NCBI Bookshelf. A service of the National Library of Medicine, National Institutes of Health.
Madame Curie Bioscience Database [Internet]. Austin (TX): Landes Bioscience; 2000-2013.
Regulation of the phagocytic process involves complex signaling pathways that lead to particle internalization and destruction. Phagocytosis, however, is not a cellular response occurring as an isolated event. Phagocytic signaling involves the regulation of many phagocytosis-associated cell responses that are important for host defense and for the resolution of the inflammatory process. In addition, due to the destructive nature of the phagocytic process, this cell response is tightly controlled, and phagocytes must respond to activation and differentiation signals that modulate their phagocytic efficiency. Presently it is unclear how all these events are coordinated. Available information, however, suggests a model in which phagocytosis-associated cell responses are regulated through signaling pathways that occur in parallel to, and partially overlap those regulating the ingestion process itself. Additionally, activation and differentiation signals appear to potentiate, or modify the utilization important signaling enzymes that regulate phagocytosis, in order to make this process more efficient.
Introduction
The immune system has a specialized subset of cells, named professional phagocytes, equipped for rapidly and efficiently ingesting invading microorganisms at sites of inflammation. These phagocytes are neutrophils and macrophages.1 Monocytes (the macrophage precursors) are often included among the professional phagocytes, though they display a lower phagocytic response than neutrophils and macrophages.1,2 Other nonprofessional phagocytes are dendritic cells,3,4 fibroblasts,5 microglia,6 and some epithelial cell types.7,8 An important difference between the phagocytic process of professional and nonprofessional phagocytes is that professional phagocytes respond to a phagocytic stimulus with many associated cell responses. These responses are of great importance in the context of the immune response, during tissue remodeling, and wound healing. Phagocytosis-associated cell responses include immuno-modulatory responses like the generation and release of pro-inflammatory9,10 and anti-inflammatory mediators,11 and also cell responses of destructive nature such as the respiratory burst,12 and the release of toxic and microbicidal molecules by degranulation.13,14 Additionally, in contrast to nonprofessional phagocytes, professional phagocytes are capable of recognizing a wide variety of phagocytic targets, and of ingesting them at a higher rate. However, in order to achieve controlled, yet efficient, phagocytic functions, professional phagocytes must respond to activation and differentiation signals that modulate their phagocytic abilities. The regulation of phagocytosis-associated cell responses and the modulation of phagocytic efficiency thus represent a higher level of complexity for phagocytic signaling. Available evidence of how these events are regulated at the molecular level will be deal with in the next sections.
Regulation of Phagocytosis-Associated Cell Responses
As early as 1977 it was recognized that, in contrast to nonprofessional phagocytes, professional phagocytes were capable of secreting pro-inflammatory mediators in response to a phagocytic stimulus.15 It is now well established that professional phagocytes respond to a phagocytic stimulus with a wide variety of associated cell responses.1,2 Phagocytosis-associated cell responses appear to be either inflammatory or anti-inflammatory, predominantly depending on the properties of the phagocytic target. Inflammatory responses include the respiratory burst,12 the release of pro-inflammatory mediators (cytokines, prostaglandins, and leukotrienes),9,10 and the release of various microbicidal molecules by degranulation.13,14 Anti-inflammatory responses are mainly characterized by the production and release of anti-inflammatory cytokines, and the active inhibition of the generation of pro-inflammatory mediators.10
The nature of the phagocytic target has a strong influence on the particular cell response that will accompany phagocytosis. Early reports indicated that phagocytosis of IgG-,16-18 but not complement-opsonized18,19 particles induced the release of granule enzymes and lipid-derived pro-inflammatory mediators. In contrast, phagocytosis of apoptotic cells by macrophages occurred in the absence of both responses.20 More recently it was found that phagocytosis of apoptotic cells actually increases the secretion of the anti-inflammatory cytokine TGF-β.21 Phagocytosis of necrotic cells by macrophages, on the other hand, induces an inflammatory phenotype characterized by the up-regulation of T cell costimulatory molecules, and enhanced ability for antigen presentation.21 From these observations it follows that the receptors engaged in the phagocytic process will determine what associated cell responses will be triggered (fig. 1). Phagocytosis mediated by immunoglobulin (Ig) receptors (FcRs) is associated with various inflammatory responses. Phagocytosis mediated by FcγRs (receptors for IgG) or FcαR (receptor for IgA) triggers pro-inflammatory cell responses including the respiratory burst, the release of microbicidal molecules by degranulation, and the production and release of lipid-derived pro-inflammatory factors and cytokines2,22-25 (fig. 1A). Phagocytosis mediated by complement receptors (CRs), on the other hand, is not always associated with inflammatory cell responses. Phagocytosis mediated by complement receptor 4 (CR4) occurs in association with the respiratory burst26 (fig. 1B). Complement receptor 3 (CR3), on the other hand, recognizes a wide variety of ligands,27 and phagocytosis mediated by this receptor can occur in the absence or presence of the respiratory burst. This will depend on the ligand-binding site engaged on the receptor.28 Engagement of the CR3 binding site for complement, during phagocytosis, does not trigger inflammatory responses.18,19,26,29 In contrast, when the fibrinogen (a component of the coagulation cascade) binding site,30 or the lectin site (that recognizes microorganism-derived sugars) on CR328,31 are engaged phagocytes present a strong respiratory burst and cytotoxic activity (fig. 1C). In contrast to phagocytosis mediated by Fc receptors or complement receptors, phagocytosis of apoptotic cells has been directly associated with anti-inflammatory cell responses11 (fig. 1D). The anti-inflammatory nature of apoptotic cell phagocytosis may be fundamental for the clearance of apoptotic cells during tissue remodeling and wound healing. The engagement of the phosphatidylserine receptor during phagocytosis of apoptotic cells induces the production of anti-inflammatory mediators, and actively suppresses the production of inflammatory mediators.5,32,33 MER is another receptor with a relevant role in the clearance of apoptotic cells,34,35 and it was recently found that its stimulation is necessary for down-regulating inflammatory responses.36,37 Interestingly, engagement of CR3 during phagocytosis of apoptotic cells also appears to down-regulate the production and secretion of pro-inflammatory cytokines.38 Because CR3 may cooperate with the phosphatidylserine receptor during phagocytosis of apoptotic cells,39 it is possible that the anti-inflammatory effect of CR3 is due to simultaneous phosphatidylserine receptor stimulation.
