NCBI Bookshelf. A service of the National Library of Medicine, National Institutes of Health.
Liedtke WB, Heller S, editors. TRP Ion Channel Function in Sensory Transduction and Cellular Signaling Cascades. Boca Raton (FL): CRC Press/Taylor & Francis; 2007.
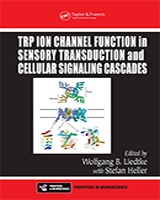
TRP Ion Channel Function in Sensory Transduction and Cellular Signaling Cascades.
Show detailsCa2+-activated nonselective cation channels were first described in the early 1980s [1,15,31], shortly after the patch-clamp recording technique was developed [5]. Patch-clamp recording, and in particular the excised-patch recording configuration, made it possible to rapidly and reproducibly change the intracellular milieu of cells and therefore to discover intracellular activators and modulators of ion channels. In this context, intracellular Ca2+ at micromolar concentrations was found to activate ion channels that were equally permeable to Na+ and K+ ions, and these ion channels were detected in a variety of cell types. The Ca2+-activated nonselective channels found in cultured heart cells were proposed to play a role in modulating the duration of the action potential, whereas those in pancreatic acinar cells were proposed to play a role in exocrine secretion [1,15]. However, without specific pharmacological or genetic tools, a clear attribution of function was difficult. In 2002 this situation changed with the cloning and functional expression of the first Ca2+-activated non-selective cation channel, TRPM4 [9]. Following this discovery, a second Ca2+-activated cation channel, TRPM5, was characterized [6,12,22,24,32]. Our understanding of the biological significance of these channels, their functional regulation, and their structural properties has since proceeded at a rapid pace.
CLONING, DISTRIBUTION, AND STRUCTURE OF TRPM4 AND TRPM5
TRPM4 and TRPM5 are members of the TRPM subfamily of transient receptor potential ion channels. The first member of the TRPM subfamily of ion channels, melastatin (TRPM1), was identified as a gene that was downregulated in mouse melanoma cell lines [2]. Based on similarity to TRPM1, a partial sequence of TRPM4 was detected in the database of human-expressed sequence tags and used to identify full-length cDNAs with two possible splice variants encoding proteins of 1,040 (TRPM4a) [30] and 1,214 (TRPM4b) [9] amino acids. Subsequently, only TRPM4b has been shown to be functional and therefore the term TRPM4 will be used to refer to this splice variant. TRPM4’s message is expressed widely and is at its highest levels in the heart, prostate, colon [30], placenta, and pancreas [9]. No localization of the mRNA or protein has yet been reported within these tissues.
TRPM5 was initially identified by homology to TRPM1 [4,23] and was later found by two independent groups to be a key component of mammalian taste cells [22,32]. Presently only a single splice variant has been reported. In contrast to TRPM4, TRPM5 mRNA is expressed in a highly restricted manner, and among major tissues of the body it is found only at high levels in the tongue, small intestine, and stomach [22]. Lower levels of TRPM5 may be expressed in other tissues [23].
TRPM4 and TRPM5, which are structurally more related to each other than to other TRP channels, show ~40 percent amino acid identity. Based on hydropathy analysis and homology to voltage-activated ion channels, both have been proposed to contain six transmembrane-spanning regions and to assemble as tetramers. The cytoplasmic N- and C-termini of TRPM4 and TRPM5 contain a number of potential protein–protein binding sites: the C-termini of both channels contain predicted coiled-coil domains and N- and C-termini of TRPM4 contain calmodulin-binding sites [19]. The pore of TRPM4 appears to be located between the fifth and sixth transmembrane domains, an assignment that is supported by the observation that mutations within this region alter ion selectivity [17]. Moreover, a histidine residue in the C-terminal portion of this region mediates an external proton block of TRPM5, supporting the contention that this region forms the outer pore vestibule [13].
ION PERMEABILITY, UNITARY PROPERTIES, AND SPECIFIC BLOCKERS OF TRPM4 AND TRPM5
Both TRPM4 and TRPM5 are robustly activated by intracellular Ca2+, making it possible to study their function properties in detail with patch-clamp recording [6,9,12,16,24]. These studies have revealed a number of similarities and differences between the two channels that will be helpful in classifying native channels and in understanding structure-function relationships. Both channels have a unitary conductance of ~25 pS [6,9,12,24], but whereas the openings of TRPM4 channels are long-lived (several hundred milliseconds; e.g., see reference 33), openings of TRPM5 channels are transient (e.g., see reference 12). Both channels are permeable to monovalent cations and impermeable to divalent cations [6,9,12,24].
