NCBI Bookshelf. A service of the National Library of Medicine, National Institutes of Health.
Madame Curie Bioscience Database [Internet]. Austin (TX): Landes Bioscience; 2000-2013.
During the last two decades, efforts in the protein trafficking field have focused primarily on the identification of the machinery components of vesicular transport and mechanisms that underlie it. In addition, research has started to reveal how intracellular trafficking is regulated. Here, we summarize the current state of our knowledge about the regulation of vesicular transport and its coordination with other cellular processes. At the most basic level, individual transport steps are regulated spatially and temporally in two different ways. First, molecular switches of the Arf, Rab and Rho GTPase families regulate the assembly of components of the vesicular transport machinery on membranes, mediating the formation, targeting and fusion of vesicles that shuttle cargo between intracellular compartments. Second, reversible posttranslational modifications, like phosphorylation and ubiquitination, allow efficient cargo sorting and machinery component recycling. At a higher level, individual transport steps are integrated into whole pathways, with GTPases as a mechanism for this integration. Finally, intracellular trafficking pathways are coordinated with other cellular processes. Here too, GTPases appear to play a role by orchestrating coordination.
Introduction
Eukaryotic cells have a complex array of exocytic and endocytic membrane systems. Movement of membranes and cargos between organelles must occur efficiently while maintaining the integrity and structure of the organelles. Such maintenance requires sorting of proteins for forward transport while retaining resident proteins, as well as recycling of membranes and resident proteins back to donor organelles. Our current knowledge of the intracellular compartments and pathways are the subject of the first section of this book.1 Transport between organelles is mediated by membrane-bounded vesicles, which move membranes and proteins in both directions. Identification of the machinery components of vesicular transport and the mechanisms by which they function, the major issue the field has dealt with during the last two decades, is summarized in Section II. The progress made in these studies has made it possible to embark on the next major challenge in this field: understanding the spatial and temporal regulation of vesicular transport and the integration of individual transport steps into whole pathways in the context of the cell. This topic is the subject of Section III.
Two different mechanisms regulate individual vesicular transport steps. The first involves monomeric GTPases that act as molecular switches. These proteins regulate all aspects of vesicle life, from formation at the donor compartment to fusion with the acceptor compartment and are the subject of Chapter 16.2 The second type of regulatory mechanism uses posttranslational modifications, i.e., phosphorylation and ubiquitination of proteins. The best-characterized examples of this type of regulation occur in the endocytic pathway, where both endocytic cargo and endocytic machinery components are modified in a reversible way to allow cargo sorting and machinery component recycling. This type of regulation is discussed in Chapter 17.3
Individual transport steps require coordination to allow integration of the steps into whole pathways. Monomeric GTPases and their upstream regulators play a key role in this process too. GTPase cascades were shown to regulate other cellular processes,4 and there is growing evidence that such cascades act in intracellular trafficking as well.5,6
It has become clear that intracellular trafficking needs to be coordinated with other processes to allow for proper cell function. Evidence of such coordination is beginning to emerge and thee examples are discussed in Chapters 18-20. First, intracellular trafficking is important for polarized cell growth.7 Second, intracellular trafficking is crucial for proper signaling, with Rab GTPases playing a role in this coordination too.8 Finally, both exocytosis9 and endocytosis10 are required for development of multi-cellular organisms.
Here, we summarize what is currently known about the regulation of intracellular trafficking, its coordination with other processes and the importance of this regulation to human health, and we discuss future perspectives in this field.
Regulation of Individual Transport Steps
Components of the trafficking machinery cannot by themselves drive efficient vesicular transport. For example, specific SNARE combinations can drive synthetic membrane fusion; however, the fusion reaction is extremely slow.11 Two types of highly conserved regulations ensure that intracellular trafficking is a specific and efficient process: monomeric GTPases and posttranslational modifications of cargo and machinery components.
GTPases Regulating Individual Vesicular Transport Steps
Monomeric GTPases of the Arf, Rab, Rho and dynamin families control specific vesicular transport steps. GTPases in general act as molecular switches as they cycle between the inactive GDP-bound and the active GTP-bound forms. This switching is catalyzed by guanine-nucleotide exchange factors (GEFs) that activate the GTPases and by GTPase activating proteins (GAPs) that inactivate them. When in the active state, GTPases that regulate intracellular trafficking interact with downstream effectors. These effectors and their binding proteins mediate the various steps of vesicle life, from formation at the donor compartment to fusion with the acceptor compartment.2
Vesicle formation involves a number of processes: coat assembly, cargo sorting, membrane curvature, vesicle fission and vesicle un-coating. All these processes are regulated by GTPases (Fig. 1). Members of the Arf and Rho families regulate assembly of specific coats and coat adaptors required for cargo sorting. For example, Sar1, a member of the Arf family, recruits the ER coat COPII, Arf1 recruits the Golgi coat COPI, and Arf6 and Rho GTPases recruit the clathrin coat at the plasma membrane. Rabs were also suggested to function in vesicle formation and cargo sorting12-14, even though specific coats have not been implicated yet in Rab-mediated vesicle formation. Furthermore, protein coats induce membrane curvature into spherical buds. Therefore, the regulation of coat assembly and disassembly by GTPases plays a role in both cargo sorting and membrane curvature of budding vesicles.15 Vesicle fission at the neck is mediated by dynamin GTPases.16,17 Finally, Arfs and Rabs were implicated in vesicle un-coating in the exocytic and endocytic pathways, respectively.18,19
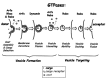
Figure 1
Regulation of individual vesicular transport steps by monomeric GTPases from the Arf, Rab, Rho and dynamin families (top).
