NCBI Bookshelf. A service of the National Library of Medicine, National Institutes of Health.
Madame Curie Bioscience Database [Internet]. Austin (TX): Landes Bioscience; 2000-2013.
Until recently, structural information about coronins was scarce and the earlier identification of five WD40 repeats gave rise to a structural prediction of a five-bladed β propeller for the N-terminal domain of these proteins. More detailed analyses revealed the presence of seven WD40 repeats and the hypothesis of a seven-bladed β propeller structure. This model has recently been validated due to structural information from crystal structures of C-terminally truncated coronin 1 (1A), as well as its C-terminal coiled coil domain. Further structural information is available only indirectly from binding and functional studies.
Phosphorylation at distinct serine and tyrosine residues seems to be a common theme for various coronins. There are indications that this modification regulates the quaternary structure of coronin 3 (1C) and thus has implications for the cellular localisation and the general link between signalling and cytoskeletal remodelling. Similarly, phosphorylation-dependent sorting sequences recently discovered on coronin 7 might prove important for the molecular mechanisms of the longer coronins.
A matter that will require further clarification is the localisation of protein binding sites on coronins. While earlier reports presented a rather diverse map of actin binding sites, more recent studies, including the crystal structure of the coronin 1 N-terminal domain, deliver more detailed information in this respect. Interaction sites for other target proteins, such as Arp2/3, remain to be identified. Also, while membrane binding is a known feature of coronins, further details as to the binding sites and molecular level events remain to be elucidated. The N-terminal WD40 repeat domain seems to be the membrane-interacting domain, but other domains might provide regulatory effects, most likely by posttranslational modification, in a fashion that is specific for each coronin.
In this chapter, we provide a structural overview of coronins 1 (1A), 2 (1B), 3 (1C) and 7 and also present results of our recent efforts to obtain structural models of coronins 3 and 7. Possible implications of these models on the function of these proteins are discussed.
Introduction
Members of the family of coronin proteins are important for the downregulation of the Arp2/3 complex activity that controls F-actin assembly. Functional work so far has concentrated mainly on coronins 1 (1A), 2 (1B) and 3 (1C) and a significant amount of data has been accumulated yielding first insights into localisation and cellular functions of coronins.
While there are two incomplete nomenclatures used in the literature, either differentiating three subclasses of coronins,1 or a numbering scheme from 1 to 7,2 we have arbitrarily chosen to use the latter scheme for the purpose of this review. Based on amino acid sequence lengths, coronins can be divided into two classes. Coronins 1-6 are comprised of three domains, whereas mammalian coronin 7 and the POD-1 proteins from Caenorhabditis elegans and Drosophila melanogaster seem to represent tandem repeats of the short coronin topology.2 The three-domain topology (see Fig. 1) comprises an N-terminal domain containing WD40 repeats, a linker domain consisting of a C-terminal extension and a unique region, as well as a C-terminal domain that forms a coiled coil structure in some members of the family. Initially, the N-terminal domain was thought to consist of five WD40 repeats.3 The β subunit of G proteins is the “prototype” WD40 repeat protein and, accordingly, the structure of a five-bladed β propeller has been anticipated for coronins. More recent molecular modelling efforts, however, suggested that seven WD40 repeats might be encoded in the N-terminal domain and the first structure of a coronin N-terminal domain by Appleton and coworkers confirmed this hypothesis.4 Preceding the WD40 repeat region is a rather short coronin signature motif composed of a dodeca-peptide with conserved basic residues (see Fig. 2, 3).2 The N-terminal domain is followed by the C-terminal extension and a unique region that differs in length and sequence among different coronins. The C-terminal domain is constituted by a moderately conserved coiled coil, which is of the leucine zipper type in coronin 1 (1A). The coiled coil domain is responsible for homo-oligomerisation observed with several coronin proteins.
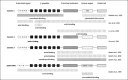
Figure 1
Domain organisation of coronins. Mapped binding sites for yeast coronin (crn1p), as well as mammalian coronins 1 (1A), 2 (1B) and 3 (1C) are indicated.
This chapter will discuss the structural aspects of coronins and possible implications for its molecular functions. Structural information about coronins is in high demand and the recent crystal structure of a coronin 1 (1A) construct allowed first valuable insights into the building principles and three-dimensional organisation of coronins. However, as with many proteins involved in complex pathways, further structural details are required to dissect and understand the molecular workings and interactions with target proteins. Therefore, this chapter is also a reflection of “work in progress” and some new results from molecular modelling are presented.
WD Repeat
The WD repeat motif has first been identified in the β subunit of the GTP-binding protein transducin and been referred to as transducin repeat, GH-WD repeat, or WD40 repeat.5-7 A single WD repeat comprises a peptide sequence of 44-60 residues length. The canonical repeat ends with the W-D motif and possesses a G-H sequence about 11-24 residues from its N-terminus.6 This ‘structural tetrad’ engages in side chain-mediated hydrogen bonding patterns, thereby stabilising the fold. Between the G-H and the W-D motifs, a conserved core sequence has been identified. Hydrophobic residues in this conserved sequence contribute to the stability of the overall fold by van der Waals-interactions between adjacent blades. WD repeat sequences form a secondary structure motif of four consecutive β-strands (D, A, B, C), connected by loops and turns. Crystal structures of the β subunits of G proteins define the prototype WD40 protein structures as β propellers.8,9 Within the propeller, the blades are arranged in a circular fashion around a central axis. Each blade is made up by four anti-parallel β-strands extending from the centre of propeller to the periphery. Structurally, strand A forms the first and innermost β-strand of a blade. The outermost strand D is provided by the first strand of the next WD repeat.
