown composition (given the absence of genes encoding fibrillar collagen).45,39 Tiggrin can be captured from the extracellular fluid at these sites, as demonstrated by the normal distribution of Tiggrin when its site of synthesis was changed from the mesodermal cells to cells of the nervous system (tiggrinX; elav::Gal4/UAS::tiggrin).44 Surprisingly, integrins are not required for the capture of Tiggrin at the muscle attachment site, and the tendon matrix, as visualised by EM, still assembles. 18,39,45,46 A null tiggrin mutation causes a weakening of the attachment site, but does not fully mimic loss of integrins, indicating the presence of additional ligands.44 A protein M-spondin, related to vertebrate F-spondin is found at the muscle attachment site, but its mutation does not disrupt integrin adhesion.47 Mutation in the gene encoding the muscle-specific transcription factor Muscleblind causes a great reduction in the tendon matrix without altering tiggrin distribution or strongly affecting muscle attachment, further highlighting the redundancy of the components the tendon matrix.48
In summary, three integrin ECM ligands have been identified and genetic evidence suggests other unidentified ligands must exist. The combination of genetic redundancy and novelty in sequence of Tiggrin suggests that identification of additional ligands will most likely succeed by a proteomics approach.
Intracellular Integrin-Associated Proteins
A large number of intracellular proteins are enriched at sites of integrin adhesion in vertebrate cells.49 The Drosophila genome sequence has revealed that the majority of these proteins are conserved4 (Tables 2, 3, 4, 5). Mutations are available in many of them, making it possible to examine whether the absence of the protein produces a phenotype indicative of an essential role in contributing to integrin adhesion. Furthermore, this analysis will reveal whether the protein has functions independent of integrins, as revealed by phenotypes that are not shared with integrin mutants. One of the advantages of Drosophila is that different signaling pathways appear to have clearly independent functions,50 suggesting that some of the crosstalk between pathways observed in vertebrate cells in culture is not critical for the function of these pathways within the intact organism. To give an example, components of PI3 kinase and MAP kinase pathways have been implicated in integrin function, but mutations in PI3 kinase, MAP kinase and integrins produce clearly distinct phenotypes in the developing wing, with PI3 kinase regulating cell size,51 MAP kinase regulating proliferation,52 and integrins mediating adhesion between the two layers of the wing.103 Model organisms such as Drosophila can therefore be used to advantage to separate components into their core pathways.
The list of intracellular proteins confirmed via genetics to participate in integrin dependant adhesion in Drosophila is fairly small and includes the spectraplakin Short stop (shot), talin, integrin-linked-kinase (ILK), PINCH, and tensin (Table 2). Short stop appears to have a specific role in linking integrins to microtubules in the embryonic epidermis and the wing epithelia,13,14,86 and has other functions including axon extension (the failure in this led to the name) and oocyte specification which are independent of integrins.53,54,109 Talin appears to be required for almost all integrin functions, with the exception of cell migration mediated by αPS3βν19 and possibly signalling to regulate gene expression (for caveats see the section on signaling).15 PINCH is required for integrin function in strong adhesion and does affect one dynamic morphogenetic process, apparently through the regulation of signaling.96 ILK appears to be only required for integrin function in strong adhesion and not in more dynamic events, while a function for tensin is only apparent during adhesion in the wing, as the null mutant flies are viable and fertile with a wing blister phenotype.16,22,23
Several proteins including vinculin and focal adhesion kinase (FAK) are not required for integrin function; both can be removed without obvious detriment to the fly.20,55 This is unexpected because mutations in these genes in other organisms cause lethality.56,57 Overexpression of FAK causes defects that mimic loss of integrin function. This was previously thought to suggest that a dominant negative effect on endogenous FAK, but the fact that removing FAK does not cause this phenotype shows that this is not the explanation. Instead FAK must be acting to inhibit other proteins. In the muscles, overexpression of FAK causes the detachment of integrins from the ECM, suggesting that FAK downregulates integrin affinity for the ECM.20 Several other proteins have essential functions that are distinct from those mediated by integrins, including one of the two filamins (encoded by cheerio) and α-actinin58-61 while others, such as talin have additional functions that are apparently integrin independent (ref. 15 and unpublished observations).
The roles of a further set of proteins in integrin function have not been easy to resolve. For example, cells lacking Rho cannot divide, and thus it is not possible to test whether clones of cells completely lacking Rho have a failure in integrin adhesion. Weaker (hypomorphic) mutations in Rho can proliferate and have defects in cell polarity while integrin adhesion appears normal. Thus, one can only conclude that if Rho is required for integrin adhesion, this requires lower levels of the protein than for polarity, as is true for its role in cytokinesis.62 For other proteins, genetic redundancy combined with diverse roles preclude a simple conclusion. This is true for the Src kinases, where there is redundancy between the two Src kinases (Src42A and Src 64B), and the related Tec (Btk) kinase. Src64 and Tec have a role in regulating the actin cytoskeleton of the ring canals of the ovary, which is completely independent of integrins.63 Combinations of mutants in these kinases show defects in dorsal closure, which is an integrin-dependent process, but also involves many other pathways.64 The recent identification of mutations in Csk has confirmed its role as a negative regulator of the Src family of kinases in vivo but the presence of integrin-like phenotypes has not yet been examined.65,66
Several proteins of interest await genetic characterisation, including the second filamin, the two orthologues of the Kindler syndrome protein/UNC-112, p130Cas, Crk, and the LIM domain-containing proteins paxillin, zyxin and TES/testin.
Integrin Functions in Fly Development: Sculpting Tissues through Adhesion and Migration
The three most prominent defects in development caused by the loss of βPS integrin function in the fly are: detachment and rounding up of the embryonic muscles, a hole in the dorsal epidermis of the embryo, and blisters in the adult wing. It should be noted that null mutants in the gene encoding βPS cause embryonic lethality due to the first two defects and the wing blister phenotype of the adult fly can only be observed when chimeric flies are generated with patches of tissues that are mutant, or with unusual mutant alleles that particularly affect function in the adult. These prominent defects can be largely explained by the loss of an integrin adhesive function in keeping different layers of cells attached to each other through an intervening ECM, and it is still not clear whether integrin signalling is involved.
Integrin Signalling: Still Elusive
Although vertebrate cell culture has provided a wealth of literature supporting the existence of, requirements for and pathways mediating integrin signalling,67,68 studies in the fly have not been very forthcoming. We should clarify how we define integrin signalling. The assembly of a link between the integrin cytoplasmic tail and the cytoskeleton by a series of protein-protein interactions does not, in our view, constitute integrin signalling. However, if integrins trigger a cascade of protein modification, such as phosphorylation, that is required for the assembly of this link, then we would describe that as integrin signalling. Similarly, integrin regulation of gene expression must involve a signalling pathway that leads into the nucleus. There is an uncomfortable grey area that is harder to define: if the assembly of a link between integrins and the cytoskeleton requires conformational changes in each of the linker components, then does this constitute a signalling pathway? We leave that for the reader to decide.