Members of the Ypt/Rab GTPase family regulate all the steps that follow vesicle formation (Fig. 1). Because individual Ypt/Rabs can recruit multiple effectors, these GTPases can control processes as diverse as vesicle motility, tethering and fusion.20 Currently, Rabs are envisioned as organizers of membrane micro-domains, definers of compartment identity, and drivers of compartment maturation.21,22 These roles might explain the high number of Rabs (70 human Rabs) relative to Arfs (six human Arfs), which are involved only in vesicle formation. Indeed, a global genomic study suggests that Rabs define membrane identity, membrome, of different cell and organ systems.23
Progress has been made in recent years in the identification of upstream regulators of GTPases that control intracellular trafficking. For the Arf and Rho families, numerous GEFs and GAPs have been identified. All Arf GEFs contain a Sec7 domain, whereas Rho GEFs contain the Dbl homology (DH) domain; these domains comprise the catalytic core of the GEFs. There are numerous Arf GAPs that can be identified by a Zn-finger GAP domain in addition to other regulatory and protein-protein interaction domains. Similarly, a conserved Ypt/Rab GAP domain allows the identification of multiple Rab GAPs. In contrast, there is a paucity of identified Rab GEFs. The reason for this shortage is that the known Rab GEFs do not share similarity, which makes it harder to identify them. Interestingly, the number of GAPs for GTPases that regulate intracellular trafficking is higher than the number of GEFs. For example, many more mammalian GAPs for Arfs have been identified than Arfs themselves. This observation suggests that either GAPs are more cell- or stage-specific than the GTPases themselves, or that GAPs are also effectors that act in feedback inhibition of their GTPase recruiters.
The major open questions in the GTPase field concern the nature of the molecular mechanisms by which GTPases are regulated and how the GTPases control their downstream effectors. To this end, the full inventory of players is being identified in proteomic studies and molecular mechanisms are being determined in vitro using biochemistry and structural studies, as well as in vivo using knockdown experiments and expression of dominant negative mutations.
Posttranslational Modifications Regulating Cargo Sorting
Posttranslational modifications (PTMs) regulate sorting of membrane proteins en route to their degradation in lysosomes. This process is important for down regulation of plasma-membrane (PM) receptors and for quality control of membrane proteins in the Golgi and the PM. Two types of PTMs are known to regulate sorting of membrane proteins to endosomes: phosphorylation and ubiquitination.3 Signals for these PTMs are found in the cytoplasmic tails of the membrane proteins and on components of the PTM machinery.
Phosphorylation is required for internalization of a number of PM receptors, notably G-protein coupled receptors (GPCRs). In this case, protein kinases phosphorylate the cytoplasmic tails of receptors, resulting in a signal for interaction with arrestins. Arrestins, in turn, recruit the endocytic machinery, resulting in the internalization and down regulation of the receptors.24
Ubiquitin (Ub) is a highly conserved 76-amino acid polypeptide that can be attached covalently to lysines in other proteins and in Ub itself. Because Ub can be linked to itself, poly-ubiquitin chains can accumulate on cellular proteins. The ubiquitination reaction is carried by a set of enzymes that act successively, with the E3 ubiquitin ligase acting at the end of the reaction. The original role assigned to ubiquitination was for protein degradation in the cytoplasm by proteasomes. However, subsequently ubiquitin was shown to serve as a signal for sorting proteins into endosomes. On endosomes, the ESCRT (endosomal sorting complex required for transport) machinery assembles to target ubiquitinated proteins to intra-lumenal vesicles (ILVs) that bud into endosomes, forming multivesicular bodies (MVBs). Fusion of MVBs with lysosomes results in the degradation of the sequestered proteins.
One question that the field addressed is how the Ub signal that sends proteins for degradation in lysosomes is different from the signal that sends proteins to proteosomes. One suggestion was that the difference lies in the numbers of Ub ligands attached to the protein, mono-Ub for endocytosis en route to the lysosome and poly-Ub for sending proteins to the proteasome. Current thinking is that the difference lies not in the number, but in the type of poly-ubiquitination: Ub K48 for the proteosome and Ub K63 for sorting into MVBs. Endocytosis-related ubiquitination is performed by the conserved Rsp5/Nedd4 Ub ligase. This ligase recognizes PY motifs on the cytoplasmic tails of membrane proteins, or on adaptors that attach to these tails in the Golgi and the PM.
Both phosphorylation and ubiquitination are reversible PTMs. This reversibility might be required for cargo sorting to lysosomes, i.e., the Ub required for sending proteins to the lysosome has to be removed before the proteins enter this compartment. Alternatively, the reverse reactions might be important because machinery components that perform these PTMs are also modified. For example, the AP-2 chlathrin adaptors can be phosphorylated and the Ub-adaptors arrestins, as well as ESCRT subunits, can be ubiquitinated. In this scenario, reversible PTMs are important for the activity of the machinery components or for their recycling.