At present only a few “specific” blockers of TRPM4 and TRPM5 channels have been identified. TRPM4 channels are blocked by micromolar concentrations of intracellular ATP and other adenine nucleotides [20], whereas TRPM5 channels are insensitive to these small molecules. TRPM5 channels are blocked by external pH of 6.0 or lower [13], whereas TRPM4 channels are insensitive to acid pH.
Based on these characteristics, one can assign the likely genetic basis for native channel activity; for example, based on the above criteria, the Ca2+-activated channels expressed in pheromone-sensing cells of the vomeronasal organs, which show long openings and are blocked by adenine nucleotides, are likely encoded by TRPM4 [10].
SENSITIVITY OF TRPM4 AND TRPM5 TO INTRACELLULAR CA2+
One of the most important ways in which TRPM4 and TRPM5 channels differ is in sensitivity to intracellular Ca2+. In excised inside-out patches immediately upon patch excision, the EC50 for activation by Ca2+ of mTRPM4 is 100–200 μM [27,33], and the EC50 for activation by Ca2+ of mTRPM5 is 20–30 μM [12,27] (Figure 15.1). Sensitivity to Ca2+ decreases for both channels by ~four-fold following patch excision and exposure to Ca2+ ; the EC50 for activation by Ca2+ after desensitization is ~500 μM and ~80 μM for TRPM4 and TRPM5, respectively [12,33]. Somewhat mysteriously, TRPM4 and TRPM5 currents in whole-cell recording mode are ~10–50 times more sensitive to Ca2+, and both can be maximally activated by concentrations of Ca2+ as low as 1 μM (e.g., see reference 27). It is not known why there is such a large discrepancy in Ca2+ sensitivity between excised-patch and whole-cell recording modes. One possibility is that a factor is lost upon patch excision that controls sensitivity (see below).
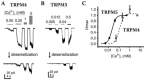
FIGURE 15.1
Ca2+-dependent activation of TRPM4 and TRPM5 currents. (A,B) Responses of TRPM4 and TRPM5 currents to increasing concentrations of Ca2+. The lower panels show currents recorded from the same patches after desensitization. (C) Dose-response relations of (more...)
ACTIVATION OF TRPM4 AND TRPM5 BY VOLTAGE
In the presence of an activating concentration of intracellular Ca2+, depolarization strongly increases the opening of TRPM4 and TRPM5 channels [6,12,16,26]. This is apparent in the strong outward rectification of TRPM4 and TRPM5 currents in response to voltage ramps, despite the fact that the underlying channels display a linear I–V relation [6,12,16,24,26]. In response to depolarizing voltage steps, both TRPM4 and TRPM5 currents show time-dependent relaxation, reflecting the opening of channels (Figure 15.2B). Voltage-dependent activation has also been reported for TRPM8 and TRPM4 and thus may be a common feature of TRPM channels [6,16,21,25]. It has been hypothesized that the weak voltage dependence of these channels allows their gating to be easily modulated [21], a hypothesis that is supported by work on cold regulation of TRPM8, TRPV1, and TRPM5 [26,29], decavanadate modulation of TRPM4 [18], and PI(4,5)P2 regulation of TRPM4 and TRPM8 [25,33] (see below). The structural mechanism of voltage sensing of these TRP channels is not presently understood, but the presence of several positively charged residues in the fourth transmembrane of these channels suggests that this may be the voltage sensor [7,21].
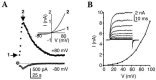
FIGURE 15.2
TRPM5 currents are Ca2+ and voltage dependent. (A) In whole-cell recording mode, 40 μM Ca2+ in the pipette elicited a large rectifying current in a HEK293 cell expressing TRPM5. Recording began shortly after break in to the whole-cell mode. Inset (more...)