It is currently unclear how these varied cell responses are regulated along with the phagocytic process. In some cases phagocytosis and accompanying cell responses appear to be regulated by parallel signaling pathways that are triggered by the same receptors that mediate phagocytosis (fig. 2). For example, it has been shown that in monocytes FcγR-mediated phagocytosis is regulated through a signaling pathway dependent on PCK, and independent of PI 3-K and ERK40 (fig. 2). In the same cells, however, FcγR stimulation triggers a signaling pathway for cytokine production that depends on PI 3-K and ERK40 (fig. 2). In other cases phagocytosis and its associated cell responses appear to be regulated through partially overlapping signaling pathways (fig. 3). In professional phagocytes, for instance, FcγR-mediated phagocytosis is regulated through a signaling pathway dependent on phosphatidylinositol 3-kinase (PI 3-K),41,42 protein kinase C (PKC),43,44 extracellular signal-regulated kinase (ERK),40,45,46 Rac,29 and various phospholipases25 (fig. 3). FcγR-mediated phagocytosis, however, can proceed independently of changes in the concentration of intracellular calcium47,48 (fig. 3). The activation of the respiratory burst, on the other hand, also depends PI 3-K,49-51 PKC,49,52 ERK,53,54 Rac,55,56 and various phospholipases,50,57-59 but is in contrast dependent on calcium60,61 (fig. 3). In macrophages the activity of different PKC isoforms has been ascribed to the regulation of either phagocytosis, or the respiratory burst.52 Phagocytosis appears to be regulated by PKCδ, and PKCε, while the respiratory burst is regulated by the PKCα isoform.52 This observations suggest that, although the signaling pathways for phagocytosis and the respiratory burst share key signaling enzymes, they may not overlap completely.
Modulation of Phagocytic Efficiency
Compared to nonprofessional phagocytes, professional phagocytes present a great ability to recognize phagocytic targets, and ingest them at a higher rate.1,2 In addition to this, professional phagocytes are able to modulate their phagocytic functions in response to activation and differentiation signals.
Cell Activation and Phagocytic Signaling
Phagocytosis efficiency of IgG- and complement-opsonized targets is up-regulated in response to a wide variety of activating stimuli (fig. 4). These include, bacterial peptides,62,63 cytokines,64-68 lipid-derived pro-inflammatory mediators,64,69,70 and the simultaneous stimulation of additional adhesive or phagocytic receptors.71-74 How these activation signals up-regulate phagocytic efficiency at the molecular level is still unclear, however, in some cases the activating stimulus appears to potentiate the activation of signaling enzymes with important roles in phagocytosis (fig. 4). For example, neutrophil activation with leukotriene B4 (a lipid-derived pro-inflammatory mediator) results in enhanced Syk activation.70 This event correlates with increased phagocytic efficiency towards IgG-opsonized targets,70 which is a cell response regulated by Syk.75 Similarly, other activating stimuli have been reported to activate enzymes that professional phagocytes require for efficient phagocytosis, particularly ERK and PI 3-K.42 Activating stimuli known to activate ERK and/or PI 3-K include, the bacterial peptide fMLP,76,77 leukotrienes,78,79 and cytokines such as IL-8,80 granulocyte colony-stimulating factor,81 and granulocyte-macrophage colony-stimulating factor.77
Cell Differentiation and Phagocytic Signaling
In the immune system neutrophils and macrophages represent the fully differentiated phagocytes. While neutrophils leaving the bone marrow are fully differentiated, macrophages differentiate from circulating monocytes in extra-vascular tissues.82 Monocytes display a lower phagocytic response, compared to neutrophils and macrophages, and must respond to activation and differentiation signals in order to achieve optimal phagocytic capacity1,2 (fig. 5). It has been shown that the monocyte ability to ingest various targets is influenced by their state of differentiation. IgG-coated targets are ingested at a higher rate by mature macrophages, compared to undifferentiated monocytes42 (fig. 5, fig. 6). Similarly, the ability to phagocytose via complement receptors is dependent on monocyte maturation.83,84 While monocytes require an activating stimulus for internalizing complement-coated targets83 (fig. 5), mature macrophages ingest complement-opsonized targets in the absence of additional stimulus83 (fig. 6). Similarly, the ability of monocytes and bone marrow-derived macrophages to recognize and ingest apoptotic cells is inducible, and depends on their state of differentiation85,86 (fig. 5, fig. 6). While the process of monocyte-to-macrophage differentiation has been relatively well characterized,9,82,87 the way the differentiation process enhances phagocytic ability is still unclear. Recent work, however, suggests that the differentiation process involves changes in the molecular machinery that regulates phagocytosis (fig. 7, fig.8). In monocytes, the signaling pathway for phagocytosis depends on PKC,42 but is independent of PI 3-K and ERK40 (fig.7). The process of monocyte-to-macrophage differentiation, however, involves the ordered recruitment of PI 3-K and ERK for phagocytosis regulation.42 Thus in mature macrophages phagocytosis of IgG-coated targets is regulated by PKC, and also by PI 3-K and ERK (fig.8). The PKC isoforms required for phagocytosis, however, appear to be different in monocytes and mature macrophages. In monocytes it was found that FcγRI stimulation increases PKC activity corresponding to the membrane translocation of the PKC isoforms δ, ε, and ζ88 (fig. 7). In monocyte-differentiated macrophages, on the other