Earlier Predictions
Earlier structure predictions with coronins resulted in models of five-bladed β propellers, because only five WD repeats were recognised.3,10 However, many β propeller structures contain WD repeats with little or no obvious sequence homology to the canonical sequence. Additionally, while typical WD repeats possess four to eight copies and up to 16 copies have been reported,11,12 almost all structures of WD repeat proteins in the PDB contain seven blades per propeller. Keeping this in mind, more detailed analyses of coronins have been carried out and additional WD-like repeats were identified based on secondary structure prediction from amino acid sequences, indicating the presence of seven copies for coronins 1(1A)13,14 and 3 (1C)14 and 14 for coronin 7.14 The fold of a seven-bladed propeller was then confirmed by the crystal structure of a coronin 1 (1A) fragment.4
Overall, the WD repeats of coronins show substitutions for the canonical W-D of the structural tetrad, such as Y-E, F-D, L-E, I-E, I-D or W-N. While these substitutions can be considered conservative, others, such as W-R, Y-R or L-R, present a change of electrostatics. Other substitutions such as I-I or I-A seem far of the W-D motif.
Experimental Structures: Coronin 1 N-Terminal and Middle Domain
The first structural information for a coronin construct consisting of the N-terminal domain and the C-terminal extension became available with the crystal structure of coronin 1 (1A) by Appleton and coworkers.4 Interestingly, despite expressing the full-length murine coronin 1 (1A) in a eukaryotic expression system, these authors found the protein to be proteolytically degraded thus yielding a construct that lacked the C-terminal coiled coil domain. The crystal structure revealed a seven-bladed β-propeller topology for the N-terminal WD repeat domain and a C-terminal domain tightly packed against the bottom side of the propeller. The D strand of blade 7 is provided by the N-terminal residues 17 to 31. Despite the structural tetrad being strictly conserved in blades 2 and 3 only, blades 2 to 6 also possess secondary structures that fit the requirements of the β propeller fold. These blades also correspond to the predicted five WD repeats of coronin 1. Blades 1 and 7 are rather atypical in that their W-D motif is substituted by L-P and I-A and their fold shows some divergence from the standard blade. In blade 1, some residues that normally form strand D bulge out and connect to strand C with β-type interactions only at two positions. The D strand of blade 7 consists of two β-strands due to an insertion of six amino acids with a single helical turn in the connecting linker. Some variations in the lengths of strands B and C of blade 7 further add to the structural divergence of this blade from the standard WD40 blade.
It has been known for some time that coronins possess two stretches of conserved amino acid sequence in the C-terminal extension. In the case of coronin 1 (1A), these regions span residues 353-367 and 372-387. The structure by Appleton and coworkers4 highlighted the distinct roles of the highly conserved residues of Tyr364 and Trp 379. Both residues anchor their side chains into hydrophobic pockets on opposite sides of the bottom surface of the N-terminal β propeller domain, thus stabilising the fold of the C-terminal extension. This domain adopts a rather unstructured conformation and packs against the β propeller due to the high degree of shape complementarity to the N-terminal domain. A network of electrostatic interactions and hydrogen bonds, as well as a hydrophobic interface ensure the strong interactions between both domains.
While there is no structural information on coronin constructs that only contain the N-terminal WD repeat (propeller) domain, severe aggregation has been reported for coronin 1 (1A) truncation mutants lacking the C-terminal extension and the coiled coil domain.13 The crystal structure of coronin 1 (1A)4 implies an eminently important role of the C-terminal extension domain for the stability of the protein, especially for the residues directly interacting with the β propeller. Appleton et al.4 have thus put forward the hypothesis that the region 1-392 of coronin 1 (1A), which comprises the WD repeat domain and the C-terminal extension, represents a folding unit, albeit this awaits experimental validation. Notably, a construct of coronin 3 (1C) extending from the last blade of the β propeller to the coiled coil domain (300-474), possesses secondary structure as predicted and thus appears to be folded.15 It still remains a matter of speculation, whether blade 7 adopts its native conformation within this construct because strand D is missing.
Experimental Structures: Coiled Coil Peptides
In the context of structural investigation of coiled coil motifs, the group of Steinmetz solved the crystal structure of the mouse coronin 1 (1A) C-terminal coiled coil domain.16 It is accepted in the field that homo-oligomerisation of coronins is mediated by the C-terminal coiled coil domain. While electron micrographs, as well as analytical ultracentrifugation, showed coronin 1 (1A) homo-trimerisation,13 prediction programs such as MultiCoil17 or Scorer18 suggest two-stranded structures. A study by Oku and coworkers also reported the presence of dimeric coronin 1 (1A) based on gel filtration and sucrose density centrifugation19 and therefore is at odds with the generally accepted view. It has been suggested that their results might have been biased due to shape-dependency of the methods used.
Coiled coil motifs usually show a characteristic repeat pattern of hydrophobic amino acids spaced four and then three residues apart. This results in a hepta-peptide repeat (abcdefg)n, where positions a and d are predominantly hydrophobic residues and positions e and g are charged residues. Coronin 1 (1A) possesses a threefold repeat of such a characteristic hepta-peptide sequence and the motif R-L/I/V-X-X-L/V-E (450-455 in coronin 1) has been identified as a mediator of trimerisation in coiled coils.16 The arginine residue (position 1) of one monomer engages in interactions with the glutamate residue (position 6) from a second monomer. The interaction is stabilised by further contacts of this arginine residue (position 1) with an aspartate residue of the second monomer at position 3. Overall, this network of interactions maintains a contact interface of three monomers.