REGULATION OF TRPM4 AND TRPM5 BY PIP2
A consistent observation is that TRPM4 and TRPM5 currents run down following activation, regardless of whether the currents are measured in whole-cell, perforated-patch, or excised-patch recording modes (Figure 15.2A and Figure 15.3). In excised patches, this rundown is associated with a decrease in the Ca2+ sensitivity of the channels, and thus can be formally considered desensitization [12,33]. What is the basis for desensitization? Recent evidence indicates that it is at least in part due to hydrolysis of membrane phophoinosides, most likely by Ca2+-activated membrane-associated PLCs [28]. The evidence for this conclusion includes the demonstration that (1) both TRPM5 and TRPM4 currents can be rescued from desensitization by PI(4,5)P2 [12,33] (Figure 15.3C); (2) TRPM4 currents can be rescued from desensitization by intracellular MgATP at concentrations that activate lipid kinases and are expected to restore PI(4,5)P2 levels, and this effect can be blocked by lipid kinase inhibitors [19,33] (Figure 15.3A); (3) desensitization of TRPM4 is promoted by depletion of PI(4,5)P2 with polyamines (Figure 15.3B).
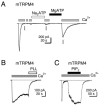
FIGURE 15.3
ATP and PI(4,5)P2 restore TRPM4 currents from desensitization. Inward currents evoked in response to 100 μM cytoplasmic Ca2+ were recorded from inside-out patches from TRPM4-expressing ChoK1 cells (Vm = −80 mV). (A) Following desensitization, (more...)
At present the mechanism and structural determinants for PI(4,5)P2 regulation of TRPM4 and TRPM5 is not known. Detailed study shows that PI(4,5)P2 changes the voltage-dependent gating of TRPM4 channels, promoting channel activation at negative membrane potentials by stabilizing the channels in the “voltage-activated” regulation state [33]. This mechanism is similar to one proposed to explain PI(4,5)P2 regulation of the structurally related, cold-activated channel TRPM8 [25]. Sensitivity to PI(4,5)P2 is conferred by positively charged residues in the TRP domain of TRPM8, a loosely conserved sequence of 25 amino acids located adjacent to the sixth transmembrane domain [25]. It is not known whether this region also mediates interaction of TRPM4 with PI(4,5)P2.
FUNCTION OF TRPM4 AND TRPM5
Insight into the function of TRPM5 came with the discovery that its expression is largely restricted to taste cells and the GI tract [22,32]. Taste consists of five distinct modalities of which three—bitter, sweet, and umami—are mediated by G-protein-coupled receptors. These receptors activate a signaling cascade of which several downstream targets have been identified, including Gα13, gustducin, and PLCβ2 [11,14]. TRPM5 is coexpressed with all three types of G-protein-coupled receptors as well as with their downstream signaling components [22,32], suggesting that it acts in the pathway for bitter, sweet, and umami taste transduction. In support of this idea, knockout of TRPM5 or PLCβ2 severely impairs the ability of mice to detect bitter, sweet, and umami tastes [32]. Based on the present genetic and physiological data, a preliminary model for transduction of bitter, sweet, and amino acid tastes can be proposed. In this model, receptor activation of PLCβ2 generates IP3, which releases Ca2+ from intracellular stores, and store-released Ca2+ opens TRPM5 channels. Opening of TRPM5 channels leads to a depolarization of the taste cell and transmitter release. Adaptation of taste responses may be partly mediated by the hydrolysis of PI(4,5)P2, which is a cofactor for activation of TRPM5 [12] (Figure 15.4, left).
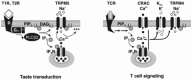
FIGURE 15.4
Models for physiological activation of TRPM5 and TRPM4. (Left) A model for taste transduction. Binding of taste stimuli to G-protein-coupled taste receptors (T1R and T2R) leads to dissociation of the heterotrimeric G protein. βγ subunits (more...)
TRPM4’s function has been more difficult to discern and a knockout of TRPM4, which would expedite this task, has not yet been reported. Moreover, TRPM4 mRNA is widely distributed, suggesting that it may play a less specific functional role than TRPM5. Despite these difficulties, there is strong evidence that TRPM4 plays a role in cytokine secretion by T lymphocytes and myogenic constriction of cerebral arteries [3,8]. T lymphocytes respond to stimulation with phyohemagglutinin (PHA) with Ca2+ oscillations that release the cytokine IL-2. Dominant negative suppression of TRPM4 and RNA knockdown of TRPM4 prolongs the Ca2+ response, leading to an increase in IL-2 production [8]. Thus TRPM4 appears to play a role in dampening the response of T cells to PHA. Based on these results, a model has been proposed in which activation of T-cell receptors induces a PLC-signing pathway leading to the depletion of Ca2+ from intracellular stores and the consequent entry of Ca2+ through CRAC channels. Ca2+ entry triggers activation of Ca2+-activated K+ channels, the opening of which promotes entry of Ca2+ by increasing the driving force across the plasma membrane. Activation of TRPM4 by Ca2+ entering through CRAC channels causes a depolarization of the membrane potential, which opposes further Ca2+ entry and promotes the opening of V-gated K+ channels. The interplay of these four conductances generates the Ca2+ oscillations observed in response to stimulation of T-cell receptors [8] (Figure 15.4, right).