hand, FcγRI stimulation leads to PKC activity that corresponds to membrane translocation of the PKC isoforms α, β, and γ88,89 (fig. 8). It has also been demonstrated that PKC activation in monocyte-differentiated macrophages results in a stronger phagocytic response, compared to that of undifferentiated monocytes.42 It is thus possible that by recruiting PI 3-K and ERK for phagocytosis regulation, and by regulating which PKC isoforms are recruited to the membrane, professional phagocytes achieve optimal levels of phagocytosis (fig. 7, fig. 8). Phospholipase A2 (PLA2) is another signaling molecule whose enzymatic activity is necessary for phagocytosis regulation. This enzyme acts down-stream of PKC and ERK, and the product of its enzymatic activity (arachidonic acid) regulates membrane remodeling events, that are necessary for pseudopod extension during phagocytosis.90,91 During phagocytosis arachidonic acid is released through the enzymatic activity of various PLA2 isoforms,25 and the release of this product also appears to be subjected to differentiation-dependent modulation. In monocytes arachidonic acid is released solely by calcium independent-PLA2, under PKC control44,92,93 (fig. 7). In macrophages, on the other hand, arachidonic acid is released by calcium dependent-PLA2, under ERK and p38 MAPK control,94,95 and also by a calcium-independent PLA2, under PKC control96 (fig. 8). It is thus likely that by modulating the signaling pathways leading to arachidonic acid release, professional phagocytes also achieve an optimal phagocytic function. It thus appears that the process of monocyte-to-macrophage differentiation involves changes in the utilization of key signaling enzymes, whose activity may result in an enhanced phagocytic function.
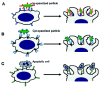
Figure 6
Recognition and ingestion of phagocytic targets by macrophages. Macrophages recognize and ingest very efficiently immunoglobulin (Ig)-opsonized targets (A), complement (Cpt)-opsonized targets (B), and also apoptotic cells (C).
Conclusion
Phagocytosis is cell response with important functions in the course of an immune response, but also during tissue remodeling and wound healing. Professional phagocytes are specialized cells capable of performing this function with great efficiency. The phagocytic process, however, is not a cell response that occurs as an isolated event, and a phagocytic stimulus triggers associated cell responses of destructive nature, but also immuno-modulatory cell responses. The concurrent regulation of these cell responses with phagocytosis sometimes involves separate, apparently independent signaling pathways. In other cases, however, although the signaling pathways for phagocytosis and associated responses share key signaling enzymes, these pathways may not be completely overlapping.
Professional phagocytes, in order to achieve controlled, yet efficient, phagocytic functions, must respond to activation and differentiation signals that modulate their phagocytic abilities. On one hand, activating stimulus appears to potentiate the activation of signaling enzymes with important roles in phagocytosis. On the other hand, differentiation signals that control the process of monocyte-to-macrophage differentiation promote a change in the utilization of key signaling enzymes for phagocytosis. This changes thus result in an enhanced phagocytic function.
The regulation of phagocytosis-associated cell responses and the modulation of phagocytic efficiency in response to activation and differentiation signals thus represent a higher level of complexity for phagocytic signaling. Although much work remains to be done in this field, future studies will enable us to better understand the complex signaling networks that regulate fundamental cellular mechanisms of the immune response.
Definitions
Apoptotic cell—Cell that has undergone the process of programmed cell death (apoptosis). This process is the result of the activation of specific signaling pathways, relevant during embryonic development, during the course of the immune response, and during the wound-healing process.
Complement—Family of soluble blood proteins that are part of the innate defense system. These proteins circulate in an inactive form, but become activated by the recognition of molecular components of microorganisms. Activation of complement components promotes their binding to the surface of microorganisms and function as opsonins. Additionally, activation of the complement cascade culminates in the formation of the membrane attack complex, that is capable of forming pores on the membrane of cells and pathogens.
Cytokines—Soluble, hormone-like proteins produced by leukocytes that act as a messengers between cells. Cytokines can stimulate or inhibit the growth and activity of various immune cells, and have effects on the differentiation and proliferation of the hematopoietic stem cells, as well as on the activation of lymphocytes and phagocytes.
Degranulation—Process whereby cells eject the contents of cytoplasmic vesicles (granules), either into a phagosome, or to the exterior of the cell through exocytosis. This process is characteristic of mast cells, basophils, neutrophils, macrophages, eosinophils, and platelets. During this process pro-inflammatory mediators, microbicidal and potentially cytotoxic substances are released from preformed cytoplasmic granules.
Nonprofessional phagocytes—Various cell types with low phagocytic activity. Nonprofessional phagocytes have more limited recognition and ingestion capabilities, compared to professional phagocytes.
Opsonization—Process whereby soluble proteins (such as immunoglobulins or complement components) bind to the surface pathogens and make them susceptible to ingestion by phagocytes.