Coronin 3 N-Terminal Domain
Coronin 3 (1C) belongs to the subfamily of the short coronins with a length of 474 amino acids. It is expressed in all tissues and performs roles in wound healing, protrusion formation including lamellipodia, filopodia and axonal growth, migration, invasion, cell proliferation, cytokinesis, endocytosis, secretion of norepinephrine and matrix metalloproteinases.15,20 Localised to the leading edges of a cell and the sub-membranous cytoskeleton, it acts by bundling F-actin and crosslinking the Arp2/3 complex and cofilin.15,21,22 Based on the crystal structures of coronin 1 (1A) (8-402)4 and the three-stranded coiled coil domain (430-461),16 homology models for these domains in coronin 3 (1C) have been generated.15,23
Most likely, coronin 3 (1C) seems to exhibit the same feature as coronin 1 (1A) with the first N-terminal β-strand completing the last propeller blade of the WD40 repeat domain.15 Intra-molecular interactions might stabilise the C-terminal extension by anchoring it to the WD40 β-propeller. The main contributors to these interactions are hydrogen bonds of Tyr362 to the back- bone carbonyl groups of Val327 and Glu331 and the side chain interactions of the N-terminal Lys9 with Arg352 and Asp360. Between the WD40 repeat domain and the C-terminal extension, there is also a hydrophobic interface comprising residues His139, Ile157, Trp182, Ile189 and Trp377 and Phe378. The high degree of conservation between both coronins in these regions underpins the potential significance of these interactions. The potential F-actin binding sites identified in coronin 1 (1A)4 seem highly probable in coronin 3 (1C), including a region on the top of blades 1, 6, 7 and a second region on the bottom side of blades 6 and 7. A stretch of positively charged amino acids is also present in the C-terminal extension (398-419).15
An amino acid alignment of coronin 3 (1C) and coronin 1 (1A) generated before these models have been available, identified the peptide of 308-349 as seventh WD40 repeat14 and gave rise to two truncation mutants comprising peptides spanning (i) the last WD40 repeat and the entire C-terminal part of coronin 3 (300-474) and (ii) the last WD40 repeat and the C-terminal extension domain (315-444). CD spectra for both constructs show the of secondary structure as expected from structure prediction.15
An interesting observation in this context is the fact that these truncation mutants of coronin 3 seem to adopt functional structures. Both constructs have been shown to bind, bundle and crosslink F-actin in in vitro studies. The same activity has been found with coronin 3 (300-474), the construct described above.21,22
Coronin 3 Coiled Coil Domain: Effects of Phosphorylation
The extreme C-terminal region of coronin 3 (1C) is eight residues longer than that of coronin 1 (1A) and secondary structure prediction indicates a long helical segment (see Fig. 2). Furthermore, coronin 3 (1C) possesses a fourfold repeat of the characteristic coiled coil hepta-peptide sequence. Using the programme MultiCoil,17 the C-terminal domain of coronin 3 (1C) is predicted to form mainly homo-trimers (60% probability), but also homo-dimers (18% probability). Homo-trimers have also been observed in vitro.21
Using the crystal structure of the coronin 1 (1A) coiled coil trimer (PDB entry 2akf )16 and molecular graphics, a model of the three-stranded coronin 3 (1C) coiled coil has been generated.23 Like coronin 1 (1A), coronin 3 (1C) possesses the characteristic hexa-peptide motif R-L/I/V-X-X-L/V-E (461-466) proposed to be regulating trimerisation of coiled coil strands. Notably, while coronin 1 has Asp452 in position 3 of this motif, coronin 3 (1C) possesses Ser463 instead. Ser463 is a predicted phosphorylation site and the addition of a phosphate group to this residue will lead to disruption of the Arg461’-Glu466 interaction. Phosphorylation will require displacement of the Arg461' side chain and thus lead to the loss of the one of the coils. The remaining two-stranded coiled coil is probably stabilised by interactions arising from the reoriented Arg461’ side chain and possibly bridging effects from the posttranslational modification. Ser450 is part of a hexa-peptide insertion in coronin 3 (1C) that is not present in the coronin 1 (1A) coiled coil and can potentially be phosphorylated as well. While a role of (phosphorylated) Ser450 for di-/trimerisation cannot be excluded, it seems unlikely as judged by the current homology model. Ser450 is purely surface-exposed and does not seem to be involved in strategic interactions.
This mechanism is supported by results from gel filtration experiments with the phosphomimetic mutant Ser463Asp of a coronin 3 (1C) C-terminal peptide, as well as the full-length protein, which elute as a dimeric species. The wild type peptide and protein, in contrast, elute as a trimer in the size exclusion experiments.23 Also, phosphorylation at Ser463 regulates the oligomerisation state of coronin 3 (1C) and subsequently alters its cellular activity.
While phosphorylation of coronin 3 (1C) has been found to be correlated with its cytosolic location,21 cytosolic coronin 7 is not phosphorylated; the membrane-associated pool of coronin 7, however, is phosphorylated at tyrosine residues.24 A similar situation is found with coronin 1, where dissociation from phagocytotic vacuoles is dependent on the protein being phosphorylated.25 For coronin 2 (1B), a partial redistribution from vesicular structures to the leading edge of actin-rich filopodia has been reported upon phosphorylation by PKC on Ser2, a residue present in coronin 1 (1A), but not coronins 3 (1C)and 7.26,27 The modification also disrupts the interaction of the protein with the Arp2/3 complex.
Actin Binding Site
Actin binding can be considered the landmark features of coronins as they are collectively defined as F-actin-associated proteins.3 The common theme appears to be that coronins possess an actin binding site in the C-terminal domain, although further details remain to be clarified (see Fig. 1). For coronin 3 (1C), constructs comprising the last WD40 repeat and the entire C-terminal part, with (315-474) or without (315-444) the coiled coil domain, have been shown to bind and crosslink F-actin.21 Different studies for mammalian coronin 1 (1A) agree on the finding that the C-terminal part possesses a binding site for actin.13,28,29 The smallest common region in this context has been described by Gatfield and coworkers to be the region from 400 to 416 which is in the unique part of the C-terminal domain of coronin 1 (1A) and contains a patch of basic amino acids.13 Many actin binding proteins use such a feature to form favourable electrostatic interactions with acidic regions on F-actin.30,31 Oku and coworkers have also described actin-binding properties of peptides in the N-terminal domain of mammalian coronin 1 (1A), namely the very N-terminal region (signature motif ) and a region spanning propeller blades 2 and 3.29 One study concerned with yeast coronin (crn1p) mapped the actin binding property to the N-terminal domain with a peptide spanning the residues 1-400. At the same time, C-terminal constructs of yeast coronin did not show any actin binding behaviour.32
The crystal structure of the coronin 1 (1A) N-terminal and C-terminal extension domains provides some insights into the apparent confusion. Appleton and coworkers4 identified two patches conserved among the short coronins that might be involved in actin binding. One patch is formed by residues from propeller blades 1, 6 and 7 on the top face of the WD40 repeat domain. The other patch is located on the bottom face and is made up by residues from blades 6 and 7, as well as from the C-terminal extension domain.4
Very recently, a study concerned with coronin 2 (1B) revealed a crucial role of Arg30 in F-actin binding.33 Consistent with an important role for interactions between coronin 2 (1B)and F-actin in vivo, an Arg30Asp mutant does not bind F-actin and localises to the leading edge only inefficiently. The authors show that this residue selectively impairs the actin binding step, since interactions with Arp2/3 and others are identical to the wild type protein. An exciting result from co-immunoprecipitation using the Arg30Asp mutant as a tool suggests that it is the actin binding that increases the affinity of coronin 2 (1B) for Arp2/3.33 Interestingly, Arg30 is part of the coronin signature motif 2 and, accordingly, a homology model of coronin 2 (1B) showing this residue surface-exposed and not involved in any other interactions is presented.33 The residue is in vicinity of Lys73 and both residues have been shown to impair F-actin bundling. The basic patch on the surface of coronin 2 (1B) can putatively act as actin binding site and the conservation of these residues in coronins 1(1A) and 3 (1C) imply that the other short coronins also possess the same mechanism.