Two models for the functional roles of Ca2+-activated TRPs have been proposed [8,12]. One posits that TRPM5 is activated by Ca2+ released from intracellular stores and that TRPM5 generates the primary electrical response to tastants. The second posits that TRPM4 is activated by Ca2+ entry through CRAC channels and that the primary function of TRPM4 is to dampen this signaling pathway. In this context, it may be significant that TRPM4 and TRPM5 differ widely in Ca2+ sensitivity and thus may respond to different Ca2+ signals in cells. For example, the higher sensitivity of TRPM5 to Ca2+ may allow it to respond to global changes in Ca2+ concentration following release of Ca2+ from intracellular stores, whereas TRPM4 might be insensitive to such signals and respond only to entry of Ca2+ through closely opposed membrane Ca2+ channels. A challenge will be to determine the sources of Ca2+ that activate these two channels under physiological conditions and to identify the specific attributes of each channel that allow it to respond selectively to the appropriate stimulus.
REFERENCES
- 1.
- Colquhoun D, Neher E, Reuter H, Stevens CF. Inward current channels activated by intracellular Ca in cultured cardiac cells. Nature. 1981;294:752–754. [PubMed: 6275271]
- 2.
- Duncan LM, Deeds J, Hunter J, Shao J, Holmgren LM, Woolf EA, Tepper RI, Shyjan AW. Down-regulation of the novel gene melastatin correlates with potential for melanoma metastasis. Cancer Res. 1998;58:1515–1520. [PubMed: 9537257]
- 3.
- Earley S, Waldron BJ, Brayden JE. Critical role for transient receptor potential channel TRPM4 in myogenic constriction of cerebral arteries. Circ Res. 2004;95:922–929. [PubMed: 15472118]
- 4.
- Enklaar T, Esswein M, Oswald M, Hilbert K, Winterpacht A, Higgins M, Zabel B, Prawitt D. Mtr1, a novel biallelically expressed gene in the center of the mouse distal chromosome 7 imprinting cluster, is a member of the Trp gene family. Genomics. 2000;67:179–187. [PubMed: 10903843]
- 5.
- Hamill OP, Marty A, Neher E, Sakmann B, Sigworth FJ. Improved patch-clamp techniques for high-resolution current recording from cells and cell-free membrane patches. Pflügers Arch. 1981;391:85–100. [PubMed: 6270629]
- 6.
- Hofmann T, Chubanov V, Gudermann T, Montell C. TRPM5 is a voltage-modulated and Ca(2+)-activated monovalent selective cation channel. Curr Biol. 2003;13:1153–1158. [PubMed: 12842017]
- 7.
- Jiang Y, Ruta V, Chen J, Lee A, MacKinnon R. The principle of gating charge movement in a voltage-dependent K+ channel. Nature. 2003;423:42–48. [PubMed: 12721619]
- 8.
- Launay P, Cheng H, Srivatsan S, Penner R, Fleig A, Kinet JP. TRPM4 regulates calcium oscillations after T cell activation. Science. 2004;306:1374–1377. [PubMed: 15550671]
- 9.
- Launay P, Fleig A, Perraud AL, Scharenberg AM, Penner R, Kinet JP. TRPM4 is a Ca2+-activated nonselective cation channel mediating cell membrane depolarization. Cell. 2002;109:397–407. [PubMed: 12015988]
- 10.
- Liman ER. Regulation by voltage and adenine nucleotides of a Ca2+-activated cation channel from hamster vomeronasal sensory neurons. J Physiol. 2003;548:777–787. [PMC free article: PMC2342889] [PubMed: 12640014]
- 11.
- Lindemann B. Receptors and transduction in taste. Nature. 2001;413:219–225. [PubMed: 11557991]
- 12.
- Liu D, Liman ER. Intracellular Ca2+ and the phospholipid PIP2 regulate the taste transduction ion channel TRPM5. Proc Natl Acad Sci USA. 2003;100:15160–15165. [PMC free article: PMC299934] [PubMed: 14657398]
- 13.