Professional phagocytes—Specialized subset of cells in the immune system, capable of ingesting cells and pathogens with great efficiency, through the recognition of a wide array of molecular determinants of microorganisms, and also opsonins bound to pathogens and dying cells.
Prostaglandins and leukotrienes—A family of biologically active lipid compounds, derived from arachidonic acid by oxidative metabolism through the cyclooxygenase (prostaglandins), or 5-lipoxygenase (leukotrienes) pathways. They participate in host defense reactions modulating the inflammatory response. They have potent actions on many essential organs and systems, including the cardiovascular, pulmonary, and central nervous system, as well as the gastrointestinal tract and the immune system. Prostaglandins and leukotrienes are released by phagocytes.
Respiratory burst—Increase in oxidative metabolism of phagocytes following uptake of opsonized particles. The activation of the respiratory burst results in the production of toxic reactive-oxygen and nitrogen metabolites with bactericidal properties. These metabolites include hydrogen peroxide, hypohalites and nitric oxide.
Acknowledgements
Research is the author's laboratory is supported by grant 36407-M from Consejo Nacional de Ciencia y Tecnología (CONACyT), México.
References
- 1.
- Rabinovitch M. Professional and nonprofessional phagocytes: An introduction. Trends Cell Biol. 1995;5:85–87. [PubMed: 14732160]
- 2.
- Jones SL, Lindberg FP, Brown EJ. PhagocytosisIn: Paul WE, ed.Fundamental Immunology4th ed. Philadelphia: Lippincott-Raven Publishers,1999997–1020.
- 3.
- Rubartelli A, Poggi A, Zocchi MR. The selective engulfment of apoptotic bodies by dendritic cells is mediated by the alpha(v)beta3 integrin and requires intracellular and extracellular calcium. Eur J Immunol. 1997;27:1893–900. [PubMed: 9295024]
- 4.
- Albert ML, Pearce SF, Francisco LM. et al. Immature dendritic cells phagocytose apoptotic cells via alphavbeta5 and CD36, and cross-present antigens to cytotoxic T lymphocytes. J Exp Med. 1998;188:1359–68. [PMC free article: PMC2212488] [PubMed: 9763615]
- 5.
- Fadok VA, Bratton DL, Rose DM. et al. A receptor for phosphatidylserine-specific clearance of apoptotic cells. Nature. 2000;405:85–90. [PubMed: 10811223]
- 6.
- Witting A, Muller P, Herrmann A. et al. Phagocytic clearance of apoptotic neurons by Microglia/ Brain macrophages in vitro: Involvement of lectin-, integrin-, and phosphatidylserine-mediated recognition. J Neurochem. 2000;75:1060–70. [PubMed: 10936187]
- 7.
- Ryeom SW, Sparrow JR, Silverstein RL. CD36 participates in the phagocytosis of rod outer segments by retinal pigment epithelium. J Cell Sci. 1996;109(Pt 2):387–95. [PubMed: 8838662]
- 8.
- Parnaik R, Raff MC, Scholes J. Differences between the clearance of apoptotic cells by professional and nonprofessional phagocytes. Curr Biol. 2000;10:857–60. [PubMed: 10899007]
- 9.
- Gordon S, Clarke S, Greaves D. et al. Molecular immunobiology of macrophages: Recent progress. Curr Op Immunol. 1995;7:24–33. [PubMed: 7772278]
- 10.
- Rosenberg H, Gallin J. InflammationIn: Paul WE, ed.Fundamental Immunology4th ed. Philadelphia: Lippincott-Raven Publishers,19991051–1066.
- 11.
- Platt N, da SilvaRP, Gordon S. Recognizing death: the phagocytosis of apoptotic cells. Trends Cell Biol. 1998;8:365–72. [PubMed: 9728398]
- 12.
- Hampton M, Kettle A, Winterbourn C. Inside the neutrophil phagosome: Oxidants, myeloperoxidase and bacterial killing. Blood. 1998;92:3007–17. [PubMed: 9787133]
- 13.
- Martin E, Ganz T, Lehrer RI. Defensins and other endogenous peptide antibiotics of vertebrates. J Leukoc Biol. 1995;58:128–36. [PubMed: 7643008]
- 14.
- Lehrer RI, Ganz T. Antimicrobial polypeptides of human neutrophils. Blood. 1990;76:2169–81. [PubMed: 2257291]
- 15.
- Bodel P, Miller H. Differences in pyrogen production by mononuclear phagocytes and by fibroblasts or HeLa cells. J Exp Med. 1977;145:607–17. [PMC free article: PMC2180710] [PubMed: 233905]
- 16.
- Nielsen OH, Elmgreen J, Thomsen BS. et al. Release of leukotriene B4 and 5-hydroxyeicosatetraenoic acid during phagocytosis of artificial immune complexes by peripheral neutrophils in chronic inflammatory bowel disease. Clin Exp Immunol. 1986;65:465–71. [PMC free article: PMC1542312] [PubMed: 3024887]
- 17.
- Brozna JP, Hauff NF, Phillips WA. et al. Activation of the respiratory burst in macrophages. Phosphorylation specifically associated with Fc receptor-mediated stimulation. J Immunol. 1988;141:1642–7. [PubMed: 3411128]
- 18.
- Stein M, Gordon S. Regulation of tumor necrosis factor (TNF) release by murine peritoneal macrophages: role of cell stimulation and specific phagocytic plasma membrane receptors. J Cell Sci. 1991;21:431–7. [PubMed: 1999225]
- 19.