While any detailed model awaits experimental evidence, it is tempting to speculate that two separate actin binding sites could fulfill a role of coronins in F-actin crosslinking. On the other hand, it is still not undoubtedly clear whether the N- and C-terminal domains each harbour an actin binding site or whether one of them (most likely the one on the C-terminal side) acts as a regulatory element. Furthermore, it is not yet proven whether Arg3 0 in coronin 2 (1B) binds actin directly or actually interacts with the nucleotide.
For coronin 7, actin binding has not been observed to date.24 While it is difficult to explain this absence on the basis of the available structural models, it seems possible that the two anticipated actin-binding patches identified in the crystal structure of coronin 1(1A) are not accessible in the same way in coronin 7 due to inter-domain contacts between coronin 7a and 7b. Arg30, the residue critical for actin binding in coronin 2 (1B), is conserved in coronin 7a, but not 7b, albeit we hypothesise it to be located in a different structural element (see Figs. 2 and 3). Lys67 in coronin 7a is a conserved lysine residue in coronins 1(1A) and 3 (1C), but not coronin 7b (Tr536) and has also been reported to be involved in actin binding of coronin 2 (1B).33 The structural localisation of this lysine residue in coronin 7a might be conserved according to available models (Figs. 2 and 3), but this residue might only be functional in actin binding when in close vicinity to Arg30.
Association with Plasma Membrane
To date, no binding partners of coronins at the plasma membrane have been identified. The common function of the β propeller fold of WD repeat proteins is that of a binding platform yielding complexes by coordinating sequential or simultaneous interactions with binding proteins.6 Because the coiled coil domain is involved in homo-oligomerisation and possibly binding to the Arp2/3 complex (see below) and the C-terminal extension domain might bind F-actin, one could assume that the membrane-associated functions reside on the β propeller domains of coronins. Notably, this might have implications on the N-terminal actin binding site, if the sites of interaction with the membrane are identical or in close vicinity to the actin-binding patch. However, the scenario is supported by the observation that an N-terminal domain construct of coronin 1 (1A) shows plasma membrane binding in the absence of the unique C-terminal and the coiled coil domains (see Fig. 1).13 One would thus assume that plasma membrane association of coronin 1 does not require a trimeric species, but this remains to be proven by further experimental data. In an earlier study, these authors have suggested that binding partners can include integral and peripheral membrane proteins, as well as phospholipid moieties themselves.34
A different situation has been found with coronin 3 (1C), where the deletion of the coiled coil domain abolishes plasma membrane binding.22 Taking into account the role of the coiled coil domain for homo-oligomerisation and the fact that phosphorylation in this domain can alter the oligomerisation state, a further twist becomes apparent. Phosphorylation of coronin 3 (1C) leads to a cytosolic relocation of coronin 3 (1C), i.e., loss of association with the plasma membrane.21 One could conclude that the membrane association of coronin 3 (1C) is also mediated by the β propeller domain, but that this only occurs with the trimeric species. In the absence of experimental data, other binding sites in the C-terminal domain of coronin 3 (1C) cannot be excluded, though.
Intra- Versus Inter-Molecular Coronin Interactions
In vivo and in vitro co-immunoprecipitation studies with several coronin 3 (1C) constructs lead to the observation that the N-terminal peptide 1-71 binds to a region in the C-terminal domain (315-444).21 The site at the C-terminal domain is close to or overlaps with the actin binding site, since markedly reduced F-actin cosedimentation was also observed for certain combinations of coronin 3 (1C) truncation constructs. While the binding was observed as an inter-molecular interaction, it is in principle possible that, physiologically, this is in fact an intra-molecular interaction. In light of the crystal structure of coronin 1 (1A) and the derived models for coronin 3 (1C), there is further weight to the notion that these interactions came about as artefacts from the topology of the different constructs. Because all truncation constructs in that study, as well as in most other coronin studies to date, are sequential, but the β propeller topology is not, it might prove difficult to obtain ‘physiological’ results with correctly folded peptides. For example, a plausible scenario for an interaction study of coronin 3 (1-72) with coronin 3 (315-444) might be that the N-terminal domain peptide provides a β-strand for completion of the last propeller blade in the C-terminal construct. Obviously, like many other aspects of coronin structure and function, the question of possible coronin interactions other than the coiled coil oligomerisation would benefit from further structural data.