- Liu D, Zhang Z, Liman ER. Extracellular acid block and acid-enhanced inactivation of the Ca2+-activated cation channel TRPM5 involve residues in the S3–4 and S5–6 extracellular domains. J Biol Chem. 2005;280:20691–20699. [PubMed: 15731110]
- 14.
- Margolskee RF. Molecular mechanisms of bitter and sweet taste transduction. J Biol Chem. 2002;277:1–4. [PubMed: 11696554]
- 15.
- Maruyama Y, Petersen OH. Single-channel currents in isolated patches of plasma membrane from basal surface of pancreatic acini. Nature. 1982;299:159–161. [PubMed: 6287285]
- 16.
- Nilius B, Prenen J, Droogmans G, Voets T, Vennekens R, Freichel M, Wissenbach U, Flockerzi V. Voltage dependence of the Ca2+-activated cation channel TRPM4. J Biol Chem. 2003;278:30813–30820. [PubMed: 12799367]
- 17.
- Nilius B, Prenen J, Janssens A, Owsianik G, Wang C, Zhu MX, Voets T. The selectivity filter of the cation channel TRPM4. J Biol Chem. 2005;280:22899–22906. [PubMed: 15845551]
- 18.
- Nilius B, Prenen J, Janssens A, Voets T, Droogmans G. Decavanadate modulates gating of TRPM4 cation channels. J Physiol. 2004;560:753–765. [PMC free article: PMC1665285] [PubMed: 15331675]
- 19.
- Nilius B, Prenen J, Tang J, Wang C, Owsianik G, Janssens A, Voets T, Zhu MX. Regulation of the Ca2+ sensitivity of the nonselective cation channel TRPM4. J Biol Chem. 2005;280:6423–6433. [PubMed: 15590641]
- 20.
- Nilius B, Prenen J, Voets T, Droogmans G. Intracellular nucleotides and polyamines inhibit the Ca2+-activated cation channel TRPM4b. Pflügers Arch. 2004;448:70–75. [PubMed: 14758478]
- 21.
- Nilius B, Talavera K, Owsianik G, Prenen J, Droogmans G, Voets T. Gating of TRP channels: a voltage connection. J Physiol. 2005 [PMC free article: PMC1474154] [PubMed: 15878939]
- 22.
- Perez CA, Huang L, Rong M, Kozak JA, Preuss AK, Zhang H, Max M, Margolskee RF. A transient receptor potential channel expressed in taste receptor cells. Nat Neurosci. 2002;5:1169–1176. [PubMed: 12368808]
- 23.
- Prawitt D, Enklaar T, Klemm G, Gartner B, Spangenberg C, Winterpacht A, Higgins M, Pelletier J, Zabel B. Identification and characterization of MTR1, a novel gene with homology to melastatin (MLSN1) and the trp gene family located in the BWS-WT2 critical region on chromosome 11p15.5 and showing allele-specific expression. Hum Mol Genet. 2000;9:203–216. [PubMed: 10607831]
- 24.
- Prawitt D, Monteilh-Zoller MK, Brixel L, Spangenberg C, Zabel B, Fleig A, Penner R. TRPM5 is a transient Ca2+-activated cation channel responding to rapid changes in [Ca2+]i. Proc Natl Acad Sci USA. 2003;100:15166–15171. [PMC free article: PMC299937] [PubMed: 14634208]
- 25.
- Rohacs T, Lopes CM, Michailidis I, Logothetis DE. PI(4,5)P(2) regulates the activation and desensitization of TRPM8 channels through the TRP domain. Nat Neurosci. 2005;8:626–634. [PubMed: 15852009]
- 26.
- Talavera K, Yasumatsu K, Voets T, Droogmans G, Shigemura N, Ninomiya Y, Margolskee RF, Nilius B. Heat activation of TRPM5 underlies thermal sensitivity of sweet taste. Nature. 2005;438:1022–1025. [PubMed: 16355226]
- 27.
- Ullrich ND, Voets T, Prenen J, Vennekens R, Talavera K, Droogmans G, Nilius B. Comparison of functional properties of the Ca2+-activated cation channels TRPM4 and TRPM5 from mice. Cell Calcium. 2005;37:267–278. [PubMed: 15670874]
- 28.