- Aderem AA, Wright SD, Silverstein SC. et al. Ligated complement receptors do not activate the arachidonic acid cascade in resident peritoneal macrophages. J Exp Med. 1985;161:617–22. [PMC free article: PMC2187583] [PubMed: 3156206]
- 20.
- Meagher L, Savill J, Baker A. et al. Phagocytosis of apoptotic neutrophils does not induce macrophage release of thromboxane B2. J Leukoc Biol. 1992;52:169–73. [PubMed: 1522386]
- 21.
- Barker R, Erwig L, Pearce W. et al. Differential effects of necrotic or apoptotic cell uptake on antigen presentation by macrophages. Pathobiol. 1999;67:302–5. [PubMed: 10725808]
- 22.
- Monteiro R, Van De Winkel J. IgA Fc receptors. Annu Rev Immunol. 2003;21:177–204. [PubMed: 12524384]
- 23.
- Ravetch JV, Bolland S. IgG Fc receptors. Annu Rev Immunol. 2001;19:275–290. [PubMed: 11244038]
- 24.
- Sαnchez-Mejorada G, Rosales C. Signal transduction by immunoglobulin Fc receptors. J Leukoc Biol. 1998;63:521–533. [PubMed: 9581795]
- 25.
- Lennartz MR. Phospholipases and phagocytosis: the role of phospholipid-derived second messengers in phagocytosis. Int J Biochem Cell Biol. 1999;31:415–430. [PubMed: 10224668]
- 26.
- Berton G, Laudanna C, Sorio C. et al. Generation of signals activating neutrophil functions by leukocyte integrins: LFA-1 and gp150/95, but not CR3, are able to stimulate the respiratory burst of human neutrophils. J Cell Biol. 1992;116:1007–17. [PMC free article: PMC2289342] [PubMed: 1346398]
- 27.
- Ehlers MR. CR3: a general purpose adhesion-recognition receptor essential for innate immunity. Microbes Infect. 2000;2:289–94. [PubMed: 10758405]
- 28.
- Le CabecV, Cols C, Maridonneau-Parini I. Nonopsonic phagocytosis of zymosan and Mycobacterium kansasii by CR3 (CD11b/CD18) involves distinct molecular determinants and is or is not coupled with NADPH oxidase activation. Infect Immun. 2000;68:4736–45. [PMC free article: PMC98424] [PubMed: 10899880]
- 29.
- Caron E, Hall A. Identification of two distinct mechanisms of phagocytosis controlled by different Rho GTPases. Science. 1998;282:1717–21. [PubMed: 9831565]
- 30.
- Rubel C, Fernandez GC, Rosa FA. et al. Soluble fibrinogen modulates neutrophil functionality through the activation of an extracellular signal-regulated kinase-dependent pathway. J Immunol. 2002;168:3527–35. [PubMed: 11907115]
- 31.
- Vetvicka V, Thornton BP, Ross GD. Soluble beta-glucan polysaccharide binding to the lectin site of neutrophil or natural killer cell complement receptor type 3 (CD11b/CD18) generates a primed state of the receptor capable of mediating cytotoxicity of iC3b-opsonized target cells. J Clin Invest. 1996;98:50–61. [PMC free article: PMC507400] [PubMed: 8690804]
- 32.
- Fadok VA, Bratton DL, Konowal A. Trends in CellBiology. Macrophages that have ingested apoptotic cells in vitro inhibit pro-inflammatory cytokine production through autocrine/paracrine mechanisms involving TGF-beta, PGE2, and PAF. J Clin Invest. 1998;101:890–8. [PMC free article: PMC508637] [PubMed: 9466984]
- 33.
- Huynh ML, Fadok VA, Henson PM. Phosphatidylserine-dependent ingestion of apoptotic cells promotes TGF-beta1 secretion and the resolution of inflammation. J Clin Invest. 2002;109:41–50. [PMC free article: PMC150814] [PubMed: 11781349]
- 34.
- Scott RS, McMahon EJ, Pop SM. et al. Phagocytosis and clearance of apoptotic cells is mediated by MER. Nature. 2001;411:207–11. [PubMed: 11346799]
- 35.
- Behrens EM, Gadue P, Gong SY. Trends in Cell Biology. The mer receptor tyrosine kinase: expression and function suggest a role in innate immunity. Eur J Immunol. 2003;33:2160–7. [PubMed: 12884290]
- 36.
- Camenisch T, Koller B, Earp H. Trends in Cell Biology. A novel receptor tyrosine kinase, MER, inhibits TNF-alpha production and lipopolysacharide-induced endotoxic shock. J Immunol. 1999;162:3498–3503. [PubMed: 10092806]
- 37.
- Cohen PL, Caricchio R, Abraham V. et al. Delayed apoptotic cell clearance and lupus-like autoimmunity in mice lacking the c-mer membrane tyrosine kinase. J Exp Med. 2002;196:135–40. [PMC free article: PMC2194017] [PubMed: 12093878]
- 38.
- Morelli AE, Larregina AT, Shufesky WJ. et al. Internalization of circulating apoptotic cells by splenic marginal zone dendritic cells: dependence on complement receptors and effect on cytokine production. Blood. 2003;101:611–20. [PubMed: 12393562]
- 39.
- Mevorach D, Mascarenhas JO, Gershov D. Trends in CellBiology. Complement-dependent clearance of apoptotic cells by human macrophages. J Exp Med. 1998;188:2313–20. [PMC free article: PMC2212421] [PubMed: 9858517]
- 40.