Coronin Protein-Protein Interactions
Apart from F-actin, the Arp2/3 complex has been identified as a binding partner for coronins35 and the binding site has been mapped to the coiled coil region in yeast coronin (crn1p).36 Coronin constructs missing the coiled coil domain do not bind to the Arp2/3 complex.4 Recently, the structure of the yeast Arp2/3 complex has been investigated by electron microscopy and density in a distance of about 20 Å from the core complex has been assigned to the β propeller of coronin.37 The distance has been explained by the long C-terminal extension domain and unique region of yeast coronin and the arrangement thus fits the model of the coiled coil domain interacting with the complex. The structure also shows that coronin stabilises an inactive conformation of the Arp2/3 complex which agrees with functional data from polymerisation assays, where the protein inhibits the Arp2/3-mediated F-actin nucleation.37
Coronin 2 (1B) has also been found to be colocalised with the Arp2/3 complex in a variety of cells.26 The interaction of coronin 2 with Arp2/3 is phosphorylation-dependent and Ser2, located in the very N-terminal region has been identified as the crucial site of regulation by PKC in this context. Consistent with the functional data from coronin 1 (1A), a nonphosphorylatable mutant of coronin 2 (1B), Ser2Ala, shows enhanced interactions with the Arp2/3 complex and promotes hypermotility in cellular assays to a greater extent than the wild type protein.26 In line with these findings, the phospho-mimetic Ser2Asp mutant of coronin 2 (1B) displayed weaker interactions with Arp2/3 and suppressed cell speed relative to the wild type.26 The same findings were obtained with the Ser2Asp mutant of coronin 1 (1A) investigated in a coronin 1 (1A) knock out mouse.38 The functional paradox of these findings is striking: Coronin acts as a molecular inhibitor of Arp2/3 by stabilising an inactive form of the complex. At the cellular level, however, this turns out to be an activating process for Arp2/3-dependent processes such as cellular motility. Further studies will be required to shine light on these complex interconnections.
Since the Arp2/3 binding site on coronin 2 (1B) is presumably located in the C-terminal region of the protein just like in other coronins, it remains to be clarified which molecular mechanisms are responsible for coupling the modification of Ser2 in the N-terminal region to the Arp2/3 binding site in the C-terminal domain.
Very recently, slingshot phosphatase (SSH1L), a regulator of actin filament formation that acts on ADF/coflin, has been shown to bind to coronin 2 (1B) simultaneously with the Arp2/3 complex. In a tandem co-immunoprecipitation experiment, Cai and coworkers could demonstrate that all three components are present in one complex and coronin 2 (1B) bridges SSH1L to the Arp2/3 complex.39 Furthermore, the study shows that coronin 2 (1B) is also a substrate of slingshot phosphatase and the phosphatase seems to be specific for coronin and cofilin in vivo, as no dephosphorylation of other focal adhesion-associated proteins (Erk1/2, paxillin) has been observed. While dephosphorylation and complex formation provide mechanistic details about the inhibitory effect of coronin 2 (1B) on Arp2/3-mediated actin nucleation, another important aspect became apparent in that study. Coronin 2 (1B) seems to target SSH1L to the leading edge of lamellipodia, as well as mediate cofilin activity via dephosphorylation by SSH1L.
Homology Models of Coronin 7
The subfamily of long coronins is constituted by four proteins: POD-1 from Caenorhabditis elegans,40 Dpod-1 from Drosophila melanogaster,41 the mammalian coronin 7,24 as well as villidin, a long chimeric coronin-like protein.42 These proteins are about twice as long as their shorter relatives and it is assumed that the corresponding genes arose due to gene duplication. The long coronins contain two copies of the basic coronin domain structure, i.e., two WD repeat domains, two C-terminal extension domains, but both copies lack the coiled coil segments. It is assumed that the dimer character of the long coronins renders further oligomerisation of these proteins unnecessary1 The structural and functional significance of the domain organisation in the long coronins remains unknown. For Dpod-1, a microtubule-binding domain has been predicted similar to that of the microtubule-binding protein MAP1B.
Mammalian coronin 7 is the first member of this family that has been localised to the Golgi and is believed to execute membrane trafficking-related functions; however, there is no indication for a Golgilocalisation of the worm and fly PODs.24 In contrast to cytosolic coronin 3 (1C), cytosolic coronin 7 is not phosphorylated. However, the fact that membrane-associated coronin 7 is phosphorylated at tyrosine residues makes it a plausible assumption that the protein relocates from the cytosol to the membrane upon phosphorylation.24 Two tyrosine-based sorting signals recognised by the μsubunits of clathrin assembly protein 1 (AP-1) have been identified in coronin 7. Peptides containing the Y-X-X-Φ motifs at Tyr288 and Tyr758, respectively, have been found to bind to AP-1 and coronin 7 has been shown to coprecipitate with AP-1.43 Kinase src binds to coronin 7 and both tyrosines 288 and 758 are specifically phosphorylated by src. Tyrosine 758 however appears as the major phosphorylation site of src, which thus may be a key regulator of coronin 7.44
Two different models of coronin 7 structural organisation can be envisioned: One model is based on alignment with coronins 1 (1A) and 3 (1C) (see Fig. 2), the other is based on an alignment with actin-interacting protein 1 (AIP-1; see Fig. 3). AIP-1 is a WD repeat protein that enhances actin filament disassembly in the presence of actin-depolymerising factor/cofilin. The sequence similarities of the two WD40 domains of human AIP-1 with the ones of coronin 7 are about 20%; the sequence similarities with C. elegans AIP-1 are 23% and 26%, respectively. We have chosen AIP-1 as a template for modelling coronin 7 structural organisation due to its structure having two β propeller domains in tandem.45
Based on the structures of coronin 1 (1A) and AIP-1, the main difference in topologies between the two derived coronin 7 models (see Fig. 4) is the involvement of the most N-terminal β-strand and, subsequently, the first β-strand after the C-terminal extension domain of coronin 7a in the formation of the β propeller structure. When analysing the secondary structure prediction of coronin 7a, β-strand topology is predicted for residues 32-34. One can adopt the view that this is not significant enough to make the first β-strand of blade 1, because all other coronins have a well conserved secondary structure element in this position with a length of four to six residues. A suggestion to reconcile could involve the first β-strand after the C-terminal extension of coronin 7a. The model based on AIP-1, however, makes use of the very N-terminal β-strand, thus not assigning a canonical β propeller function for the β-strand in the linker region between coronin 7a and 7b.
The second ambiguity in predicting a model of the tandem WD40 domains of coronin 7 is the role of the short β-strand in blade 7 of coronin 7b. In homology to coronin 1 (1A), this element would not participate in the canonical blade formation. The model based on AIP-1, on the other hand, makes use of that element which provides strand II.7B (see Fig. 3). Obviously, other combinations can be envisioned. Tree secondary structure elements, the most N-terminal β-strand, as well as two β-strands in the linker region, can potentially participate in any of the 14 blades as strand A or D.