There are a number of open questions in this field. For example, it is not clear whether phosphorylation and ubiquitination are linked. A recent study suggests that arrestin-related proteins serve as ubiquitin ligase adaptors for PM proteins in yeast.25 Because arrestins can recognize the phosphorylated cytoplasmic tails of PM proteins, this finding suggests a link between the two PTMs, phosphorylation and ubiquitination. Other open questions are how ESCRT promotes the formation of ILVs and whether PTMs can sequester proteins into routes other than degradation, e.g., recycling to the PM.26
Transport Step Coordination
Coordination of individual transport steps can occur at two levels. First, each transport step between any two compartments, e.g., ER-to-Golgi or Golgi-to-PM, involves a number of vesicular transport steps: from vesicle packaging and formation, through its delivery and docking, to its final fusion with the acceptor compartment. These individual vesicular transport steps have to be coordinated. The second level involves integration of individual transport steps of the same pathway, e.g., the various steps of the exocytic and endocytic pathways. Evidence is emerging that monomeric GTPases play a role not only in the regulation of individual vesicular transport steps, but also in the step-integration process.
Coordination of Individual Vesicular Transport Steps
It makes sense that mechanisms exist for ensuring that a cargo-loaded vesicle that forms at any donor compartment has the capability to be targeted efficiently to the right acceptor compartment and fuse with it. Because monomeric GTPases regulate the multiple individual steps of vesicular transport, they are also obvious candidates for the integration process. The GTPase-dependent cooperation idea was first suggested for the integration of exocytic pathway steps based on genetic studies in yeast. Genetic interactions between Arf GEFs and Ypt/Rabs suggested coupling between Arf-dependent vesicle formation with Ypt/Rab-dependent vesicle targeting and fusion.27
More recently, a number of specific GTPase cascades that couple vesicular transport steps were described (Fig. 2). In the exocytic pathway, interaction of the Golgi coiled-coil protein p115 with the Arf GEF GBF1, Rab1 and SNAREs was suggested as a way for integrating ER-to-Golgi vesicle formation, tethering and fusion in mammalian cells.28 Another example of cooperation between two Ypt/Rab GTPases that regulate individual vesicular transport steps of Golgi-to-PM transport has also been shown in yeast. In this case, the Ypt31/32 functional pair required for Golgi vesicle formation and motility13,29 interacts with Sec2, a GEF for the Ypt/ Rab Sec4, which is required for the fusion of these vesicles with the PM.30
Two recent papers suggest cooperation between GTPases in both packaging and tethering of endosome-to-TGN vesicles. The first paper demonstrates that cooperation between Rab5 and Rab7 is required for recruitment of the two parts of the retomer complex. These two parts of the retromer are needed for formation of vesicles containing the mannose 6-phosphate receptor (MPR).31 A second paper identifies interaction of the golgin GCC185 with multiple GTPases, Rab9, Rab6 and Arl1, as a requirement for the tethering of MPR-containing vesicles to the TGN.32
Integration of Individual Transport Steps into Whole Pathways
To ensure unobstructed transport flow through a pathway as well as maintenance of compartment size, individual steps must be coordinated.6 Evidence of such coordination by monomeric GTPases and their GEF activators is beginning to emerge. One example is sequential activation of Ypt/Rab GTPases that regulate Golgi entry and exit in yeast by the modular GEF/tethering complex TRAPP.33 TRAPP is found on the Golgi in two confirmations: TRAPPI at the cis Golgi and TRAPPII at the trans Golgi. The finding that these two complexes act as GEFs for the Golgi Ypt/Rab gatekeepers, Ypt1 and Ypt31/32, raises the exciting possibility that sequential activation of the Ypt/Rabs coordinates Golgi entry and exit.34 Another example of a Rab cascade was suggested to drive endosome maturation. In this case, a conversion of early-to-late endosome is driven by Rab5 on early endosomes, recruiting the GEF for the late-endosome organizer Rab7.22
Coordination of Intracellular Trafficking with Other Cellular Processes
Proper cell function requires the coordination of all cellular processes, including intracellular traffic. This section describes the mechanisms by which intracellular trafficking is coordinated with cell polarization, efficient signal transduction and development.
Intracellular Trafficking and Cell Polarity
In polarized cells, compartments and functions are distributed asymmetrically. Therefore, during the establishment of cell polarity, PM symmetry has to be broken and the newly established asymmetry has to be maintained. In yeast, cell polarity is important for both asymmetric cell division,7 and for response to mating pheromones.35 In multi-cellular organisms, cell polarization is important for the functioning of polarized tissues; e.g., asymmetry of the apical and basolateral surfaces is required for epithelial cell function,36 asymmetry of the axonal and dendritic sides is important for neuronal cell function37 and asymmetry during stem cell division is crucial for their differentiation.38 Consequently, disturbance of cell polarity can result in cancer, problems in transmission of information in the brain and developmental abnormalities.
Establishment of cell polarity requires coordination between a number of cellular machineries (Fig. 3). First, polarity cues must be positioned on the PM in response to internal or external signals. Second, the polarity cues have to be decoded and the actin cytoskeleton and the exocytic pathway have to reorient towards these positional cues. Third, maintenance of cell polarity depends on microtubules.39 Exocytosis and endocytosis are required for breaking the cell symmetry and for maintaining the asymmetry.38,40
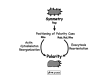
Figure 3
Coupling of intracellular trafficking with the establishment of cell polarity. Monomeric GTPases from the Rap, Rho, Ral and Rab families play key roles in all steps.