- Garcia-Garcia E, Sanchez-Mejorada G, Rosales C. Phosphatidylinositol 3-kinase and ERK are required for NF-κB activation, but not for phagocytosis. J Leukoc Biol. 2001;70:649–658. [PubMed: 11590203]
- 41.
- Cox D, Tseng CC, Bjekic G. et al. A requirement for phosphatidylinositol 3-kinase in pseudopod extension. J Biol Chem. 1999;274:1240–1247. [PubMed: 9880492]
- 42.
- Garcia-Garcia E, Rosales R, Rosales C. Phosphatidylinositol 3-kinase and extracellular signal-regulated kinase are recruited for Fc receptor-mediated phagocytosis during monocyte to macrophage differentiation. J Leukoc Biol. 2002;72:107–114. [PubMed: 12101269]
- 43.
- Breton A, Descoteaux A. Protein kinase C-α participates in FcγR-mediated phagocytosis in macrophages. Biochem Biophys Res Com. 2000;276:472–476. [PubMed: 11027499]
- 44.
- Karimi K, Gemmill TR, Lennartz MR. Protein kinase C and a calcium-independent phospholipase are required for IgG-mediated phagocytosis by Mono-Mac-6 cells. J Leukoc Biol. 1999;65:854–862. [PubMed: 10380910]
- 45.
- Mansfield PJ, Shayman JA, Boxer LA. Regulation of polymorphonuclear leukocyte phagocytosis by myosin light chain kinase after activation of mitogen-activated protein kinase. Blood. 2000;95:2407–2412. [PubMed: 10733514]
- 46.
- Raeder EM, Mansfield PJ, Hinkovska-Galcheva V. et al. Syk activation initiates downstream signaling events during human polymorphonuclear leukocyte phagocytosis. J Immunol. 1999;163:6785–6793. [PubMed: 10586078]
- 47.
- Myers JT, Swanson JA. Calcium spikes in activated macrophages during Fcgamma receptor-mediated phagocytosis. J Leukoc Biol. 2002;72:677–84. [PubMed: 12377936]
- 48.
- Edberg JC, Lin CT, Lau D. et al. The Ca2+ dependence of human Fc gamma receptor-initiated phagocytosis. J Biol Chem. 1995;270:22301–22307. [PubMed: 7673212]
- 49.
- Yamamori T, Inanami O, Nagahata H. et al. Roles of p38MAPK, PKC and PI3-K in the signaling pathways of NADPH oxidase activation and phagocytosis in bovine polymorphonuclear leukocytes. FEBS Lett. 2000;467:253–258. [PubMed: 10675549]
- 50.
- Liu J, Liu Z, Chuai S. et al. Phospholipase C and phosphatidylinositol 3-kinase signaling are involved in the exogenous arachidonic acid-stimulated respiratory burst in human neutrophils. J Leukoc Biol. 2003;74:428–37. [PubMed: 12949247]
- 51.
- Hirsch E, Katanaev VL, Garlanda C. et al. Central role for G protein-coupled phosphoinositide 3-kinase gamma in inflammation. Science. 2000;287:1049–53. [PubMed: 10669418]
- 52.
- Larsen EC, DiGennaro JA, Saito N. et al. Differential requirement for classic and novel PKC isoforms in respiratory burst and phagocytosis in RAW 264.7 cells. J Immunol. 2000;165:2809–2817. [PubMed: 10946313]
- 53.
- Downey GP, Butler JR, Tapper H. et al. Importance of MEK in neutrophil microbicidal responsiveness. J Immunol. 1998;160:434–443. [PubMed: 9552001]
- 54.
- Dewas C, Fay M, Gougerot-Pocidalo MA. et al. The mitogen-activated protein kinase extracellular signal-regulated kinase 1/2 pathway is involved in formyl-methionyl-leucyl-phenylalanine-induced p47phox phosphorylation in human neutrophils. J Immunol. 2000;165:5238–44. [PubMed: 11046057]
- 55.
- Kreck ML, Freeman JL, Abo A. et al. Membrane association of Rac is required for high activity of the respiratory burst oxidase. Biochemistry. 1996;35:15683–92. [PubMed: 8961931]
- 56.
- Knaus UG, Heyworth PG, Evans T. et al. Regulation of phagocyte oxygen radical production by the GTP-binding protein Rac 2. Science. 1991;254:1512–5. [PubMed: 1660188]
- 57.
- Giron-Calle J, Srivatsa K, Forman HJ. Priming of alveolar macrophage respiratory burst by H(2)O(2) is prevented by phosphatidylcholine-specific phospholipase C inhibitor Tricyclodecan-9-yl-xanthate (D609). J Pharmacol Exp Ther. 2002;301:87–94. [PubMed: 11907161]
- 58.
- Watson F, Lowe GM, Robinson JJ. et al. Phospholipase D-dependent and -independent activation of the neutrophil NADPH oxidase. Biosci Rep. 1994;14:91–102. [PubMed: 7948774]
- 59.
- Aebischer CP, Pasche I, Jorg A. Nanomolar arachidonic acid influences the respiratory burst in eosinophils and neutrophils induced by GTP-binding protein. A comparative study of the respiratory burst in bovine eosinophils and neutrophils. Eur J Biochem. 1993;218:669–77. [PubMed: 8269958]
- 60.
- Bei L, Hu T, Qian ZM. et al. Extracellular Ca2+ regulates the respiratory burst of human neutrophils. Biochim Biophys Acta. 1998;1404:475–83. [PubMed: 9739175]
- 61.
- Hoyal CR, Gozal E, Zhou H. et al. Modulation of the rat alveolar macrophage respiratory burst by hydroperoxides is calcium dependent. Arch Biochem Biophys. 1996;326:166–71. [PubMed: 8579366]
- 62.