In an attempt to learn about the putative conformation of the two β propellers of coronin 7, we compared the fold of AIP-1 (PDB entry 1nr0)45 with that of another tandem β propeller protein with known three-dimensional structure, namely Sro7 (PDB entry 2oaj).46 Sro7 is a regulator of polarised exocytosis and interacts with Q-SNARE proteins, thereby coordinating the actin cytoskeleton and the exocytic machinery responsible for fusion of secretory vesicles. The comparison indicates that there is considerable variability in the orientation of the two β propellers in tandem WD40 repeat domain structures (see Fig. 5). In both proteins, blades 7 and 1 of the first propeller are in close proximity to blades 1 and 7 of the second propeller. However, the elevations between the two propeller axes are about 45° for Sro7 and 60° for AIP-1. The azimuth between the two propeller axes is about 15° for Sro7 and -30° for AIP-1. While the two β propellers of the latter protein have several contacts between blades 1 and 7 of both propellers, the smaller elevation angle in Sro7 results in only blade 1 of the first propeller and blade 7 of the second propeller being in close proximity. In both proteins, it is the loop area between strands C and D of blade 1 of the first propeller that interacts with blade 7 of the second propeller. The evident variability of the orientation of propellers in tandem WD40 repeat domain structures adds to the complexity of any modelling endeavours for coronin 7 and calls upon experimental structures for clarification of structural details.
The N-terminal coronin signature motif R-X-X-K-X-R is present in coronin 7a only. The extended signature motif in coronins 1 and 3 of R-X-X-K-X-R-X7-K-X8-R finds a pendant in the sequence R-X-X-K-X-R-X7-R-X8-R in coronin 7a. While in coronin 1 (1A) this extension is located in strand D of blade 7 and Lys20/Arg29 are accessible on the surface of the propeller, a definite answer as to the location of this motif in coronin 7a cannot be given based on the current models (see Fig. 4). A model where this motif is not surface-accessible in coronin 7a would explain the observation that coronin 7 is not colocalising with actin. While coronin 7a shows a conservation of Arg27 and Lys67 that have been proven crucial for F-actin cable formation in coronin 2 (1B), the location of Arg27 is not the same as in coronin 1 (1A), since the N-terminal β-strand is not forming the strand D of the last propeller blade in neither of our two coronin 7 models (see Figs. 2, 3). Lys67, however, might be in the same location but seems to play a less important role in F-actin regulation.33 Coronin 7b has neither of the two basic residues conserved and is thus unlikely to possess this molecular mechanism.
The alignment of coronin 7 with coronins 1 (1A) and 3 (1C) allows some other interesting observations (see Fig. 2). While coronin 7 is not predicted to form any coiled coil structure, coronin 7b does seem to posses a C-terminal extension domain, albeit smaller than in other coronins. This assumption is based on the conserved helical segment in the linker region following the last propeller blade. Furthermore, the two aromatic residues involved in anchoring the C-terminal extension to the β propeller are conserved in both coronin 7 parts (Phe357, Trp372, Phe828, Trp844). Phe357 and Phe828 would be expected to engage in contacts with a V-X-X-X-E motif in the seventh propeller blade which is also conserved in coronin 7a and 7b, although the mechanism observed in coronin 1 (1A) involves hydrogen bonding from Tyr364 to the backbone carbonyls of Val327 and Glu331. The exchange of the tyrosine residue against a phenylalanine renders this type of interaction obsolete. Other residues involved in formation of this interaction interface are conserved to varying extents in coronin 7a and 7b (white on gray highlighted residues in Fig. 2). Trp379, the second anchor in the linker domain, is actually part of a W-Φ motif also conserved in all coronins 1, 3, 7a and 7b. This residue forms a hydrophobic interaction interface with various residues in blades 3 and 4 of the β propeller. While coronin 7a has several, but not all of these interface residues conserved, coronin 7b shows full conservation (white on black highlighted residues in Fig. 2). Taken together, this would allow the conclusion that an interface similar to that observed in coronin 1 (1A) can be expected for both coronin 7 propellers at the bottom faces.
Conclusion
Based on cell biological studies, as well as the frst crystal structure of a coronin 1 (1A) fragment, frst insights into structure and mechanisms of coronins have been obtained, mainly pointing out the features of the WD40 repeat domain and the importance of the C-terminal extension for the stability of the former. From a mechanistic point of view, the upcoming main questions are those of oligomerisation, actin binding and the features of coronin 7 which represents a tandem coronin monomer. With the recent identification of Arg30 on coronin 2 (1B) as an important regulator for F-actin bundling, a new tool for further investigation of the actin binding mechanisms of coronins has become available. At the molecular level, the events and localisations in this context remain to be identified. A model for the oligomerisation mechanism of coronin 3 (1C) has been put forward and is currently being validated by cell biological experiments. The area with the highest level of speculation is that of structure-function relationships of the long coronins, such as coronin 7. In this chapter, we have presented two possible models outlining structural parameters of these tandem WD40 repeat domain proteins. Aiding the further proceeding of this field, structural biology will be able to provide insights into the molecular functions of coronins and help define their roles in cell development.
Acknowledgements
AH gratefully acknowledges funding by Griffth University Research Grant, and an award from the European League Against Rheumatism (EULAR).
References
- 1.
- Uetrecht AC, Bear JE. Coronins: the return of the crown. Trends Cell Biol. 2006;16:421–426. [PubMed: 16806932]
- 2.
- Rybakin V, Clemen CS. Coronin proteins as multifunctional regulators of the cytoskeleton and membrane trafficking. BioEssays. 2005;27:625–632. [PubMed: 15892111]
- 3.
- de Hostos EL. The coronin family of actin-associated proteins. Trends Cell Biol. 1999;9:345–350. [PubMed: 10461187]
- 4.