Monomeric GTPases play a role in the establishment of cell polarity and its coordination with intracellular trafficking (Fig. 3). These GTPases regulate the positioning of the cues, the reorientation of the actin cytoskeleton and the coordination between the actin cytoskeleton and the trafficking machinery. In budding yeast, a GTPase cascade plays a role in this coordination. The Rap GTPase Bud1 and its upstream regulators play a role in positioning cues for the emerging bud. Bud1 recruits another GTPase, Cdc42. The Rho GTPase Cdc42 and its downstream effectors are required for establishing a landmark for the actin cytoskeleton reorientation and polarized secretion.7 In the latter process, the exocyst complex, which serves as a tether between secretory vesicles and the PM, plays a role in the special regulation of exocytosis. Rab, Ral and Rho GTPases regulate the assembly and activation of the exocyst.41 All these components are conserved in all eukaryotes and the emerging basic mechanisms are similar in all eukaryotic cells.
Intracellular Trafficking and Signal Transduction
Transduction of external signals through the PM is essential for the interaction of cells with their environment. In this process, receptors present on the cell surface bind external ligands, such as growth factors, neurotransmitters or hormones, and transduce the signal to the inside of the cell. This process is crucial for the functioning of all cells, tissues and organs, and its disruption results in aberrant cell growth and function leading to human disease. Interdependence between intracellular trafficking and signaling is key for proper response to external signals (Fig. 4).
On the one hand, signal transduction also regulates endocytosis (Fig. 4). For example, a stimulated G-protein coupled receptor (GPCR) interacts with β-arrestin. This interaction induces the assembly of the endocytic machinery and internalization of the GPRC receptor. Activation of endocytosis by signaling is achieved through regulation of endocytic Rabs. For example, activation of the EGFR leads to the activation of Rab5 and stimulation of endocytosis, thus leading to internalization of the EGF receptor.8
Conversely, the endocytic pathway is required for signaling regulation. The established role for endocytosis in signaling is in the internalization of ligand-bound receptors, which serves as a mechanism for signaling down-regulation. Internalized receptors are delivered to early endosomes and then can be either recycled back to the PM for further signaling or transported to lysosomes for degradation. The balance between receptor recycling and degradation determines signaling amplitude and duration. Therefore, Rab GTPases that regulate endocytosis play an important role in the regulation of signal transduction (Fig. 4).
In addition, there is evidence that signaling events occur not only on the PM, but also on endosomes. For example, the internalized epidermal growth factor receptor (EGFR) remains active and associates with its downstream signaling molecules, like Shc, GRb2 and Sos, on endosomes.8 Moreover, some signaling events require endocytosis. For example, inhibition of endocytosis results in inhibition of some signaling pathways, like the PI3K and ERK1/2 pathways downstream of insulin receptors, but not others, like the insulin receptor Akt pathway.42 The functional importance of endocytosis for signaling was recently shown for the stimulation of cell migration by receptor tyrosine kinases (RTKs). Activation of RTKs results in a Rab5-dependent endocytosis of Rac GTPase to endososmes, where Rac is activated by its GEF Tiam1.43 Together, these findings imply that in addition to down-regulation, GTPase-dependent endocytosis plays a positive role in signaling (Fig. 4).
Intracellular Trafficking and Development
Development of multi-cellular organisms is regulated at the transcriptional level. However, cell-fate transcriptional regulation depends on the dynamic secretion of signals by some cells and on correct responses to these signals by receiving cells. Therefore, proper regulation of both exocytosis and endocytosis is as important for development as it is for any other process that depends on signaling (see above). In addition, development of some tissues, like epithelia, neurons, and stem cells, requires polarization that also depends on intracellular trafficking (see above). Therefore, a field that interfaces between development and intracellular trafficking has now blossomed. Of particular importance, because development engages multiple tissues and organs, this field involves an extra level of complexity; this complexity is discussed here.
The role of the endocytic pathway in development is well established. During cell-fate decision, gradients of signaling molecules, morphogens, are formed, and the slope of these gradients determine the signaling range. These gradients and their slope depend not only on diffusion, but also on vesicular trafficking of morphogens through cells. For example, formation of a gradient of the Drosophila TGF-β homologue Dpp depends on receptor-mediated endocytosis of this ligand. The slope of the Dpp gradient depends on the ratio between its sorting in endosomes for recycling to the PM or degradation in lysosomes.44 Therefore, endocytic Rabs that regulate this ratio play a key role in this process.
