NCBI Bookshelf. A service of the National Library of Medicine, National Institutes of Health.
Liedtke WB, Heller S, editors. TRP Ion Channel Function in Sensory Transduction and Cellular Signaling Cascades. Boca Raton (FL): CRC Press/Taylor & Francis; 2007.
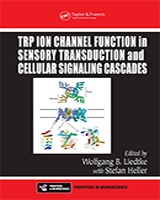
TRP Ion Channel Function in Sensory Transduction and Cellular Signaling Cascades.
Show detailsPain is a major health problem. Despite the importance of adequate pain modulation in relieving patients’ suffering, the available pharmacological agents for treating pain are limited. Existing pain-treating drugs that predominantly target the cyclooxygenase and opioid pathways are often inadequate in providing relief from pain and can have undesirable side effects. A first step in improving the armamentarium of physicians in treating pain is identifying new molecular targets for analgesic drugs. In this chapter, I describe a system for using Drosophila as a platform for discovering genes required for the function of insect nociceptive sensory neurons [1].
Forward genetics using Drosophila has resulted in important mechanistic insights into a variety of nervous system processes for which suitable behavioral assays have been developed. While these contributions are too numerous to list, notable examples include identification of the period locus affecting circadian rhythms, which led to a detailed understanding of molecular clocks [2], and mutations at the shaker locus that led to the cloning of the first voltage-activated K+ channel [3].
A DROSOPHILA MODEL FOR NOCICEPTION
For studies of nociception, my colleagues and I developed a paradigm based on a behavioral output that occurs in Drosophila larvae that have been stimulated with noxious heat or noxious mechanical stimuli. In response to such stimuli, the larvae produce a stereotyped escape response [1]. In contrast to normal locomotion, where the larva moves with caudal to rostral peristaltic waves of muscle contraction, after a noxious heat or mechanical stimulus larval locomotion changes to a characteristic “writhing” pattern. The defensive pattern of locomotion causes the larvae to roll across the substrate [1].
The threshold probe temperature for eliciting the Drosophila nociception behavior is 39°C. Similarly, through extracellular electrophysiological recordings we identified temperature-sensitive neurons of the peripheral nervous system that initiate firing at approximately 39°C [1]. Importantly, 39°C is similar to the threshold temperature of mammalian nociceptive nerve endings [4]. The similarity in temperature thresholds in mammalian nociceptive nerve endings and in insect nociception may indicate that nociception evolved in an ancient common ancestor of insects and mammals to protect a vital cellular process that begins to fail at or above approximately 39°C.
Our goal is to identify precisely the signaling mechanisms that evolved to acutely protect the organism from this critical failure. While I was a postdoc in the laboratory of Seymour Benzer, I took advantage of the stereotyped larval nociception behavioral response to conduct a genetic screen for mutations that produce a delayed behavioral response to noxious heat. In this screen, approximately 50 lines with altered nociception were identified.
MUTATIONS IN A TRPA ION CHANNEL INCREASE MECHANICAL AND THERMAL NOCICEPTION THRESHOLDS
The mutant we characterized in greatest detail was named painless [1]. We found that larvae mutant for painless have an increased threshold for eliciting the defensive behavioral response to either noxious heat or strong mechanical stimuli. Approximately 90 percent of wild-type larvae responded to a 46°C stimulus in 0.4 second [1]. In contrast, the average response latency is greater than three seconds in painless mutant larvae. The delayed behavioral response of painless mutant larvae is not due to a defective motor output because the mutant larvae show a rapid (0.4 second) response to a hotter (53°C) probe [1]. In wild-type larvae, the defensive rolling behavior also occurred in response to a 30mN punctate mechanical stimulus. In contrast, an equivalent level of response in painless mutant animals required a 100mN stimulus [1]. These results indicate that the painless mutation results in increased thresholds for both thermal and mechanical nociception.
In electrophysiological recordings, we found that bulk spiking activity increased three-fold in the noxious temperature range (>39°C) in wild-type larval nerves and that this increase of spiking did not occur in the painless mutant larvae. In wild type, at least two classes of neurons responded to increases in temperature. One class (low threshold) showed spontaneous room temperature firing and a marked increase in firing from 29–39°C. A second class (high threshold) was silent at room temperature and initiated strong firing above 39°C. Both classes of temperature response were absent in recordings from painless mutant nerves [1].
We rescued the painless mutant phenotype with 8.5Kb of genomic DNA containing a single predicted open reading frame (ORF). By in situ hybridization using cDNA probes encoding the ORF, we found that the transcript was expressed in the larval multidendritic neurons. In addition, an antibody raised against a peptide within the painless protein detected punctate structures associated with the dendritic arbor of multidendritic neurons. We isolated an enhancer trap allele in which the yeast transcription factor GAL4 was inserted at the painless locus. We used this GAL4 line to drive expression of green fluorescent protein (GFP) under control of GAL4-binding sites (UAS sites) and again observed expression in both the multidendritic neurons and chordotonal neurons. Interestingly, multidendritic neurons of Drosophila larvae have morphological similarity to naked nerve endings of mammalian nociceptors in that they are apparently not associated with a specialized receptor cell and have neurites that are in direct contact with epidermal cells that secrete cuticle (Figure 16.1) [1].