Despite the identification of genes that are regulated by intracellular events downstream of the integrin βPS subunit cytoplasmic tail,69 so far of all the mutations tested, only mutations in the αPS1 subunit affect this signalling (refs. 15, 20, unpublished results). Part of the problem is that when one produces embryos completely lacking some of the candidate proteins, then the embryo does not develop far enough to assay the known target genes (this is true for both βPS and talin). In other cases integrin mediated adhesion is essential for the correct transmission of signals, for example in the transmission of an EGF signal from the muscles to the tendon cells70 where it regulates MAPK activity through the localisation of the EGFR ligand. Potential functions of integrin signalling synergising with TGFβ and EGF signalling have also been suggested during wing development, but the mechanisms are not yet clear.71,72
More extensive analysis of the flies lacking integrin function has revealed defects in migration, maintenance of epithelial polarity, dynamic regulation of synapses and regulation of gene expression. These developmental defects will be described in more depth below, but it is also worth mentioning some of the things that integrins do not do in fly development. Perhaps most importantly in the context of development, there is no evidence that integrins are involved in cell fate choice. This is in contrast to transmembrane receptors of several well known signalling pathways, including Notch and the receptors for a variety of secreted ligands: EGF, FGF, Wnt, Hedgehog, and TGF-β. The cell-cell adhesion molecules cadherins also present an interesting comparison. Cadherins do not appear to directly influence cell fate, but it may be more than a coincidence that the linker molecule β-catenin/Armadillo, which links the cytoplasmic domain of cadherins to the actin-binding protein α-catenin, is also a key component of Wnt signalling. In addition, cadherin-mediated adhesion plays a crucial role in the establishment of the body axes.73,74 In Drosophila, integrin-mediated adhesion is not essential for proliferation,19 so anchorage-dependent growth does not appear to operate in this organism. Integrins are not generally required for differentiation, as many cell type-specific proteins are produced normally in embryos lacking integrins. However, a screen through a small set of genes expressed at late stages of gut development identified two that were regulated by integrins.69 This suggested that integrins are part of a mechanism for coordinating differentiation and morphogenesis of a tissue.
Migration
Integrins are essential for some, but not all, cellular migrations during Drosophila development. In the embryo, integrins are essential for the movement of three groups of cells over a substrate formed by the visceral mesoderm. The primordial midgut endoderm arises in two populations, one at each end of the embryo, that undergo an epithelial to mesenchymal transition, crawl over the visceral mesoderm in an integrin-dependent fashion to meet in the middle and then reform an epithelium. The development of the endoderm is the only tissue where the integrin βν subunit plays a role, which appears to be redundant with βPS. In the absence of βPS alone, the migration is severely delayed, but eventually occurs,75,76 while in the absence of both β subunits, the cells never move.19 The trachea, the “lungs” of the fly, is a set of branching tubes that migrate to reach a variety of target tissues. One of the branches in every segment - the branch that migrates over the visceral mesoderm to reach the endoderm- requires integrins for its migration.77 Similarly, the tip of the salivary gland, which is an invaginated epithelial sac, migrates in a posterior direction over the visceral mesoderm using integrins.78 In each case, integrins are required both in the visceral mesoderm (αPS2βPS) and in the migrating tissue, which employs one or both of αPS1βPS and αPS3βPS. The current model is that αPS2βPS is required in the visceral mesoderm to assemble an ECM that serves as a substrate for the migration of these different tissues. The composition of this predicted ECM is not known. A further example of integrin-dependent migration is when a portion of the extraembryonic epithelial layer called the amnioserosa extends over the epidermis during the process of germ band retraction (fig. 4). This involves αPS3, αPS1 and the second laminin isoform, lamininW.27
These cases show that integrins play a crucial role in the positioning of tissues within the embryo. In the absence of integrins, the cells that lead these movements lack their characteristic lamellipodial and filopodial extensions.27,76,78 Integrins are not required for all cell movements in the embryo. As mentioned, other tracheal branches reach their target without integrins, and the germ cells move normally in the absence of integrins from their original position outside the embryo into the gonad. Thus, the mechanism of cell movement in development can be separated from the use of particular receptors to specify traction.
Integrin Function in Muscle Attachment; A Focal Adhesion-Like Structure within the Embryo
The role of integrins in muscle attachment has proven to be a useful model for integrin function in stable adhesion within the embryo. The eponymous “rounded up” muscle phenotype of mutations in the βPS integrin subunit (encoded by the gene myospheroid,7,79) is easily visualised. The major sites of integrin accumulation in the developing embryo are muscle attachments (see fig. 2). Integrins are required for the maintenance of these structures, not their formation. The muscle attachment sites are points of contact between the ends of the muscles and specialised epidermal cells, the tendon cells. The tendon cells are specified independently of the existence of the muscles, and appear to send signals to attract the muscles.80 The muscle founder cells and myoblasts undergo fusion simultaneously with their extension toward and adhesion to the tendon cells. Integrins do not to contribute to myoblast fusion in Drosophila, as they can do in vertebrates.81 After the initial contact of muscles with tendon cells, the tendon matrix builds up, particularly at sites where muscles also make end-end attachment to each other. The contact of the muscle with the tendon cell sends signals to support the further differentiation of the tendon cell. The transmission of the signal requires integrins, but this is due to their role in keeping the muscle in contact with the tendon cell, rather than being part of the signalling pathway.70 Shortly after the muscles are attached they begin to contract, which they do prior to innervation by the nervous system or the formation of striations. As the muscles develop further the contractions build in strength, and eventually the resultant larva hatches and crawls away.
This gradual increase in the strength of muscle contraction provides a useful measure of the strength of adhesion that remains in the absence of a particular component. For example, in an embryo completely lacking integrins, the muscles detach soon after contractions begin.7,19,75,82 If just the αPS2βPS integrin, the only known integrin in the muscles, is removed, the muscles detach slightly later,39,82 presumably because the αPS1βPS integrin in the tendon cell holds the structure together a little longer; embryos lacking both αPS1 and αPS2 have equivalent defects as embryos lacking βPS.39 The loss of talin results in the muscles detaching at the same developmental stage as in the absence of integrins,15 while the loss of ILK results in a detachment some 8 hours later23 (out of the 22 hrs of embryogenesis). This later detachment looks different, with less rounding up of the muscles. Closer examination revealed that whereas the early defect results from the muscles detaching from the tendon matrix,15 in the later cases the point of detachment occurs first inside the cell, separating the integrins from the cytoskeleton, resulting in an “actin detachment” rather than muscle detachment23 (fig. 3). This suggests that there is some redundancy between ILK and other components, such that the structure is only weakened in the absence of ILK alone, so that stronger contractions can be withstood before the attachment structure fails. Tensin is tightly localised to the muscle attachment, as is phosphorylated FAK, but removal of these proteins does not cause visible defects in the muscles.20,22
The final muscle attachment structure consists of the ends of the contractile machinery connected via integrins to the tendon matrix, which is in turn bound by integrins on the basal surface of the tendon cells. In these cells microtubule bundles connect the basal integrin junctions to junctions on the apical surface of the cell that binds to the exoskeleton or cuticle. In this way, muscle contractions achieve movement of the exoskeleton. The cuticle can be considered an apical ECM, but the receptors for this ECM are not integrins and have yet to be identified. Components of the apical ECM were identified as the wing blister mutants dumpy, piopio and papillote.83-85 Short stop plays a key role in connecting the microtubules to the integrin junctions in tendon cells (fig. 2).14,86
Integrin Functions in Dynamic Epithelial Morphogenesis
Integrins have complex functions during the processes of germband retraction and dorsal closure. In these normal developmental events, the embryo retracts back from the extension that occurred during gastrulation and the epidermis extends dorsally to cover the “hole” in the surface of the embryo that has been covered by the extraembryonic epithelial layer called the amnioserosa (fig. 4). At the end of dorsal closure the amnioserosa invaginates and undergoes apoptosis, while the dorsal edges of the epidermis seal up along the dorsal midline. The retraction of the embryo and extension of the epidermis dorsally are driven by cell shape changes; there is no cell proliferation in the epidermis at this stage. Dorsal closure bears similarities in both appearance and molecular mechanism with embryonic wound healing.41
Recently a third player in these epithelial transformations was identified, in addition to the epidermis and the amnioserosa, which is the giant yolk cell that lies in the centre of the embryo. 40 This cell is a product of the unusual early development of insect embryos. After fertilisation, rather than undergoing cell cleavages Drosophila undergoes 14 rounds of nuclear division, without any cell division. The nuclei then migrate to the surface of the egg, and the plasma membrane invaginates around each nucleus. When the invaginating membranes meet underneath each nucleus this generates a monolayer of blastoderm cells and a single large internal cell, the yolk cell, which contains a few nuclei.