A role for exocytosis in development, including cellularization, establishment of polarized tissues and cell-fate determination, is beginning to emerge. During cellularization, the fertilized egg undergoes synchronous divisions to generate the primary epithilia. Exocytic compartments, like ER and Golgi, and vesicular transport components, like SNAREs, are required for this process. Establishment of polarized tissues, like epithilia and neurons, requires components of the secretory vesicle fusion machinery; e.g., the exocyst complex and PM SNAREs. Cell-fate determination depends on secretion of morphogens from source cells, as well as presentation of receptors on the PM of receiving cells. Both processes depend on exocytosis. For example, in Drosophila, the Wnt and Hedgedog morphogens are glycoproteins that also undergo acylation by the acyltransferases Procupine and Ski, respectively. This lipid modification occurs in the ER and is required for the secretion of these morphogens. In addition, a specific chaperone, Evi, is required for shuttling Wnt from the Gogli to the PM.9
Therefore, it is not surprising that there are multiple examples of mutations in genes encoding intracellular components and regulators that result in impaired development. A temperature-sensitive mutation in the Drosophila dynamin, shibire, allowed studying the effects of inhibition of endocytosis on development.10 Mutations in the exocytic machinery, like cargo receptors, vesicle coats, tethering factors and SNAREs, result in developmental defects in Drosophila, mice and humans. Finally, mutations in GTPases from the Arf/Sar1 and Rab families that regulate the endocytic and exocytic pathways also affect development.9
Traffic Regulation and Human Disease
Impairment of secretion of substances like hormones, antibodies and neuro-transmitters, defects in presentation of receptors on the plasma membrane, and obstruction of uptake of ligands from the environment can result in malfunctioning of various body systems and, therefore, can cause human diseases. Thus, it is expected that disruption of traffic regulation and coordination would result in human diseases as well. Because down-regulation of PM receptors and quality control of PM transporters and channels are important for the response of cells to environmental signals, the regulation of these processes by PTM has also been implicated in human disease. For example, dysfunction of the ESCRT machinery, which is required for targeting ubiquinated proteins into MVBs, was shown to contribute to cancer and neuro-degeneration.45
In the past few years, malfunctioning of trafficking GTPases and their upstream regulators were implicated in various human disorders. Because GTPases are expressed ubiquitously, it is reasonable that they would be involved in common multifactorial disorders. Indeed, Rabs, Arfs, Rhos and their associated proteins have been implicated in endocrinological diseases like diabetes,46 immunity disorders,47 cancer,48 heart disease,49 and brain disorders like Parkinson's.50 In addition, GTPases and their associated proteins were also implicated in rare monogenic diseases. This implication is probably due to differential expression of these regulators in specific tissues at specific times.23 Examples include: the ALS2 mutation in a Rab5 GEF is associated with the neurodegenerative disease ALS (Amyotrophic Lateral Sclerosis);51 mutations in Rab27 result in the rare Griscelli syndrome;52 Rab8 was implicated in Huntington disease;53 mutations in Rab25 were linked to cancer aggressiveness.54
Finally, infectious viruses, bacteria and other pathogens can take over the cell by altering the regulation of cellular trafficking for their purposes. Enveloped viruses, like HIV, exploit the ESCRT machinery for their budding.55 Other intracellular pathogens exploit GTPases or their regulators for their reproduction. Examples include: Legionella pneumophila recruit Arf during an early step of its pathogenesis using its own Arf GEF RalF;56 it also expresses its own Rab1 GEF, DrrA, the GAP LepB,57 and the GDF SidM,58 to recruit Rab1 to its membrane. TBC1D20 expressed by the Hepatitis C virus is a Rab1 GAP.59 The obligate pathogens Chlamydiae recruit key Rabs into their replication inclusion.60 Salmonella expresses SopE to recruit Rab5 to phagosomes as an evasion mechanism of transport into lysosomes.61 The HIV-1 gene HRB, required for viral replication, contains an ARF GAP domain.62 Another HIV-1 gene, Nef, induces Arf6-mediated endocytosis required for MHC-I down-regulation and viral immuno-evasion.63
In summary, better understanding of how unobstructed intracellular trafficking flow is achieved will directly impinge on our ability to treat human diseases caused by obstruction of this flow. We expect that in the near future GTPases and their associated proteins, as well as trafficking-specific PTM machinery, will emerge as drug intervention targets for both common and rare human diseases and pathogen infections.
Future Perspectives
The study of regulation of individual transport steps, their integration to whole pathways, and coordination of intracellular trafficking with other processes form the future challenge of the intracellular trafficking field. Whereas some cues are already available, unanswered questions abound. The most advanced among these research fronts to date is how GTPases and posttranslational modifications regulate individual transport steps. Understanding how GTPases and their upstream regulators integrate individual transport steps into whole pathways is just beginning to be unraveled. The field is just beginning to scratch the surface of how intracellular trafficking is coordinated with other cellular processes. The ultimate goal of the cell biology research is to understand how all the various processes are integrated to form an efficient living cell.
Why is it important to understand how intracellular trafficking is regulated? Unobstructed flow of proteins and membranes inside cells is crucial for proper functioning of all eukaryotic cells and therefore for all body processes. Malfunctioning of intracellular trafficking has been implicated in multiple human disorders, from rare monogenic disorders to common multifactorial diseases like diabetes, cancer, heart disease and degenerative brain disorders. Therefore, studying the regulation of this process is extremely important for human health and drug intervention in multiple diseases.
How will such questions be addressed in the future? No doubt, researchers in the field will employ traditional and continually improving cell biological, molecular, biochemical and genetic approaches to address questions of regulation and coordination. In addition, mounting information from structural analyses regarding structures of large protein complexes will help elucidate molecular interaction between trafficking components and regulators. Finally, emerging information from multiple global genomics and proteomics studies will be especially important for advancing our understanding of intracellular trafficking coordination with other cellular processes.
Acknowledgements
The authors thank Gregory Payne and Andrei Tokarev for critical reading of the manuscript, Andrei Tokarev for help with the figures, Eran Segev for text editing, Andrei Tokarev for help with the figures, and acknowledge support from National Institutes of Health GM45-444 to N.S. and the Division of Intramural Research of the National Heart, Lung, and Blood Institute, NIH to J.G.D.
References
- 1.
- Segev N, editor. Trafficking Inside Cells: Pathways, Mechanisms and Regulation. Austin/New York: Landes Biosciences/Springer Science+Business Media; 2009. pp. 1–102. this volume.
- 2.
- Franco M, Chavrier P, Niedergang F. Regulation of protein trafficking by GTP-binding proteins. In: Segev N, editor. Trafficking Inside Cells: Pathways, Mechanisms and Regulation. Austin/New York: Landes Biosciences/Springer Science+Business Media; 2009. pp. 342–62. this volume.