- Ogle JD, Noel JG, Sramkoski RM. et al. Effects of chemotactic peptide f-Met-Leu-Phe (FMLP) on C3b receptor (CR1) expression and phagocytosis of microspheres by human neutrophils. Inflammation. 1990;14:337–53. [PubMed: 2141826]
- 63.
- Rosales C, Brown EJ. Two mechanisms for IgG Fc-receptor-mediated phagocytosis by human neutrophils. J Immunol. 1991;146:3937–44. [PubMed: 2033263]
- 64.
- Ogle JD, Noel JG, Sramkoski RM. et al. The effects of cytokines, platelet activating factor, and arachidonate metabolites on C3b receptor (CR1, CD35) expression and phagocytosis by neutrophils. Cytokine. 1990;2:447–55. [PubMed: 1716488]
- 65.
- Collins HL, Bancroft GJ. Cytokine enhancement of complement-dependent phagocytosis by macrophages: synergy of tumor necrosis factor-alpha and granulocyte-macrophage colony-stimulating factor for phagocytosis of Cryptococcus neoformans. Eur J Immunol. 1992;22:1447–54. [PubMed: 1601035]
- 66.
- Pitrak D. Effects of granulocyte colony-stimulating factor and granulocyte-macrophage colony-stimulating factor on the bactericidal functions of neutrophils. Curr Opin Hematol. 1997;4:183–90. [PubMed: 9209834]
- 67.
- Detmers PA, Powell DE, Walz A. et al. Differential effects of neutrophil-activating peptide 1/IL-8 and its homologues on leukocyte adhesion and phagocytosis. J Immunol. 1991;147:4211–7. [PubMed: 1721641]
- 68.
- Mitchell GB, Albright BN, Caswell JL. Effect of interleukin-8 and granulocyte colony-stimulating factor on priming and activation of bovine neutrophils. Infect Immun. 2003;71:1643–9. [PMC free article: PMC152050] [PubMed: 12654776]
- 69.
- Mancuso P, Peters-Golden M. Modulation of alveolar macrophage phagocytosis by leukotrienes is Fc receptor-mediated and protein kinase C-dependent. Am J Respir Cell Mol Biol. 2000;23:727–33. [PubMed: 11104724]
- 70.
- Canetti C, Hu B, Curis J. et al. Syk activation is a leukotriene B4-regulated event involved in macrophage phagocytosis of IgG-coated targets but not apoptotic cells. Blood. 2003;102:1877–83. [PubMed: 12730109]
- 71.
- Arora M, Muñoz E, Tenner A. Identification of a site on mannan-binding lectin crititcal for enhancement of phagocytosis. J Biol Chem. 2001;276:43087–94. [PubMed: 11533031]
- 72.
- Ohkuro M, Ogura-Masaki M, Kobayashi K. et al. Effect of iC3b binding to immune complexes upon the phagocytic response of human neutrophils: synergistic functions between Fc gamma R and CR3. FEBS Lett. 1995;373:189–92. [PubMed: 7589463]
- 73.
- Schnitzler N, Haase G, Podbielsky A. et al. A costimulatory signal through ICAM-beta 2 integrin-binding potentiates neutrophil phagocytosis. Nat Med. 1999;5:231–5. [PubMed: 9930874]
- 74.
- Rubel C, Fernandez GC, Dran G, Bompadre MB, Isturiz MA, Palermo MS. Fibrinogen promotes neutrophil activation and delays apoptosis. J Immunol. 2001;166:2002–10. [PubMed: 11160249]
- 75.
- Kiefer F, Brumell J, Al-Alawi N. et al. The Syk protein tyrosine kinase is essential for Fcgamma receptor signaling in macrophages and neutrophils. Mol Cell Biol. 1998;18:4209–20. [PMC free article: PMC109005] [PubMed: 9632805]
- 76.
- Chang LC, Wang JP. Examination of the signal transduction pathways leading to activation of extracellular signal-regulated kinase by formyl-methionyl-leucyl-phenylalanine in rat neutrophils. FEBS Lett. 1999;454:165–8. [PubMed: 10413116]
- 77.
- Kodama T, Hazeki K, Hazeki O. et al. Enhancement of chemotactic peptide-induced activation of phosphoinositide 3-kinase by granulocyte-macrophage colony-stimulating factor and its relation to the cytokine-mediated priming of neutrophil superoxide-anion production. Biochem J. 1999;337(Pt 2):201–9. [PMC free article: PMC1219953] [PubMed: 9882616]
- 78.
- Woo CH, You HJ, Cho SH. et al. Leukotriene B(4) stimulates Rac-ERK cascade to generate reactive oxygen species that mediates chemotaxis. J Biol Chem. 2002;277:8572–8. [PubMed: 11756405]
- 79.
- Ito N, Yokomizo T, Sasaki T. et al. Requirement of phosphatidylinositol 3-kinase activation and calcium influx for leukotriene B4-induced enzyme release. J Biol Chem. 2002;277:44898–904. [PubMed: 12244116]
- 80.
- Knall C, Worthen GS, Johnson GL. Interleukin 8-stimulated phosphatidylinositol-3-kinase activity regulates the migration of human neutrophils independent of extracellular signal-regulated kinase and p38 mitogen-activated protein kinases. Proc Natl Acad Sci USA. 1997;94:3052–7. [PMC free article: PMC20320] [PubMed: 9096344]
- 81.