Integrins have been known to contribute to germ band retraction and dorsal closure for some time, but how they contribute has not been clear, and recent work has suggested that they have multiple roles. At the end of embryogenesis, many embryos lacking integrin function contain a dorsal hole.7 The contraction of the amnioserosa is abnormal, and tears appear within the amnioserosa.40 If the integrins are also removed maternally, a more severe defect is observed were germ band retraction fails.75 All three layers, epidermis, yolk cell and amnioserosa, express integrins (fig. 4).40
Recent work using live imaging to examine germband retraction has shown that both the amnioserosa and the yolk cell are very active cells.27,87 One hypothesis has the amnioserosa sending membrane ruffles over the epidermis to drive germband retraction via an integrin-dependent migration event.27 Similarly, live analysis of dorsal closure showed that the contraction of the amnioserosa, which occurs simultaneously with the extension of the epidermis, was delayed in embryos lacking integrins.88 This was suggested to be due to a defect in the zippering up of the dorsal edges of the epidermis, the process where the filopodia of the epidermal cells at the interface with the amnioserosa appear to reach over the amnioserosa and make contact with the opposing edge of the epiderms, and then by contraction, draw the two edges together.89 Our own observations provide an alternative explanation, which is that the integrin defect (and hence requirement) preceeds the zippering, in that the amnioserosa cells fail to contract sufficiently to allow the edges of the epidermis to come within zippering range.
The integrin dependant contraction of the amnioserosa is mediated in part by facilitating adhesion between the amnioserosa and the yolk cell via an intervening ECM.40 This ECM contains the basement membrane component lamininA, and in contrast to the general assembly of basement membranes, the assembly of lamininA between the amnioserosa and yolk cell is integrin dependent. Loss of integrin mediated adhesion by mutations in the integrin subunits αPS3 or βPS, or the lamininA α subunit resulted in a separation between the yolk cell and the amnioserosa and a delay in contraction and closure. Examination of the mutant phenotypes also suggested that the yolk cell serves as a cellular substrate for the contraction of the amnioserosa.
These results showed that the general feature of integrin function in development, of mediating adhesion between cell layers, is also employed during dynamic epithelial rearrangements. However, the loss of adhesion between the amnioserosa and the yolk cell is not in itself sufficient to cause a dorsal hole; in contrast to removing integrins, the lamininA mutant embryos do not end up with a dorsal hole. This indicates that integrins have additional roles that are independent of laminin. One such role appears to be mediating adhesion between the lateral sides of the amnioserosa cell to each other and to the leading edge of the dorsal epidermis.40 It is not clear yet how direct integrin function is in mediating this apparent cell-cell adhesion between the lateral sides of cells or what ligand is used.
Integrin Functions in the Epithelia
Further examples of integrin-dependent adhesion of epithelial layers are in the adult fly. The best characterised is the adhesion between the two surfaces of the developing wing; loss of this adhesion leads to a wing blister.103,108,115 This is reminiscent of the human skin diseases epidermolysis bullosa and bullous pemphigoid. Since the former is caused by loss of the hemidesmosome integrin α6β4, which is absent in flies, one might not think that the fly would provide a good model for this disease. However, the wing blister mutant short stop/kakapo turns out to encode a fly orthologue of the protein bullous pemphigoid antigen 1, BPAG1, and more recently the human orthologue of a protein required for integrin function in C. elegans has been shown to be linked to the skin blistering disease Kindler's syndrome.14,90,116 This suggests that an understanding of basic mechanisms of integrin function in invertebrates may well provide a useful model for these human epithelial diseases.
The wing arises from an epithelial sac, the imaginal disc, that grows during larval life. When the larva pupariates, the larval epidermis degenerates and a new epidermis is produced from the imaginal discs and histoblasts. At the start of pupal development the wing imaginal disc evaginates, generating a two-layered epithelial wing. Integrins are essential to hold these two layers together. Certainly part of the function is to generate strong adhesive junctions, but signaling may also be involved.117 The integrins on the two sides of the wing are different; αPS1βPS is on the dorsal side, while αPS2βPS is on the ventral side.91 The laminin α subunit encoded by the gene wing blister is essential, while mutations in the other laminin α subunit or tiggrin do not give a wing blister phenotype.38,44,118 Integrins become strongly localised into tight discs where the basal side of each epithelial cell attaches to a cell on the other surface of the wing.92 A similar function for integrins is in the attachment of the retinal cells to the underlying basment membrane in the developing eye.93
Thus, the defects caused by the absence of integrins in the epithelial layers have revealed that the primary role of integrins is to hold cell layers together. However, other potential roles for integrins in the epithelia have been explored. While integrins do not seem to mediate anchorage dependant growth, there is correlative evidence for a role for integrins in anchorage dependent cell survival. Premature degeneration of the amnioserosa seen in a small proportion of integrin mutants has been attributed to the loss of adhesion between the yolk and the amnioserosa.87 Genetic studies have uncovered interactions between integrins and ecdysteroid hormones that mediate many metamorphic cell death events during fly development that include dorsal closure and the shaping of the wing.94,95 It has also been suggested that tensin transduces integrin signals to the JNK pathway to mediate apoptosis in the wing. It seems more likely that tensin acts in parallel to the JNK pathway and that the loss of tensin uncovers an enhanced sensitivity to the reduction of JNK signals.21 More recent studies have also suggested a role for PINCH in the regulation of JNK activity during dorsal closure.96
Vertebrate studies have provided conflicting views on the requirements for the establishment of polarity in epithelia, with particular reference to the role of integrin dependant adhesion to the substrate. Studies on the development of the follicular epithelium in Drosophila, a secondary epithelium fomed by a mesenchymal-epithelial transition have shown that while contact with the basement membrane is sufficient to exclude apicolateral membrane markers from the “basal” membrane domain and prevent the lateral spread of apicolateral components in the absence of apical cues, it is insufficient to fully polarise the epithelium.97 On the basis of the analysis of secondary epithelia lacking all integrin function it has been suggested that integrins are not required for establishment of polarity. The aberrant distribution of membrane markers in the cells that have lost their attachment to the basement membrane and rounded up has lead to the suggestion that integrins are required for the maintenance of polarity.19 It is not been established whether the cells round up because of a loss of polarity. However, secondary epithelia are atypical, since some of them, such as the midgut epithelium in which integrin function has been examined,19 are not completely polarised until long after their establishment. It is therefore still plausible that the establishment of polarity is very robust and that integrins play a redundant role. This would be consistent with the finding that many molecules that contribute to polarity act in parallel and result in only modest defects when absent.98
Integrin Function in the Nervous System
A modest defect in axon pathfinding has been described for integrin mutants,99 which contrasts with the severe phenotype caused by loss of Short stop.100 Although the formation of the synapses is not itself affected, changes in the levels of integrins results in dramatic alterations in their growth and sprouting.29,39,101 The potential to regulate functional synaptic plasticity processes may account for the role of integrins in the formation of memory.29 This function was uncovered through the analysis of hypomorphic mutants in βPS and αPS3 subunit. Studies have revealed that integrin effects on the synapses may be mediated through its association with discs large and the resulting modulation of Fasciclin II through CamII kinase activity.102
Complementary and Two-Sided Requirements
In all the above-mentioned integrin-dependent developmental events, βPS containing integrins are required on both faces to mediate adhesion or facilitate migration. This is best inferred from the deleterious effects on adhesion of disrupting integrin function on one side (e.g., a mutant clone on one side of the wing, or driving the expression of a dominant negative integrin construct in just the yolk or the amnioserosa during dorsal closure,40,103). A recurring theme in the deployment of Drosophila integrins is therefore that they mediate adhesion between cell layers rather than of a cell layer to a basement membrane (muscles and tendon cell at muscle attachment sites, yolk and amnioserosa during dorsal closure, dorsal and ventral epithelial layers in the wing).