- 3.
- Piper R, Bryant N. Posttranslational control of protein trafficking in the post-Golgi secretory and endocytic pathway. In: Segev N, editor. Trafficking Inside Cells: Pathways, Mechanisms and Regulation. Austin/New York: Landes Biosciences/Springer Science+Business Media; 2009. pp. 363–87. this volume.
- 4.
- Chant J, Stowers L. GTPase cascades choreographing cellular behavior: movement, morphogenesis, and more. Cell. 1995;81(1):1–4. [PubMed: 7720065]
- 5.
- Markgraf DF, Peplowska K, Ungermann C. Rab cascades and tethering factors in the endomembrane system. FEBS Lett. 2007;581(11):2125–30. [PubMed: 17316615]
- 6.
- Segev N. Ypt/rab gtpases: regulators of protein trafficking. Sci STKE. 2001;2001(100):RE11. [PubMed: 11579231]
- 7.
- Osman M, Cerione R. Actin doesn't do the locomotion: Secretion drives cell polarization. In: Segev N, editor. Trafficking Inside Cells: Pathways, Mechanisms and Regulation. Austin/New York: Landes Biosciences/Springer Science+Business Media; 2009. pp. 388–404. this volume.
- 8.
- Barbieri M, Wainszelbaum M, Stahl P. Intracellular trafficking and signaling: The role of endocytic Rab GTPases. In: Segev N, editor. Trafficking Inside Cells: Pathways, Mechanisms and Regulation. Austin/New York: Landes Biosciences/Springer Science+Business Media; 2009. pp. 405–18. this volume.
- 9.
- Schotman H, Rabouille C. The exocytic pathway and development. In: Segev N, editor. Trafficking Inside Cells: Pathways, Mechanisms and Regulation. Austin/New York: Landes Biosciences/Springer Science+Business Media; 2009. pp. 419–38. this volume.
- 10.
- Dudu V, Pantazis P, Gonzalez-Gaitan M. Membrane traffic during embryonic development: epithelial formation, cell fate decisions and differentiation. Curr Opin Cell Biol. 2004;16(4):407–14. [PubMed: 15261673]
- 11.
- Weber T, Zemelman BV, McNew JA, et al. SNAREpins: minimal machinery for membrane fusion. Cell. 1998;92(6):759–72. [PubMed: 9529252]
- 12.
- Carroll KS, Hanna J, Simon I, et al. Role of Rab9 GTPase in facilitating receptor recruitment by TIP47. Science. 2001;292(5520):1373–6. [PubMed: 11359012]
- 13.
- Jedd G, Mulholland J, Segev N. Two new Ypt GTPases are required for exit from the yeast trans-Golgi compartment. J Cell Biol. 1997;137(3):563–80. [PMC free article: PMC2139891] [PubMed: 9151665]
- 14.
- McLauchlan H, Newell J, Morrice N, et al. A novel role for Rab5-GDI in ligand sequestration into clathrin-coated pits. Curr Biol. 1998;8(1):34–45. [PubMed: 9427626]
- 15.
- Antonny B. Membrane deformation by protein coats. Curr Opin Cell Biol. 2006;18(4):386–394. [PubMed: 16782321]
- 16.
- Song BD, Schmid SL. A molecular motor or a regulator? Dynamin's in a class of its own. Biochemistry. 2003;42(6):1369–76. [PubMed: 12578348]
- 17.
- Kruchten AE, McNiven MA. Dynamin as a mover and pincher during cell migration and invasion. J Cell Sci. 2006;119(Pt 9):1683–90. [PubMed: 16636070]
- 18.
- Semerdjieva S, Shortt B, Maxwell E, et al. Coordinated regulation of AP2 uncoating from clathrin-coated vesicles by rab5 and hRME-6. J Cell Biol. 2008;183(3):499–511. [PMC free article: PMC2575790] [PubMed: 18981233]
- 19.
- Tanigawa G, Orci L, Amherdt M, et al. Hydrolysis of bound GTP by ARF protein triggers uncoating of Golgi-derived COP-coated vesicles. J Cell Biol. 1993;123(6 Pt 1):1365–71. [PMC free article: PMC2290881] [PubMed: 8253837]
- 20.
- Segev N. Ypt and Rab GTPases: insight into functions through novel interactions. Curr Opin Cell Biol. 2001;13(4):500–11. [PubMed: 11454458]
- 21.
- Barbero P, Bittova L, Pfeffer SR. Visualization of Rab9-mediated vesicle transport from endosomes to the trans-Golgi in living cells. J Cell Biol. 2002;156(3):511–8. [PMC free article: PMC2173336] [PubMed: 11827983]
- 22.
- Rink J, Ghigo E, Kalaidzidis Y, Zerial M. Rab conversion as a mechanism of progression from early to late endosomes. Cell. 2005;122(5):735–49. [PubMed: 16143105]
- 23.
- Gurkan C, Lapp H, Alory C, et al. Large-scale profiling of Rab GTPase trafficking networks: the membrome. Mol Biol Cell. 2005;16(8):3847–64. [PMC free article: PMC1182321] [PubMed: 15944222]
- 24.
- Prossnitz ER. Novel roles for arrestins in the post-endocytic trafficking of G protein-coupled receptors. Life Sci. 2004;75(8):893–9. [PubMed: 15193949]
- 25.
- Lin CH, Macgurn JA, Chu T, et al. Arrestin-related ubiquitin-ligase adaptors regulate endocytosis and protein turnover at the cell surface. Cell. 2008;135(4):714–25. [PubMed: 18976803]
- 26.