- Appleton BA, Wu P, Wiesmann C. The crystal structure of murine coronin-1: a regulator of actin cytoskeletal dynamics in lymphocytes. Structure. 2006;14:87–96. [PubMed: 16407068]
- 5.
- Vander V, Ploegh HL. The WD-40 repeat. FEBS Lett. 1992;307:131–134. [PubMed: 1644165]
- 6.
- Smith TF, Gaitatzes C, Saxena K. et al. The WD repeat: a common architecture for diverse functions. Trends Biochem Sci. 1999;24:181–185. [PubMed: 10322433]
- 7.
- Neer EJ, Schmidt CJ, Nambudripad R. et al. The ancient regulatory-protein family of WD-repeat proteins. Nature. 1994;371 [PubMed: 8090199]
- 8.
- Wall MA, Coleman DE, Lee E. et al. The structure of the G protein heterotrimer Giα1β1γ2. Cell. 1995;83:1047–1058. [PubMed: 8521505]
- 9.
- Lambright DG, Sondek J, Bohm A. et al. The 2.0 Angstrom crystal structure of a heterotrimeric G protein. Nature. 1996;379 [PubMed: 8552184]
- 10.
- Suzuki K, Nishihata J, Arai Y. et al. Molecular cloning of a novel actin-binding protein, p57, with a WD repeat and a leucine zipper motif. FEBS Lett. 1995;364 [PubMed: 7758584]
- 11.
- Li D, Roberts R. WD-repeat proteins: structure characteristics, biological function and their involvement in human diseases. Cell Mol Life Sci. 2001;58:2085–2097. [PubMed: 11814058]
- 12.
- Yu L, Gaitatzes C, Neer EJ. et al. Thirty-plus functional families from a single motif. Protein Sci. 2000;9:2470–2476. [PMC free article: PMC2144505] [PubMed: 11206068]
- 13.
- Gatfield J, Albrecht I, Zanolari B. et al. Association of the leukocyte plasma membrane with the actin cytoskeleton through coiled coil-mediated trimeric coronin 1 molecules. Mol Biol Cell. 2005;16:2786–2798. [PMC free article: PMC1142424] [PubMed: 15800061]
- 14.
- Clemen CS, Hofmann A. Unpublished .
- 15.
- Rosentreter A, Hofmann A, Xavier CP. et al. Coronin 3 involvement in F-actin-dependent processes at the cell cortex. Exp Cell Res. 2007;313:878–895. [PubMed: 17274980]
- 16.
- Kammerer RA, Kostrewa D, Progias P. et al. A conserved trimerization motif controls the topology of short coiled coils. Proc Natl Acad Sci. 2005;102:13891–13896. [PMC free article: PMC1236532] [PubMed: 16172398]
- 17.
- Wolf E, Kim PS, Berger B. MultiCoil: a program for predicting two- and three-stranded coiled coils. Prot Sci. 1997;6:1179–1189. [PMC free article: PMC2143730] [PubMed: 9194178]
- 18.
- Woolfson DN, Alber T. Predicting oligomerization states of coiled coils. Prot Sci. 1995;4:1596–1607. [PMC free article: PMC2143200] [PubMed: 8520486]
- 19.
- Oku T, Itoh S, Ishii R. et al. Homotypic dimerisation of the actin-binding protein p57/coronin-1 mediated by a leucine zipper motif in the C-terminal region. Biochem J. 2005;387:325–331. [PMC free article: PMC1134960] [PubMed: 15601263]
- 20.
- Thal DR, Xavier C-P, Rosentreter A. Expression of coronin 3 in diffuse gliomas is related to malignancy. 2007. [PubMed: 18189330]
- 21.
- Spoerl Z, Stumpf M, Noegel AA. et al. Oligomerization, F-actin interaction and membrane association of the ubiquitous mammalian coronin 3 are mediated by its carboxyl terminus. J Biol Chem. 2002;277:48858–48867. [PubMed: 12377779]
- 22.
- Hasse A, Rosentreter A, Spoerl Z. et al. Coronin 3 and its role in murine brain morphogenesis. Eur J Neurosci. 2005;21:1155–1168. [PubMed: 15813925]
- 23.
- Xavier C-P, Rosentreter A, Hofmann A. et al. Phosphorylation regulates the quaternary structure and activity of coronin 3. Manuscript in preparation. 2007
- 24.
- Rybakin V, Stumpf M, Schulze A. et al. Coronin 7, the mammalian POD-1 homologue, localizes to the Golgi apparatus. FEBS Lett. 2004;573:161–167. [PubMed: 15327992]
- 25.
- Itoh S, Suzuki K, Nishihata J. et al. The role of protein kinase C in the transient association of p57, a coronin family actin-binding protein, with phagosomes. Biol Pharm Bull. 2002;25:837–844. [PubMed: 12132654]
- 26.
- Cai L, Holoweckyj N, Schaller MD. et al. Phosphorylation of coronin 1B by protein kinase C regulates interaction with Arp2/3 and cell motility. J Biol Chem. 2005;280:31913–31923. [PubMed: 16027158]
- 27.
- Parente JA, Chen X, Zhou C. et al. Isolation, cloning and characterization of a new mammalian coronin family member, coroninse, which is regulated within the protein kinase C signaling pathway. J Biol Chem. 1999;274:3017–3025. [PubMed: 9915840]
- 28.
- Liu CZ, Chen Y, Sui SF. The identification of a new actin-binding region in p57. Cell Res. 2006;16:106–112. [PubMed: 16467882]
- 29.
- Oku T, Itoh S, Okano M. et al. Two regions responsible for the actin binding of p57, a mammalian coronin familz actin-binding protein. Biol Pharm Bull. 2003;26:409–416. [PubMed: 12673016]
- 30.
- Tang JX, Janmey PA. The polyelectrolyte nature of F-actin and the mechanism of actin bundle formation. J Biol Chem. 1996;271:8556–8563. [PubMed: 8621482]
- 31.
- Amann KJ, Renley BA, Ervasti JM. A cluster of basic repeats in the dystrophin rod domain binds F-actin through an electrostatic interaction. J Biol Chem. 1998;273:28419–28423. [PubMed: 9774469]
- 32.