- Varnai P, Balla T. Visualization of phosphoinositides that bind pleckstrin homology domains: calcium- and agonist-induced dynamic changes and relationship to myo-[3H]inositol-labeled phosphoinositide pools. J Cell Biol. 1998;143:501–510. [PMC free article: PMC2132833] [PubMed: 9786958]
- 29.
- Voets T, Droogmans G, Wissenbach U, Janssens A, Flockerzi V, Nilius B. The principle of temperature-dependent gating in cold- and heat-sensitive TRP channels. Nature. 2004;430:748–754. [PubMed: 15306801]
- 30.
- Xu XZ, Moebius F, Gill DL, Montell C. Regulation of melastatin, a TRP-related protein, through interaction with a cytoplasmic isoform. Proc Natl Acad Sci USA. 2001;98:10692–10697. [PMC free article: PMC58528] [PubMed: 11535825]
- 31.
- Yellen G. Single Ca2+-activated nonselective cation channels in neuroblastoma. Nature. 1982;296:357–359. [PubMed: 6278324]
- 32.
- Zhang Y, Hoon MA, Chandrashekar J, Mueller KL, Cook B, Wu D, Zuker CS, Ryba NJ. Coding of sweet, bitter, and umami tastes: different receptor cells sharing similar signaling pathways. Cell. 2003;112:293–301. [PubMed: 12581520]
- 33.
- Zhang Z, Okawa H, Wang Y, Liman ER. Phosphatidylinositol 4,5-bisphosphate rescues TRPM4 channels from desensitization. J Biol Chem. 2005;280:39185–39192. [PubMed: 16186107]
- 34.
- Nilius B, Mahieu F, Prenen J, Janssens A, Owsianik G, Vennekens R, Voets T. The Ca2+-activated cation channel TRPM4 is regulated by phosphatidylinositol 4,5-biphosphate. Embo J. 2006;25:467–478. [PMC free article: PMC1383543] [PubMed: 16424899]
After this chapter was submitted, Nilius et al. [34] showed a similar regulation of TRPM4 by PIP2 as we described.
- Review Functional Significance of Transient Receptor Potential Channels in Vascular Function.[TRP Ion Channel Function in Se...]Review Functional Significance of Transient Receptor Potential Channels in Vascular Function.Earley S, Reading S, Brayden JE. TRP Ion Channel Function in Sensory Transduction and Cellular Signaling Cascades. 2007
- Comparison of functional properties of the Ca2+-activated cation channels TRPM4 and TRPM5 from mice.[Cell Calcium. 2005]Comparison of functional properties of the Ca2+-activated cation channels TRPM4 and TRPM5 from mice.Ullrich ND, Voets T, Prenen J, Vennekens R, Talavera K, Droogmans G, Nilius B. Cell Calcium. 2005 Mar; 37(3):267-78.
- TRPM4 and TRPM5 Channels Share Crucial Amino Acid Residues for Ca(2+) Sensitivity but Not Significance of PI(4,5)P(2).[Int J Mol Sci. 2019]TRPM4 and TRPM5 Channels Share Crucial Amino Acid Residues for Ca(2+) Sensitivity but Not Significance of PI(4,5)P(2).Yamaguchi S, Tanimoto A, Iwasa S, Otsuguro K. Int J Mol Sci. 2019 Apr 24; 20(8). Epub 2019 Apr 24.
- Biochemical, single-channel, whole-cell patch clamp, and pharmacological analyses of endogenous TRPM4 channels in HEK293 cells.[Neurosci Lett. 2013]Biochemical, single-channel, whole-cell patch clamp, and pharmacological analyses of endogenous TRPM4 channels in HEK293 cells.Amarouch MY, Syam N, Abriel H. Neurosci Lett. 2013 Apr 29; 541:105-10. Epub 2013 Feb 18.
- Review The non-selective monovalent cationic channels TRPM4 and TRPM5.[Adv Exp Med Biol. 2011]Review The non-selective monovalent cationic channels TRPM4 and TRPM5.Guinamard R, Sallé L, Simard C. Adv Exp Med Biol. 2011; 704:147-71.
- The Ca2+-Activated TRP Channels: TRPM4 and TRPM5 - TRP Ion Channel Function in S...The Ca2+-Activated TRP Channels: TRPM4 and TRPM5 - TRP Ion Channel Function in Sensory Transduction and Cellular Signaling Cascades
Your browsing activity is empty.
Activity recording is turned off.
See more...