- Chen SH, Chen S, Tokarev AA, et al. Ypt31/32 GTPases and their novel F-box effector protein Rcy1 regulate protein recycling. Mol Biol Cell. 2005;16(1):178–92. [PMC free article: PMC539162] [PubMed: 15537705]
- 27.
- Jones S, Jedd G, Kahn RA, et al. Genetic interactions in yeast between Ypt GTPases and Arf guanine nucleotide exchangers. Genetics. 1999;152(4):1543–56. [PMC free article: PMC1460709] [PubMed: 10430582]
- 28.
- Garcia-Mata R, Sztul E. The membrane-tethering protein p115 interacts with GBF1, an ARF guanine-nucleotide-exchange factor. EMBO Rep. 2003;4(3):320–5. [PMC free article: PMC1315890] [PubMed: 12634853]
- 29.
- Lipatova Z, Tokarev AA, Jin Y, et al. Direct interaction between a myosin V motor and the Rab GTPases Ypt31/32 is required for polarized secretion. Mol Biol Cell. 2008;19(10):4177–87. [PMC free article: PMC2555963] [PubMed: 18653471]
- 30.
- Ortiz D, Medkova M, Walch-Solimena C, Novick P. Ypt32 recruits the Sec4p guanine nucleotide exchange factor, Sec2p, to secretory vesicles; evidence for a Rab cascade in yeast. J Cell Biol. 2002;157(6):1005–15. [PMC free article: PMC2174052] [PubMed: 12045183]
- 31.
- Rojas R, van Vlijmen T, Mardones GA, et al. Regulation of retromer recruitment to endosomes by sequential action of Rab5 and Rab7. J Cell Biol. 2008;183(3):513–26. [PMC free article: PMC2575791] [PubMed: 18981234]
- 32.
- Hayes GL, Brown FC, Haas AK, et al. Multiple Rab GTPase Binding Sites in GCC185 Suggest a Model for Vesicle Tethering at the Trans Golgi. Mol Biol Cell. 2009;20(1):209–17. [PMC free article: PMC2613123] [PubMed: 18946081]
- 33.
- Sacher M, Kim YG, Lavie A, et al. The TRAPP Complex: insights into its architecture and function. Traffic. 2008;9(12):2032–42. [PMC free article: PMC3417770] [PubMed: 18801063]
- 34.
- Morozova N, Liang Y, Tokarev AA, et al. TRAPPII subunits are required for the specificity switch of a Ypt-Rab GEF. Nat Cell Biol. 2006;8(11):1263–9. [PubMed: 17041589]
- 35.
- Park HO, Bi E. Central roles of small GTPases in the development of cell polarity in yeast and beyond. Microbiol Mol Biol Rev. 2007;71(1):48–96. [PMC free article: PMC1847380] [PubMed: 17347519]
- 36.
- Bryant DM, Mostov KE. From cells to organs: building polarized tissue. Nat Rev Mol Cell Biol. 2008;9(11):887–901. [PMC free article: PMC2921794] [PubMed: 18946477]
- 37.
- Barnes AP, Solecki D, Polleux F. New insights into the molecular mechanisms specifying neuronal polarity in vivo. Curr Opin Neurobiol. 2008;18(1):44–52. [PMC free article: PMC2488405] [PubMed: 18514505]
- 38.
- Coumailleau F, Gonzalez-Gaitan M. From endocytosis to tumors through asymmetric cell division of stem cells. Curr Opin Cell Biol. 2008;20(4):462–9. [PubMed: 18511252]
- 39.
- Li R, Gundersen GG. Beyond polymer polarity: how the cytoskeleton builds a polarized cell. Nat Rev Mol Cell Biol. 2008;9(11):860–73. [PubMed: 18946475]
- 40.
- Mellman I, Nelson WJ. Coordinated protein sorting, targeting and distribution in polarized cells. Nat Rev Mol Cell Biol. 2008;9(11):833–45. [PMC free article: PMC3369829] [PubMed: 18946473]
- 41.
- Wu H, Rossi G, Brennwald P. The ghost in the machine: small GTPases as spatial regulators of exocytosis. Trends Cell Biol. 2008;18(9):397–404. [PMC free article: PMC6521695] [PubMed: 18706813]
- 42.
- Sadowski L, Pilecka I, Miaczynska M. Signaling from endosomes: Location makes a difference. Exp Cell Res. 2009;315(9):1601–9. [PubMed: 18930045]
- 43.
- Palamidessi A, Frittoli E, Garre M, et al. Endocytic trafficking of Rac is required for the spatial restriction of signaling in cell migration. Cell. 2008;134(1):135–47. [PubMed: 18614017]
- 44.
- Entchev EV, Gonzalez-Gaitan MA. Morphogen gradient formation and vesicular trafficking. Traffic. 2002;3(2):98–109. [PubMed: 11929600]
- 45.
- Slagsvold T, Pattni K, Malerod L, Stenmark H. Endosomal and non-endosomal functions of ESCRT proteins. Trends Cell Biol. 2006;16(6):317–26. [PubMed: 16716591]
- 46.
- Dugani CB, Klip A. Glucose transporter 4: cycling, compartments and controversies. EMBO Rep. 2005;6(12):1137–42. [PMC free article: PMC1369215] [PubMed: 16319959]
- 47.
- Janka GE. Familial and acquired hemophagocytic lymphohistiocytosis. Eur J Pediatr. 2007;166(2):95–109. [PubMed: 17151879]
- 48.