The α subunits often demonstrate complementarity of distribution in the apposing layers (but not necessarily mutual exclusion, with more than one α expressed in a given layer in some instances) and show requirements predominantly in that layer. This is evident from the altered distribution and poor transport of the βPS subunit in the absence of the α subunit exclusively in that layer.104 Overexpression of the wrong heterodimer in the wing epithelial layer produces a blister analogous to that seen in the loss of function clones. The reason for this is not yet clear, but genetic studies have suggested that it is a gain of function phenotype.71,117 One mechanism that may explain complementarity is that adhesion is facilitated by the bridging of a ligand that binds to sites in two α subunits. It has indeed been shown that the specificity that each α subunit brings to integrin function resides in the extracellular domain; swapping the cytoplasmic domains between αPS1 and αPS2 had no detectable effects on their function.104
Insights into the Mechanism of Integrin Function
Establishing a Hierarchy: Not All Integrin-Associated Proteins Are Equally Important
As progress is made toward isolating mutations in every protein required for integrin function, the mutations isolated so far can be used to address two fundamental problems of integrin biology relating to the diversity and complexity of the intracellular complexes recruited by integrins. Our discussion will focus on the proteins characterised both molecularly and genetically in flies: talin, ILK, PINCH, tensin and Short stop. First a brief mention about the structure of these proteins. Talin is large, (>2500 residues) and has two well defined domains, an N-terminal FERM domain, which generally seems to be used for interacting with membrane proteins, and a C-terminal I/LWEQ domain, shared with the yeast actin binding protein Sla2.105 It has been found to bind to several proteins of interest, including the integrin β subunit cytoplasmic tail, FAK, vinculin, PI4-5 kinase, and actin. Integrin-linked-kinase (ILK) has an N-terminus containing ankyrin repeats and a kinase domain that is diverged at several residues normally highly conserved in active kinases, making it unclear whether it has substantial kinase activity.106,107 The ankyrin repeats bind to PINCH, and this has been confirmed in vertebrate cells, worms and flies. The kinase domain binds to integrins (but this could not be verified in flies or worms), the protein parvin (also known as actopaxin, affixin, PAT-6), paxillin, and to UNC-112. PINCH consists of 5 LIM domains, and binds to ILK and the adaptor Nck2. The former interaction has been confirmed in flies and worms, but not the latter. The fly tensin appears to have lost the N-terminal phosphatase domain, although it retains actin binding activity. At the C-terminus, tensin contains two phosphotryosine binding domains, SH2 and PTB.22 Short stop is a giant protein of the spectrin and plakin families, containing an N-terminal actin binding domain and a C-terminal microtubule binding domain.109
An outstanding question in integrin biology is how the same integrin performs different functions at different times and places during development. For example, we wish to understand what changes an integrin from one that mediates migration to one that forms stable adhesive junctions. The simplest explanation is that the differences result from a change in the intracellular proteins available to interact with integrins and therefore it seems likely that the intracellular machinery downstream of integrins is different for the two integrin functions. The genetics of the integrin-associated proteins has supported this view, by showing that the components are only essential for some integrin functions.
Talin is required for almost all integrin functions, including migration, muscle attachment, germband retraction, dorsal closure and wing adhesion, and so can be viewed as part of the “core” of integrin intracellular machinery. This view has been supported by recent work in vertebrate cells,110 despite the fact that the analysis is hampered by the existence of two talin homologues in mouse and in humans. For example, the knockout of the single fly talin gene results in the inability of the integrins to form to focal adhesion-like structures in the wing primordium,15 while mouse cells lacking talin1 are still able to form focal adhesions, although at a reduced rate and delayed in time.111,112 There are two known integrin-dependent processes in the fly that do not require talin function: signalling to regulate gene expression (or FAK phosphorylation in the mouse,112) and βν-dependent migration, as previously discussed. The talin mutant phenotype in the midgut is the same as βPS, with a delay and eventual recovery of migration and unlike the double β subunit mutation in which migration is blocked.19 These results show that the βν-mediated migration, which can only be observed as the completion of migration in the absence of βPS, does not use talin. This is further supported by the fact that the well characterised binding site for talin on β subunit cytoplasmic domains is not conserved in βν. The protein that substitutes for talin in βν-mediated migration is not known.
Other integrin-associated proteins characterised to date have even more limited functions. Mutations in the gene encoding the interacting protein ILK shows only a subset of the defects found in animals lacking integrins or talin, namely defects in muscle attachment and wing adhesion23 while PINCH mutants have dorsal closure defects in addition to these.16,96 Flies lacking tensin only have a wing blister phenotype, which is distinct from the blisters produced by other mutations in that it is caused by the normal mechanical abrasion produced by stroking the wings with the legs to help flatten the wing.22 Thus, it is possible to rescue the tensin mutant wing blister phenotype by immobilising the legs of the mutant flies. The distribution of these proteins does not fully correlate with function: ILK colocalises with integrins and talin during dorsal closure, but is not required there, while tensin is neither associated with these proteins during dorsal closure nor required there, but it is found with them at the muscle ends even though it is not required. It is possible that the activity of these proteins is regulated differently for different functions. The distribution of Short stop more accurately reflects its function. In the embryo, Short stop is only expressed in the tendon cells, not the muscles, and its function with integrins appears to be limited to cases where integrin junctions are connected to microtubules, a junction that appears to be analogous to the hemidesmosome of vertebrate epithelial cells.13,14 Short stop has roles in the development of the trachea, nervous system and oocyte that are independent of integrins.54,109 In the converse situation, several proteins are known to be required for dorsal closure but not other integrin events, e.g., jun kinase kinase.121 Proteins that are specifically required for integrin-dependent migration have not yet been identified.
The second related problem is to discover why the intracellular machinery connected to integrins is so complex; over 60 proteins have been documented that may play a role in this connection.49 Several of them have the potential to bind directly to integrins and actin. Even if we take the rigorous line of requiring mutations to give phenotypes similar to integrin mutants, we still have several intracellular proteins required, and there are many remaining to be tested. By comparing the detailed cell biology of the different mutant phenotypes it has been possible to build up a hierarchy of function and obtain clues towards the different roles of the proteins. The large attachment sites of embryonic muscle have been most amenable for this analysis, due to the ease of antibody staining compared to the attachments within the pupal wing. It has been informative to document the timing of the defect and the position of the failure in the ECM-integrin/plasma membrane-actin cytoskeleton link (fig. 3). Thus, in embryos lacking integrins the detachment occurs right after the muscles become contractile, and occurs between the ECM and plasma membrane, with some plasma membrane-actin connection still formed.7,23,39,45 In the absence of talin, the detachment occurs at the same early stage, with a detachment between integrins and actin. Muscles can be seen without any integrin on the cell surface, and it appears as if the integrin has been left behind, still attached to the ECM. Whether the integrins are pulled out of the plasma membrane or whether a small “vesicle” of plasma membrane and cytoskeleton remains associated is not known. In embryos lacking either ILK and PINCH the detachment occurs at the very end of embryogenesis, about 8 hours later than in the absence of integrins or talin.16,23 Our hypothesis is that this indicates that the connection between integrins and actin has been weakened, rather than eliminated, and it takes building up the muscle structure and the production of stronger contractions to generate a force strong enough to break the connection. The failure in the connection also occurs at a different point, between the integrins/plasma membrane and actin cytoskeleton, an “actin detachment” phenotype rather than “muscle detachment”.23 Thus, components of the integrin complex function at different levels in the link between the membrane and the cytoskeleton.