- Goode BL, Wong JJ, Butty AC. et al. Coronin promotes the rapid assembly and cross-linking of actin filaments and may link the actin and microtubule cytoskeletons in yeast. J Cell Biol. 1999;144:83–98. [PMC free article: PMC2148128] [PubMed: 9885246]
- 33.
- Cai L, Makhov AM, Bear JE. F-actin binding is essential for coronin 1B function in vivo. J Cell Sci. 2007;120:1779–1790. [PubMed: 17456547]
- 34.
- Gatfield J, Pieters J. Essential role for cholesterol in entry of mycobacteria into macrophages. Science. 2000;288:1647–1650. [PubMed: 10834844]
- 35.
- Machesky LM, Reeves E, Wientjes F. et al. Mammalian actin-related protein 2/3 complex localises to regions of lamellipodial protrusion and is composed of evolutionarily conserved proteins. Biochem J. 1997;328:105–112. [PMC free article: PMC1218893] [PubMed: 9359840]
- 36.
- Humphries CL, Balcer HI, D'Agostino JL. et al. Direct regulation of Arp2/3 complex activity and function by the actin binding protein coronin. J Cell Biol. 2002;159:993–1004. [PMC free article: PMC2173993] [PubMed: 12499356]
- 37.
- Rodal AA, Sokolova O, Robins DB. et al. Conformational changes in the Arp2/3 complex leading to actin nucleation. Nat Struct Mol Biol. 2005;12:26–31. [PubMed: 15592479]
- 38.
- Föger N, Rangell L, Danilenko DM. et al. Requirement for coronin 1 in T-lymphocyte trafficking and cellular homeostasis. Science. 2006;313:839–842. [PubMed: 16902139]
- 39.
- Cai L, Marshall TW, Uetrecht AC. et al. Coronin 1B coordinates Arp2/3 complex and cofilin activities at the leading edge. Cell. 2007;128:915–929. [PMC free article: PMC2630706] [PubMed: 17350576]
- 40.
- Rappleye CA, Paredez AR, Smith CW. et al. The coronin-like protein POD-1 is required for anteriorposterior axis formation and cellular architecture in the nematode Caenorhabditis elegans. Genes Dev. 1999;13:1838–1851. [PMC free article: PMC317117] [PubMed: 10557211]
- 41.
- Rothenberg ME, Rogers SL, Vale RD. et al. Drosophila pod-1 crosslinks both actin and microtubules and controls the targeting of axons. Neuron. 2003;39:779–791. [PubMed: 12948445]
- 42.
- Gloss A, Rivero F, Khaire N. et al. Villidin, a novel WD-repeat and villin-related protein from Dictyostelium, is associated with membranes and the cytoskeleton. Mol Biol Cell. 2003;14:2716–2727. [PMC free article: PMC165671] [PubMed: 12857859]
- 43.
- Rybakin V, Gounko NV, Spate K. et al. Crn7 interacts with AP-1 and is required for the maintenance of Golgi morphology and protein export from the Golgi. J Biol Chem. 2006;281:31070–31078. [PubMed: 16905771]
- 44.
- Rybakin V, Rastetter RH, Stumpf M. et al. Targeting of Crn7 to Golgi membranes requires the integrity of AP-1 complex, Src activity and presence of biosynthetic cargo 2007. submitted .
- 45.
- Mohri K, Vorobiev S, Fedorov AA. et al. Identification of functional residues on Caenorhabditis elegans actin-interacting protein 1 (UNC-78) for disassembly of actin depolymerizing factor/cofilin-bound actin filaments. J Biol Chem. 2004;279:31697–31707. [PubMed: 15150269]
- 46.
- Hattendorf DA, Andreeva A, Gangar A. et al. Structure of the yeast polarity protein Sro7 reveals a SNARE regulatory mechanism. Nature. 2007;446:567–571. [PubMed: 17392788]
- 47.
- Bryson K, McGuffin LJ, Marsden RL. et al. Protein structure prediction servers at University College London. Nucl Acids Res. 2005;33:W36–W38. [PMC free article: PMC1160171] [PubMed: 15980489]
- 48.
- Mohri K, Vorobiev S, Fedorov AA. et al. Identification of functional residues on Caenorhabditis elegans actin-interacting protein 1 (UNC-78) for disassembly of actin depolymerising factor/cofilin-bound actin filaments. J Biol Chem. 2004;279:31697–31707. [PubMed: 15150269]
- Introduction
- WD Repeat
- Earlier Predictions
- Experimental Structures: Coronin 1 N-Terminal and Middle Domain
- Experimental Structures: Coiled Coil Peptides
- Coronin 3 N-Terminal Domain
- Coronin 3 Coiled Coil Domain: Effects of Phosphorylation
- Actin Binding Site
- Association with Plasma Membrane
- Intra- Versus Inter-Molecular Coronin Interactions
- Coronin Protein-Protein Interactions
- Homology Models of Coronin 7
- Conclusion
- Acknowledgements
- References
- Coronin Structure and Implications - Madame Curie Bioscience DatabaseCoronin Structure and Implications - Madame Curie Bioscience Database
- Matrix Metalloproteases and Epithelial-to-Mesenchymal Transition: Implications f...Matrix Metalloproteases and Epithelial-to-Mesenchymal Transition: Implications for Carcinoma Metastasis - Madame Curie Bioscience Database
- Possible Roles of DNA Supercoiling in Transcription - Madame Curie Bioscience Da...Possible Roles of DNA Supercoiling in Transcription - Madame Curie Bioscience Database
- Proteases and Their Cognate Inhibitors of the Serine and Metalloprotease Subclas...Proteases and Their Cognate Inhibitors of the Serine and Metalloprotease Subclasses, in Testicular Physiology - Madame Curie Bioscience Database
- Roles of Poly(ADP-Ribose) Metabolism in the Regulation of Centrosome Duplication...Roles of Poly(ADP-Ribose) Metabolism in the Regulation of Centrosome Duplication and in the Maintenance of Neuronal Integrity - Madame Curie Bioscience Database
Your browsing activity is empty.
Activity recording is turned off.
See more...