- Tang Y, Olufemi L, Wang MT, Nie D. Role of Rho GTPases in breast cancer. Front Biosci. 2008;13:759–76. [PubMed: 17981586]
- 49.
- Wu G, Yussman MG, Barrett TJ, et al. Increased myocardial Rab GTPase expression: a consequence and cause of cardiomyopathy. Circ Res. 2001;89(12):1130–7. [PubMed: 11739277]
- 50.
- Chua CE, Tang BL. alpha-synuclein and Parkinson's disease: the first roadblock. J Cell Mol Med. 2006;10(4):837–46. [PubMed: 17125588]
- 51.
- Otomo A, Hadano S, Okada T, et al. ALS2, a novel guanine nucleotide exchange factor for the small GTPase Rab5, is implicated in endosomal dynamics. Hum Mol Genet. 2003;12(14):1671–87. [PubMed: 12837691]
- 52.
- Barral DC, Ramalho JS, Anders R, et al. Functional redundancy of Rab27 proteins and the pathogenesis of Griscelli syndrome. J Clin Invest. 2002;110(2):247–57. [PMC free article: PMC151050] [PubMed: 12122117]
- 53.
- Hattula K, Peranen J. FIP-2, a coiled-coil protein, links Huntingtin to Rab8 and modulates cellular morphogenesis. Curr Biol. 2000;10(24):1603–6. [PubMed: 11137014]
- 54.
- Cheng KW, Lahad JP, Kuo WL, et al. The RAB25 small GTPase determines aggressiveness of ovarian and breast cancers. Nat Med. 2004;10(11):1251–6. [PubMed: 15502842]
- 55.
- Martin-Serrano J. The role of ubiquitin in retroviral egress. Traffic. 2007;8(10):1297–303. [PubMed: 17645437]
- 56.
- Nagai H, Kagan JC, Zhu X, et al. A bacterial guanine nucleotide exchange factor activates ARF on Legionella phagosomes. Science. 2002;295(5555):679–82. [PubMed: 11809974]
- 57.
- Ingmundson A, Delprato A, Lambright DG, Roy CR. Legionella pneumophila proteins that regulate Rab1 membrane cycling. Nature. 2007;450(7168):365–9. [PubMed: 17952054]
- 58.
- Machner MP, Isberg RR. A bifunctional bacterial protein links GDI displacement to Rab1 activation. Science. 2007;318(5852):974–7. [PubMed: 17947549]
- 59.
- Sklan EH, Serrano RL, Einav S, et al. TBC1D20 is a Rab1 GTPase-activating protein that mediates hepatitis C virus replication. J Biol Chem. 2007;282(50):36354–61. [PubMed: 17901050]
- 60.
- Rzomp KA, Scholtes LD, Briggs BJ, et al. Rab GTPases are recruited to chlamydial inclusions in both a species-dependent and species-independent manner. Infect Immun. 2003;71(10):5855–70. [PMC free article: PMC201052] [PubMed: 14500507]
- 61.
- Madan R, Krishnamurthy G, Mukhopadhyay A. SopE-mediated recruitment of host Rab5 on phagosomes inhibits Salmonella transport to lysosomes. Methods Mol Biol. 2008;445:417–37. [PubMed: 18425466]
- 62.
- Panaro MA, Mitolo V, Cianciulli A, et al. The HIV-1 Rev binding family of proteins: the dog proteins as a study model. Endocr Metab Immune Disord Drug Targets. 2008;8(1):30–46. [PubMed: 18393921]
- 63.
- Blagoveshchenskaya AD, Thomas L, Feliciangeli SF, et al. HIV-1 Nef downregulates MHC-1 by a PACS-1- and P13K-regulated ARF6 endocytic pathway. Cell. 2002;111(6):853–66. [PubMed: 12526811]
- Introduction
- Regulation of Individual Transport Steps
- GTPases Regulating Individual Vesicular Transport Steps
- Posttranslational Modifications Regulating Cargo Sorting
- Transport Step Coordination
- Coordination of Individual Vesicular Transport Steps
- Integration of Individual Transport Steps into Whole Pathways
- Coordination of Intracellular Trafficking with Other Cellular Processes
- Intracellular Trafficking and Cell Polarity
- Intracellular Trafficking and Signal Transduction
- Intracellular Trafficking and Development
- Traffic Regulation and Human Disease
- Future Perspectives
- Acknowledgements
- References
- Regulation and Coordination of Intracellular Trafficking: An Overview - Madame C...Regulation and Coordination of Intracellular Trafficking: An Overview - Madame Curie Bioscience Database
- Pre-Ribosomal RNA Processing in Multicellular Organisms - Madame Curie Bioscienc...Pre-Ribosomal RNA Processing in Multicellular Organisms - Madame Curie Bioscience Database
- Regulation of T Cell Immunity by OX40 and OX40L - Madame Curie Bioscience Databa...Regulation of T Cell Immunity by OX40 and OX40L - Madame Curie Bioscience Database
- Overview Αβ Metabolism: From Alzheimer Research to Brain Aging Control - Madame ...Overview Αβ Metabolism: From Alzheimer Research to Brain Aging Control - Madame Curie Bioscience Database
- Metastatic Genitourinary Malignancies - Madame Curie Bioscience DatabaseMetastatic Genitourinary Malignancies - Madame Curie Bioscience Database
Your browsing activity is empty.
Activity recording is turned off.
See more...