Examination of the distribution of the components in the different mutants also supports this hierarchical view. Integrins are required for the recruitment of talin, PINCH and tensin, and reciprocally none of the intracellular proteins are required for the localisation of integrins to the ends of the muscles.15,16,22 In summary, the chronology of the onset of the phenotypes and the dependence of components on others for their localisation at the muscle attachment sites allows the ordering of the molecules into a hierarchy: integrins → talin→ ILK → tensin → PINCH, that is not strictly linear. This strengthens the notion that each component performs a crucial function, separable in time and dependant on interactions that precede it. Some are crucial for the establishment of the junction and show early phenotypes (integrin and talin). Others (ILK complex) play crucial roles linking the junctions, once established, to the actin cytoskeleton23 and still others (e.g., tensin) have been hypothesised to strengthen this link.22
Structure function analyses facilitated by engineering mutations and deletions in the genomic context have also provided insights into how the molecules function in the context of the whole organism. Such studies have revealed that ILK functions as a scaffold for protein-protein interactions and not as a kinase.23 In worms as in flies, mutations that are predicted to eliminate kinase activity do not impair function while in the mouse the results of similar experiments are less clear cut.113,114,120 Similar studies on tensin have identified a novel mechanism of recruitment implicating a crucial role for the PTB domain in targeting the protein to the muscle end where it can interact with phosphoproteins through its SH2 domain.22
Future Prospects
We anticipate that the analysis of all integrins and associated proteins in Drosophila will shed light on the hierarchical organisation and molecular architecture of the “core” complex that mediates stable adhesion. A detailed analysis of morphogenetic movements that involve “dynamic adhesion” in mutants in integrin associated proteins should help understand how different the composition of the complex is from the one that mediates stable adhesion. As mentioned earlier in this chapter, expression does not imply an essential function and it is likely that certain components are differentially activated in the two types of adhesion. How activity is modulated will be important to understand. From a cell biological standpoint, it will be interesting to understand the molecular interactions that facilitate the translation of integrin-substrate interactions into changes in cell morphology and behaviour.
Acknowledgements
We thank members of the Brown lab ‘Writing Workshop’ for comments and suggestions.
References
- 1.
- Bokel C, Brown NH. Integrins in development: Moving on, responding to, and sticking to the extracellular matrix. Dev Cell. 2002;3(3):311–321. [PubMed: 12361595]
- 2.
- Brower DL. Platelets with wings: The maturation of Drosophila integrin biology. Curr Opin Cell Biol. 2003;15(5):607–613. [PubMed: 14519396]
- 3.
- Brown NH, Gregory SL, Martin-Bermudo MD. Integrins as mediators of morphogenesis in Drosophila. Dev Biol. 2000;223(1):1–16. [PubMed: 10864456]
- 4.
- Hynes RO, Zhao Q. The evolution of cell adhesion. J Cell Biol. 2000;150(2):F89–96. [PubMed: 10908592]
- 5.
- Wilcox M, Brower DL, Smith RJ. A position-specific cell surface antigen in the Drososphila wing imaginal disc. Cell. 1981;25:159–164. [PubMed: 6791831]
- 6.
- Weinstein A. Coincidence of crossing over in Drosophila melanogaster (Amelophila). Genetics. 1918;3:135–172. [PMC free article: PMC1200433] [PubMed: 17245901]
- 7.
- Wright TRF. The phenogenetics of the embryonic mutant, lethal myospheroid, in Drosophila melanogaster. Development. 1960;118:845–858. [PubMed: 13786827]
- 8.
- Woodruff RC, Ashburner M. The genetics of a small autosomal region of Drosophila melanogaster containing the structural gene for alcohol dehydrogenase. I. Characterization of deficiencies and mapping of ADH and visible mutations. Genetics. 1979;92(1):117–132. [PMC free article: PMC1213935] [PubMed: 115743]
- 9.
- Glass HB. New mutants report. D.I.S. 1937;2:7–8.
- 10.
- Brower DL, Bunch TA, Mukai L. et al. Nonequivalent requirements for PS1 and PS2 integrin at cell attachments in Drosophila: Genetic analysis of the alpha PS1 integrin subunit. Development. 1995;121(5):1311–1320. [PubMed: 7789263]
- 11.
- Prout M, Damania Z, Soong J. et al. Autosomal mutations affecting adhesion between wing surfaces in Drosophila melanogaster. Genetics. 1997;146(1):275–285. [PMC free article: PMC1207943] [PubMed: 9136017]
- 12.
- Walsh EP, Brown NH. A screen to identify Drosophila genes required for integrin-mediated adhesion. Genetics. 1998;150(2):791–805. [PMC free article: PMC1460349] [PubMed: 9755209]
- 13.
- Strumpf D, Volk T. Kakapo, a novel cytoskeletal-associated protein is essential for the restricted localization of the neuregulin-like factor, vein, at the muscle-tendon junction site. J Cell Biol. 1998;143(5):1259–1270. [PMC free article: PMC2133081] [PubMed: 9832554]
- 14.
- Gregory SL, Brown NH. kakapo, a gene required for adhesion between and within cell layers in Drosophila, encodes a large cytoskeletal linker protein related to plectin and dystrophin. J Cell Biol. 1998;143(5):1271–1282. [PMC free article: PMC2133084] [PubMed: 9832555]
- 15.
- Brown NH, Gregory SL, Rickoll WL. et al. Talin is essential for integrin function in Drosophila. Dev Cell. 2002;3(4):569–579. [PubMed: 12408808]
- 16.
- Clark KA, McGrail M, Beckerle MC. Analysis of PINCH function in Drosophila demonstrates its requirement in integrin-dependent cellular processes. Development. 2003;130(12):2611–2621. [PubMed: 12736206]
- 17.
- Bellen HJ, Levis RW, Liao G. et al. The BDGP gene disruption project: Single transposon insertions associated with 40% of Drosophila genes. Genetics. 2004;167(2):761–781. [PMC free article: PMC1470905] [PubMed: 15238527]
- 18.
- Fogerty FJ, Fessler LI, Bunch TA. et al. Tiggrin, a novel Drosophila extracellular matrix protein that functions as a ligand for Drosophila alpha PS2 beta PS integrins. Development. 1994;120(7):1747–1758. [PubMed: 7924982]
- 19.
- Devenport D, Brown NH. Morphogenesis in the absence of integrins: Mutation of both Drosophila beta subunits prevents midgut migration. Development. 2004;131(21):5405–5415. [PubMed: 15469969]
- 20.
- Grabbe C, Zervas CG, Hunter T. et al. Focal adhesion kinase is not required for integrin function or viability in Drosophila. Development. 2004;131(23):5795–5805. [PubMed: 15525665]
- 21.
- Lee SB, Cho KS, Kim E. et al. blistery encodes Drosophila tensin protein and interacts with integrin and the JNK signaling pathway during wing development. Development. 2003;130(17):4001–4010. [PubMed: 12874122]
- 22.
- Torgler CN, Narasimha M, Knox AL. et al. Tensin stabilizes integrin adhesive contacts in Drosophila. Dev Cell. 2004;6(3):357–369. [PubMed: 15030759]
- 23.
- Zervas CG, Gregory SL, Brown NH. Drosophila integrin-linked kinase is required at sites of integrin adhesion to link the cytoskeleton to the plasma membrane. J Cell Biol. 2001;152(5):1007–1018. [PMC free article: PMC2198807] [PubMed: 11238456]
- 24.
- Gotwals PJ, Fessler LI, Wehrli M. et al. Drosophila PS1 integrin is a laminin receptor and differs in ligand specificity from PS2. Proc Natl Acad Sci USA. 1994;91(24):11447–11451. [PMC free article: PMC45248] [PubMed: 7972082]
- 25.
- Bunch TA, Brower DL. Drosophila PS2 integrin mediates RGD-dependent cell-matrix interactions. Development. 1992;116(1):239–247. [PubMed: 1282860]
- 26.
- Stark KA, Yee GH, Roote CE. et al. A novel alpha integrin subunit associates with betaPS and functions in tissue morphogenesis and movement during Drosophila development. Development. 1997;124(22):4583–4594. [PubMed: 9409675]
- 27.
- Schock F, Perrimon N. Retraction of the Drosophila germ band requires cell-matrix interaction. Genes Dev. 2003;17(5):597–602. [PMC free article: PMC196012] [PubMed: 12629042]
- 28.
- Nusslein-Volhard C, Wieschaus E, Kluding H. Mutations affecting the pattern of the larval cuticle in Drosophila melanogaster I. Zygotic loci on the second chromosome Roux's Arch Dev Biol|1984; 193267–2892. [PubMed: 28305337]
- 29.