- Kuroki M, O'Flaherty JT. Extracellular signal-regulated protein kinase (ERK)-dependent and ERK-independent pathways target STAT3 on serine-727 in human neutrophils stimulated by chemotactic factors and cytokines. Biochem J. 1999;341( Pt 3):691–6. [PMC free article: PMC1220407] [PubMed: 10417333]
- 82.
- Gordon S. Macrophages and the immune responseIn: Paul WE, ed.Fundamental ImmunologyPhiladelphia: Lippincott-Raven Publishers,1999533–547.
- 83.
- Newman SL, Devery-Pocius JE, Ross GD. et al. Phagocytosis by human monocyte-derived macrophages. Independent function of receptors for C3b (CR1) and iC3b (CR3). Complement. 1984;1:213–27. [PubMed: 6242391]
- 84.
- Newman SL, Becker S, Halme J. Phagocytosis by receptors for C3b (CR1), iC3b (CR3), and IgG (Fc) on human peritoneal macrophages. J Leukoc Biol. 1985;38:267–78. [PubMed: 2993460]
- 85.
- Fadok VA, Voelker DR, Campbell PA. et al. The ability to recognize phosphatidylserine on apoptotic cells is an inducible function in murine bone marrow-derived macrophages. Chest. 1993;103:102S. [PubMed: 8381344]
- 86.
- Newman SL, Henson JE, Henson PM. Phagocytosis of senescent neutrophils by human monocyte-derived macrophages and rabbit inflammatory macrophages. J Exp Med. 1982;156:430–42. [PMC free article: PMC2186761] [PubMed: 7097159]
- 87.
- Valledor AF, Borras FE, Cullell-Young M. et al. Transcription factors that regulate monocyte/macrophage differentiation. J Leukoc Biol. 1998;63:405–17. [PubMed: 9544570]
- 88.
- Melendez AJ, Harnett MM, Allen JM. Differentiation-dependent switch in protein kinase C isoenzyme activation by FcγRI, the human high-affinity receptor for Immunoglobulin G. Immunol. 1999;96:457–464. [PMC free article: PMC2326762] [PubMed: 10233728]
- 89.
- Melendez AJ, Harnett MM, Allen JM. FcγRI activation of phospholipase Cγ1 and protein kinase C in dibutyryl cAMP-differentiated U937 cells is dependent solely on the tyrosine-kinase activated form of phosphatidylinositol 3-kinase. Immunol. 1999;98:1–8. [PMC free article: PMC2326909] [PubMed: 10469227]
- 90.
- Lennartz MR, Brown EJ. Arachidonic acid is essential for IgG Fc receptor-mediated phagocytosis by human monocytes. J Immunol. 1991;147:621–626. [PubMed: 1830070]
- 91.
- Lennartz MR, Yuen AFC, McKenzie Masi S. et al. Phospholipase A2 inhibition results in sequestration of plasma membrane into electronlucent vesicles during IgG-mediated phagocytosis. J Cell Sci. 1997;110:2041–2052. [PubMed: 9378755]
- 92.
- Karimi K, Lennartz MR. Mitogen-activated protein kinase is activated during IgG-mediated phagocytosis, but it is not required for target ingestion. Inflammation. 1998;22:67–82. [PubMed: 9484651]
- 93.
- Karimi K, Lennartz MR. Protein kinase C activation precedes arachidonic acid release during IgG-mediated phagocytosis. J Immunol. 1995;155:5786–5794. [PubMed: 7499867]
- 94.
- Hazan-Halevy I, Seger R, Levy R. The requirement of both extracellular regulated kinase and p38 mitogen-activated protein kinase for stimulation of cytosolic phospholipase A2 activity by either FcγRIIA or FcγRIIIB in human neutrophils: A possible role of Pyk2 but not for the Grb-2-Sos-Shc complex. J Biol Chem. 2000;275:12416–12423. [PubMed: 10777525]
- 95.
- Gijón MA, Spencer DM, Siddiqi AR. et al. Cytosolic phospholipase A2 is required for macrophage arachidonic acid release by agonists that do and do not mobilize calcium: Novel role of mitogen-activated protein kinase pathways in cytosolic phosholipase A2 regulation. J Biol Chem. 2000;275:20146–20156. [PubMed: 10867029]
- 96.
- Akiba S, Mizunaga S, Kume K. et al. Involvement of group VI Ca2+-independent phospholipase A2 in protein kinase C-dependent arachidonic acid liberation in zymosan-stimulated macrophage-like P388D1 cells. J Biol Chem. 1999;274:19906–19912. [PubMed: 10391937]
- Adding Complexity to Phagocytic Signaling: Phagocytosis-Associated Cell Response...Adding Complexity to Phagocytic Signaling: Phagocytosis-Associated Cell Responses and Phagocytic Efficiency - Madame Curie Bioscience Database
- Asparaginyl-tRNA Synthetases - Madame Curie Bioscience DatabaseAsparaginyl-tRNA Synthetases - Madame Curie Bioscience Database
- Therapies of Nonsense-Associated Diseases - Madame Curie Bioscience DatabaseTherapies of Nonsense-Associated Diseases - Madame Curie Bioscience Database
- Coronin: The Double-Edged Sword of Actin Dynamics - Madame Curie Bioscience Data...Coronin: The Double-Edged Sword of Actin Dynamics - Madame Curie Bioscience Database
- PARP and the Release of Apoptosis-Inducing Factor from Mitochondria - Madame Cur...PARP and the Release of Apoptosis-Inducing Factor from Mitochondria - Madame Curie Bioscience Database
Your browsing activity is empty.
Activity recording is turned off.
See more...