- Grotewiel MS, Beck CD, Wu KH. et al. Integrin-mediated short-term memory in Drosophila. Nature. 1998;391(6666):455–460. [PubMed: 9461212]
- 30.
- Yee GH, Hynes RO. A novel, tissue-specific integrin subunit, beta nu, expressed in the midgut of Drosophila melanogaster. Development. 1993;118(3):845–858. [PubMed: 8076521]
- 31.
- Bloor JW, Brown NH. Genetic analysis of the Drosophila alphaPS2 integrin subunit reveals discrete adhesive, morphogenetic and sarcomeric functions. Genetics. 1998;148(3):1127–1142. [PMC free article: PMC1460035] [PubMed: 9539430]
- 32.
- Jannuzi AL, Bunch TA, Brabant MC. et al. Disruption of C-terminal cytoplasmic domain of betaPS integrin subunit has dominant negative properties in developing Drosophila. Mol Biol Cell. 2002;13(4):1352–1365. [PMC free article: PMC102274] [PubMed: 11950944]
- 33.
- Jannuzi AL, Bunch TA, West RF. et al. Identification of integrin beta subunit mutations that alter heterodimer function in situ. Mol Biol Cell. 2004;15(8):3829–3840. [PMC free article: PMC491840] [PubMed: 15194810]
- 34.
- Brown NH. An integrin chicken and egg problem: Which comes first, the extracellular matrix or the cytoskeleton? Curr Opin Cell Biol. 2000;12(5):629–633. [PubMed: 10978900]
- 35.
- Montell DJ, Goodman CS. Drosophila laminin: Sequence of B2 subunit and expression of all three subunits during embryogenesis. J Cell Biol. 1989;109(5):2441–2453. [PMC free article: PMC2115843] [PubMed: 2808533]
- 36.
- Kusche-Gullberg M, Garrison K, MacKrell AJ. et al. Laminin A chain: Expression during Drosophila development and genomic sequence. EMBO J. 1992;11(12):4519–4527. [PMC free article: PMC557027] [PubMed: 1425586]
- 37.
- Fessler LI, Campbell AG, Duncan KG. et al. Drosophila laminin: Characterization and localization. J Cell Biol. 1987;105(5):2383–2391. [PMC free article: PMC2114870] [PubMed: 3119602]
- 38.
- Martin D, Zusman S, Li X. et al. Wing blister, a new Drosophila laminin alpha chain required for cell adhesion and migration during embryonic and imaginal development. J Cell Biol. 1999;145(1):191–201. [PMC free article: PMC2148222] [PubMed: 10189378]
- 39.
- Prokop A, Martin-Bermudo MD, Bate M. et al. Absence of PS integrins or laminin A affects extracellular adhesion, but not intracellular assembly, of hemiadherens and neuromuscular junctions in Drosophila embryos. Dev Biol. 1998;196(1):58–76. [PubMed: 9527881]
- 40.
- Narasimha M, Brown NH. Novel functions for integrins in epithelial morphogenesis. Curr Biol. 2004;14(5):381–385. [PubMed: 15028212]
- 41.
- Jacinto A, Woolner S, Martin P. Dynamic analysis of dorsal closure in Drosophila: From genetics to cell biology. Dev Cell. 2002;3(1):9–19. [PubMed: 12110163]
- 42.
- Zavortink M, Bunch TA, Brower DL. Functional properties of alternatively spliced forms of the Drosophila PS2 integrin alpha subunit. Cell Adhes Commun. 1993;1(3):251–264. [PubMed: 7521756]
- 43.
- Voigt A, Pflanz R, Schafer U. et al. Perlecan participates in proliferation activation of quiescent Drosophila neuroblasts. Dev Dyn. 2002;224(4):403–412. [PubMed: 12203732]
- 44.
- Bunch TA, Graner MW, Fessler LI. et al. The PS2 integrin ligand tiggrin is required for proper muscle function in Drosophila. Development. 1998;125(9):1679–1689. [PubMed: 9521906]
- 45.
- Newman JrSM, Wright TRF. A histological and ultrastructural analysis of developmental defects produced by the mutation, lethal(1) myospheroid, in Drosophila melanogaster. Developmental Biology. 1981;86:393–402. [PubMed: 6793430]
- 46.
- Martin-Bermudo MD, Brown NH. The localized assembly of extracellular matrix integrin ligands requires cell-cell contact. J Cell Sci. 2000;113(Pt 21):3715–3723. [PubMed: 11034900]
- 47.
- Umemiya T, Takeichi M, Nose A. M-spondin, a novel ECM protein highly homologous to vertebrate F-spondin, is localized at the muscle attachment sites in the Drosophila embryo. Dev Biol. 1997;186(2):165–176. [PubMed: 9205137]
- 48.
- Artero R, Prokop A, Paricio N. et al. The muscleblind gene participates in the organization of Z-bands and epidermal attachments of Drosophila muscles and is regulated by Dmef2. Dev Biol. 1998;195(2):131–143. [PubMed: 9520330]
- 49.
- Zamir E, Geiger B. Molecular complexity and dynamics of cell-matrix adhesions. J Cell Sci. 2001;114(Pt 20):3583–3590. [PubMed: 11707510]
- 50.
- Noselli S, Perrimon N. Signal transduction. Are there close encounters between signaling pathways? Science. 2000;290(5489):68–69. [PubMed: 11183153]
- 51.
- Weinkove D, Neufeld TP, Twardzik T. et al. Regulation of imaginal disc cell size, cell number and organ size by Drosophila class I(A) phosphoinositide 3-kinase and its adaptor. Curr Biol. 1999;9(18):1019–1029. [PubMed: 10508611]
- 52.
- Diaz-Benjumea FJ, Hafen E. The sevenless signalling cassette mediates Drosophila EGF receptor function during epidermal development. Development. 1994;120(3):569–578. [PubMed: 8162856]
- 53.
- Lee S, Kolodziej PA. Short Stop provides an essential link between F-actin and microtubules during axon extension. Development. 2002;129(5):1195–1204. [PubMed: 11874915]
- 54.
- Roper K, Brown NH. A spectraplakin is enriched on the fusome and organizes microtubules during oocyte specification in Drosophila. Curr Biol. 2004;14(2):99–110. [PubMed: 14738730]
- 55.
- Alatortsev VE, Kramerova IA, Frolov MV. et al. Vinculin gene is nonessential in Drosophila melanogaster. FEBS Lett. 1997;413(2):197–201. [PubMed: 9280281]
- 56.
- Xu W, Baribault H, Adamson ED. Vinculin knockout results in heart and brain defects during embryonic development. Development. 1998;125(2):327–337. [PubMed: 9486805]
- 57.
- Ilic D, Furuta Y, Kanazawa S. et al. Reduced cell motility and enhanced focal adhesion contact formation in cells from FAK-deficient mice. Nature. 1995;377(6549):539–544. [PubMed: 7566154]
- 58.
- Fyrberg C, Ketchum A, Ball E. et al. Characterization of lethal Drosophila melanogaster alpha-actinin mutants. Biochem Genet. 1998;36(9-10):299–310. [PubMed: 9919356]
- 59.
- Roulier EM, Fyrberg C, Fyrberg E. Perturbations of Drosophila alpha-actinin cause muscle paralysis, weakness, and atrophy but do not confer obvious nonmuscle phenotypes. J Cell Biol. 1992;116(4):911–922. [PMC free article: PMC2289344] [PubMed: 1734023]
- 60.
- Sokol NS, Cooley L. Drosophila filamin encoded by the cheerio locus is a component of ovarian ring canals. Curr Biol. 1999;9(21):1221–1230. [PubMed: 10556087]
- 61.
- Robinson DN, Smith-Leiker TA, Sokol NS. et al. Formation of the Drosophila ovarian ring canal inner rim depends on cheerio. Genetics. 1997;145(4):1063–1072. [PMC free article: PMC1207876] [PubMed: 9093858]
- 62.
- Strutt D, Johnson R, Cooper K. et al. Asymmetric localization of frizzled and the determination of notch-dependent cell fate in the Drosophila eye. Curr Biol. 2002;12(10):813–824. [PubMed: 12015117]
- 63.
- Roulier EM, Panzer S, Beckendorf SK. The Tec29 tyrosine kinase is required during Drosophila embryogenesis and interacts with Src64 in ring canal development. Mol Cell. 1998;1(6):819–829. [PubMed: 9660965]
- 64.
- Tateno M, Nishida Y, Adachi-Yamada T. Regulation of JNK by Src during Drosophila development. Science. 2000;287(5451):324–327. [PubMed: 10634792]
- 65.
- Read RD, Bach EA, Cagan RL. Drosophila C-terminal Src kinase negatively regulates organ growth and cell proliferation through inhibition of the Src, Jun N-terminal kinase, and STAT pathways. Mol Cell Biol. 2004;24(15):6676–6689. [PMC free article: PMC444864] [PubMed: 15254235]
- 66.
- Pedraza LG, Stewart RA, Li DM. et al. Drosophila Src-family kinases function with Csk to regulate cell proliferation and apoptosis. Oncogene. 2004;23(27):4754–4762. [PubMed: 15107833]
- 67.
- Damsky CH, Ilic D. Integrin signaling: It's where the action is. Curr Opin Cell Biol. 2002;14(5):594–602. [PubMed: 12231355]
- 68.
- DeMali KA, Wennerberg K, Burridge K. Integrin signaling to the actin cytoskeleton. Curr Opin Cell Biol. 2003;15(5):572–582. [PubMed: 14519392]
- 69.
- Martin-Bermudo MD, Brown NH. Uncoupling integrin adhesion and signaling: The betaPS cytoplasmic domain is sufficient to regulate gene expression in the Drosophila embryo. Genes Dev. 1999;13(6):729–739. [PMC free article: PMC316550] [PubMed: 10090729]
- 70.
- Martin-Bermudo MD. Integrins modulate the Egfr signaling pathway to regulate tendon cell differentiation in the Drosophila embryo. Development. 2000;127(12):2607–2615. [PubMed: 10821759]
- 71.
- Baker SE, Lorenzen JA, Miller SW. et al. Genetic interaction between integrins and moleskin, a gene encoding a Drosophila homolog of importin-7. Genetics. 2002;162(1):285–296. [PMC free article: PMC1462259] [PubMed: 12242240]
- 72.
- Araujo H, Negreiros E, Bier E. Integrins modulate Sog activity in the Drosophila wing. Development. 2003;130(16):3851–3864. [PubMed: 12835400]
- 73.
- Gonzalez-Reyes A, St JohnstonD. The Drosophila AP axis is polarised by the cadherin-mediated positioning of the oocyte. Development. 1998;125(18):3635–3644. [PubMed: 9716529]
- 74.
- Godt D, Tepass U. Drosophila oocyte localization is mediated by differential cadherin-based adhesion. Nature. 1998;395(6700):387–391. [PubMed: 9759729]
- 75.
- Roote CE, Zusman S. Functions for PS integrins in tissue adhesion, migration, and shape changes during early embryonic development in Drosophila. Dev Biol. 1995;169(1):322–336. [PubMed: 7750648]
- 76.
- Martin-Bermudo MD, Alvarez-Garcia I, Brown NH. Migration of the Drosophila primordial midgut cells requires coordination of diverse PS integrin functions. Development. 1999;126(22):5161–5169. [PubMed: 10529432]
- 77.
- Boube M, Martin-Bermudo MD, Brown NH. et al. Specific tracheal migration is mediated by complementary expression of cell surface proteins. Genes Dev. 2001;15(12):1554–1562. [PMC free article: PMC312719] [PubMed: 11410535]
- 78.
- Bradley PL, Myat MM, Comeaux CA. et al. Posterior migration of the salivary gland requires an intact visceral mesoderm and integrin function. Dev Biol. 2003;257(2):249–262. [PubMed: 12729556]
- 79.
- Leptin M, Bogaert T, Lehmann R. et al. The function of PS integrins during Drosophila embryogenesis. Cell. 1989;56(3):401–408. [PubMed: 2492451]
- 80.
- Schnorrer F, Dickson BJ. Muscle building; mechanisms of myotube guidance and attachment site selection. Dev Cell. 2004;7(1):9–20. [PubMed: 15239950]
- 81.
- Schwander M, Leu M, Stumm M. et al. Beta1 integrins regulate myoblast fusion and sarcomere assembly. Dev Cell. 2003;4(5):673–685. [PubMed: 12737803]
- 82.
- Brown NH. Null mutations in the alpha PS2 and beta PS integrin subunit genes have distinct phenotypes. Development. 1994;120(5):1221–1231. [PubMed: 8026331]
- 83.
- Wilkin MB, Becker MN, Mulvey D. et al. Drosophila dumpy is a gigantic extracellular protein required to maintain tension at epidermal-cuticle attachment sites. Curr Biol. 2000;10(10):559–567. [PubMed: 10837220]
- 84.
- Jazwinska A, Ribeiro C, Affolter M. Epithelial tube morphogenesis during Drosophila tracheal development requires Piopio, a luminal ZP protein. Nat Cell Biol. 2003;5(10):895–901. [PubMed: 12973360]
- 85.
- Bokel C, Prokop A, Brown NH. Papillote and Piopio, Drosophila ZP-domain proteins required for cell adhesion to the apical extracellular matrix and microtubule organisation J Cell Sci 2004. In Press . [PubMed: 15657084]
- 86.
- Prokop A, Uhler J, Roote J. et al. The kakapo mutation affects terminal arborization and central dendritic sprouting of Drosophila motorneurons. J Cell Biol. 1998;143(5):1283–1294. [PMC free article: PMC2133088] [PubMed: 9832556]
- 87.
- Reed BH, Wilk R, Schock F. et al. Integrin-dependent apposition of Drosophila extraembryonic membranes promotes morphogenesis and prevents anoikis. Curr Biol. 2004;14(5):372–380. [PubMed: 15028211]
- 88.
- Hutson MS, Tokutake Y, Chang MS. et al. Forces for morphogenesis investigated with laser microsurgery and quantitative modeling. Science. 2003;300(5616):145–149. [PubMed: 12574496]
- 89.
- Jacinto A, Wood W, Balayo T. et al. Dynamic actin-based epithelial adhesion and cell matching during Drosophila dorsal closure. Curr Biol. 2000;10(22):1420–1426. [PubMed: 11102803]
- 90.
- Ashton GH, McLean WH, South AP. et al. Recurrent mutations in kindlin-1, a novel keratinocyte focal contact protein, in the autosomal recessive skin fragility and photosensitivity disorder, Kindler syndrome. J Invest Dermatol. 2004;122(1):78–83. [PubMed: 14962093]
- 91.
- Brabant MC, Fristrom D, Bunch TA. et al. Distinct spatial and temporal functions for PS integrins during Drosophila wing morphogenesis. Development. 1996;122(10):3307–3317. [PubMed: 8898242]
- 92.
- Fristrom D, Wilcox M, Fristrom J. The distribution of PS integrins, laminin A and F-actin during key stages in Drosophila wing development. Development. 1993;117(2):509–523. [PubMed: 8330522]
- 93.
- Longley JrRL, Ready DF. Integrins and the development of three-dimensional structure in the Drosophila compound eye. Dev Biol. 1995;171(2):415–433. [PubMed: 7556924]
- 94.
- D'Avino PP, Thummel CS. The ecdysone regulatory pathway controls wing morphogenesis and integrin expression during Drosophila metamorphosis. Dev Biol. 2000;220(2):211–224. [PubMed: 10753511]
- 95.
- Kozlova T, Thummel CS. Essential roles for ecdysone signaling during Drosophila mid-embryonic development. Science. 2003;301(5641):1911–1914. [PubMed: 12958367]
- 96.
- Kadrmas JL, Smith MA, Clark KA. et al. The integrin effector PINCH regulates JNK activity and epithelial migration in concert with Ras suppressor 1. J Cell Biol. 2004;167(6):1019–1024. [PMC free article: PMC2034365] [PubMed: 15596544]
- 97.
- Tanentzapf G, Smith C, McGlade J. et al. Apical, lateral, and basal polarization cues contribute to the development of the follicular epithelium during Drosophila oogenesis. J Cell Biol. 2000;151(4):891–904. [PMC free article: PMC2169434] [PubMed: 11076972]
- 98.
- Bilder D. Epithelial polarity and proliferation control: Links from the Drosophila neoplastic tumor suppressors. Genes Dev. 2004;18(16):1909–1925. [PubMed: 15314019]
- 99.
- Hoang B, Chiba A. Genetic analysis on the role of integrin during axon guidance in Drosophila. J Neurosci. 1998;18(19):7847–7855. [PMC free article: PMC6793012] [PubMed: 9742153]
- 100.
- Lee S, Harris KL, Whitington PM. et al. Short stop is allelic to kakapo, and encodes rod-like cytoskeletal-associated proteins required for axon extension. J Neurosci. 2000;20(3):1096–1108. [PMC free article: PMC6774164] [PubMed: 10648715]
- 101.
- Rohrbough J, Grotewiel MS, Davis RL. et al. Integrin-mediated regulation of synaptic morphology, transmission, and plasticity. J Neurosci. 2000;20(18):6868–6878. [PMC free article: PMC6772806] [PubMed: 10995831]
- 102.
- Beumer K, Matthies HJ, Bradshaw A. et al. Integrins regulate DLG/FAS2 via a CaM kinase II-dependent pathway to mediate synapse elaboration and stabilization during postembryonic development. Development. 2002;129(14):3381–3391. [PubMed: 12091308]
- 103.
- Brower DL, Jaffe SM. Requirement for integrins during Drosophila wing development. Nature. 1989;342(6247):285–287. [PubMed: 2509946]
- 104.
- Martin-Bermudo MD, Dunin-Borkowski OM, Brown NH. Specificity of PS integrin function during embryogenesis resides in the alpha subunit extracellular domain. EMBO J. 1997;16(14):4184–4193. [PMC free article: PMC1170044] [PubMed: 9250662]
- 105.
- Nayal A, Webb DJ, Horwitz AF. Talin: An emerging focal point of adhesion dynamics. Curr Opin Cell Biol. 2004;16(1):94–98. [PubMed: 15037311]
- 106.
- Brakebusch C, Fassler R. The integrin-actin connection, an eternal love affair. EMBO J. 2003;22(10):2324–2333. [PMC free article: PMC156003] [PubMed: 12743027]
- 107.
- Zervas CG, Brown NH. Integrin adhesion: When is a kinase a kinase? Curr Biol. 2002;12(10):R350–351. [PubMed: 12015134]
- 108.
- Wilcox M, DiAntonio A, Leptin M. The function of PS integrins in Drosophila wing morphogenesis. Development. 1989;107(4):891–897. [PubMed: 10532829]
- 109.
- Roper K, Gregory SL, Brown NH. The ‘spectraplakins’: Cytoskeletal giants with characteristics of both spectrin and plakin families. J Cell Sci. 2002;115(Pt 22):4215–4225. [PubMed: 12376554]
- 110.
- Tadokoro S, Shattil SJ, Eto K. et al. Talin binding to integrin beta tails: A final common step in integrin activation. Science. 2003;302(5642):103–106. [PubMed: 14526080]
- 111.
- Monkley SJ, Zhou XH, Kinston SJ. et al. Disruption of the talin gene arrests mouse development at the gastrulation stage. Dev Dyn. 2000;219(4):560–574. [PubMed: 11084655]
- 112.
- Giannone G, Jiang G, Sutton DH. et al. Talin1 is critical for force-dependent reinforcement of initial integrin-cytoskeleton bonds but not tyrosine kinase activation. J Cell Biol. 2003;163(2):409–419. [PMC free article: PMC2173516] [PubMed: 14581461]
- 113.
- Mackinnon AC, Qadota H, Norman KR. et al. C. elegans PAT-4/ILK functions as an adaptor protein within integrin adhesion complexes. Curr Biol. 2002;12(10):787–797. [PubMed: 12015115]
- 114.
- Troussard AA, Mawji NM, Ong C. et al. Conditional knock-out of integrin-linked kinase demonstrates an essential role in protein kinase B/Akt activation. J Biol Chem. 2003;278(25):22374–22378. [PubMed: 12686550]
- 115.
- Zusman S, Patel-King RS, Ffrench-Constant C. et al. Requirements for integrins during Drosophila development. Development. 1990;108(3):391–402. [PubMed: 2340808]
- 116.
- Jobard F, Bouadjar B, Caux F. et al. Identification of mutations in a new gene encoding a FERM family protein with a pleckstrin homology domain in Kindler syndrome. Hum Mol Genet. 2003;12(8):925–935. [PubMed: 12668616]
- 117.
- Brabant MC, Fristrom D, Bunch TA. et al. The PS integrins are required for a regulatory event during Drosophila wing morphogenesis. Ann NY Acad Sci. 1998;857:99–109. [PubMed: 9917835]
- 118.
- Henchcliffe C, Garcia-Alonso L, Tang J. et al. Genetic analysis of laminin A reveals diverse functions during morphogenesis in Drosophila. Development. 1993;118(2):325–337. [PubMed: 8223265]
- 119.
- Wu C, Dedhar S. Integrin-linked kinase (ILK) and its interactors: A new paradigm for the coupling of extracellular matrix to actin cytoskeleton and signaling complexes. J Cell Biol. 2001;155(4):505–510. [PMC free article: PMC2198863] [PubMed: 11696562]
- 120.
- Sakai T, Li S, Docheva D. et al. Integrin-linked kinase (ILK) is required for polarizing the epiblast, cell adhesion, and controlling actin accumulation. Genes Dev. 2003;17(7):926–940. [PMC free article: PMC196029] [PubMed: 12670870]
- 121.
- Stronach BE, Perrimon N. Stress signaling in Drosophila. Oncogene. 1999;18(45):6172–6182. [PubMed: 10557109]
- 122.
- Hartenstein V. Atlas of Drosophila developmentNew York: Cold Spring Harbor Laboratory Press,1993.
- Introduction
- Integrins and Associated Proteins: Simplicity Is a Good Thing
- Genetics of Integrins and Integrin-Associated Proteins
- Integrin Functions in Fly Development: Sculpting Tissues through Adhesion and Migration
- Migration
- Insights into the Mechanism of Integrin Function
- Future Prospects
- Acknowledgements
- References
- Integrins and Associated Proteins in Drosophila Development - Madame Curie Biosc...Integrins and Associated Proteins in Drosophila Development - Madame Curie Bioscience Database
- The Structure of the Type 1 Insulin-Like Growth Factor Receptor - Madame Curie B...The Structure of the Type 1 Insulin-Like Growth Factor Receptor - Madame Curie Bioscience Database
- Phylogeny of Major Intrinsic Proteins - Madame Curie Bioscience DatabasePhylogeny of Major Intrinsic Proteins - Madame Curie Bioscience Database
- Molecular Properties of Voltage-Gated Calcium Channels - Madame Curie Bioscience...Molecular Properties of Voltage-Gated Calcium Channels - Madame Curie Bioscience Database
- Matrix Metalloproteinases, Tissue Inhibitors of Metalloproteinase and Matrix Tur...Matrix Metalloproteinases, Tissue Inhibitors of Metalloproteinase and Matrix Turnover and the Fate of Hepatic Stellate Cells - Madame Curie Bioscience Database
Your browsing activity is empty.
Activity recording is turned off.
See more...