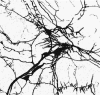
FIGURE 16.1
Type II (multidendritic) sensory neurons. While the function of these cells was previously unknown, the available evidence suggests that these cells, or a subset of them, function as nociceptors. Blocking the synaptic output of these cells blocks nociception (more...)
Several lines of evidence suggest that the multidendritic neurons, but not the chordotonal neurons, included the heat-sensing nociceptive neurons. First, blocking the synaptic output of the multidendritic neurons with tetanus toxin completely abrogates the behavioral response to noxious heat [1]. Second, multidendritic neurons show strong Ca++ responses to heating with only weak responses in chordotonal neurons [5]. Third, atonal mutants, which lack chordotonal neurons, showed a rapid response to noxious heat [1].
The painless gene encodes an ion channel of the transient receptor potential (TRP) family [1]. Isolation of mutations in a TRP ion channel in our screen is consistent with other findings that have implicated individual TRP channels in mechanotransduction [1,6–10] and in thermal sensory transduction [1,11–16]. Based on sequence homology, the TRP channel family has seven subfamilies: TRPA, TRPV, TRPP, TRPM, TRPC, TRPN, and TRPML [17]. The painless gene is a member of the TRPA subfamily.
DISTINCT CELLULAR AND MOLECULAR PATHWAYS FOR INNOCUOUS AND NOXIOUS TOUCH DETECTION IN DROSOPHILA
When we initiated our nociception screens, Kernan and colleagues had previously screened for chemically induced mutations that resulted in larval insensitivity to a light touch [18]. In this paradigm, the larvae are lightly touched on the head segment using an eyelash that elicits a maximum force of <10mN. In response, wild-type larvae pause forward movement or initiate reverse peristaltic waves of muscle contraction to move away from the stimulus. At the behavioral level, this response is completely distinct from the writhing nociception response that occurs with harsh touch of >30mN.
Kernan and others have cloned several light touch–insensitive mutants such as NompC, NompA, and unc [6,19,20]. Each of these genes has been expressed in ciliated Type I bipolar sensory neurons or in other cells of the Type I sensillum (Figure 16.2). Touch-insensitive mutants isolated in the Kernan screen do not affect the writhing response to strong mechanical stimuli (W.D. Tracey, unpublished).
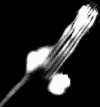
FIGURE 16.2
Type I sensory neurons. These bipolar sensory neurons function as part of a sensillum consisting of four cells. Type I sensory neurons are used in a variety of sensory processes including olfaction, gustation, light touch detection, and in hearing of (more...)
In contrast, the painless gene product is primarily absent from Type I sensory neurons but is strongly expressed in the multidendritic Type II sensory neurons. Consistent with this, painless mutants show a normal behavioral response to light touch but are defective in the strong touch response. This result genetically separates the light touch response from the harsh touch response. Because light touch response genes are absent from the Type II sensory neurons while painless is present in these cells, these data further implicate the Type II sensory neurons as nociceptors. Taken together, the available evidence suggests that Drosophila Type I mechanosensory neurons are required for light touch detection, while Type II sensory neurons are used in harsh touch detection.
THERMO TRP CHANNELS ARE TEMPERATURE SENSORS IN MAMMALS
In mammals, external temperature is sensed by the nerve endings of neurons whose cell bodies reside in sensory ganglia such as the trigeminal and dorsal root ganglia (DRG). Several TRP channels have been expressed in trigeminal and DRG neurons and have been shown to produce temperature-dependent currents in heterologous expression systems. Indeed, the first identified temperature-sensitive TRP channel (TRPV1) is activated by temperatures >42°C. TRPV1 is also activated by capsaicin, the spicy ingredient in chili peppers [12]. In addition, mice mutant for TRPV1 eat capsaicin with impunity. Combined, these observations indicate that the ‘‘hot’’ sensation produced by capsaicin and other vanilloid compounds is due to activation of thermal pain-sensing nerve endings through TRPV1 [12]. Other warmth-activated TRPs include TRPV2 (>52°C) [11], TRPV3 (>31°C) [21], and TRPV4 (>25°C) [22]. TRPM8 is activated by menthol and cooling (8–28°C) [14].
According to the properties of these channels in heterologous expression systems, the best candidate for encoding the temperature-mediated response of nociceptive sensory neurons in mice is TRPV1. This is because heat-activated nociceptors show increased firing at temperature thresholds when the nerve endings reach a temperature of approximately 39°C, and the activation threshold for TRPV1 is similar. Indeed, thermal hyperalgesia is significantly reduced in TRPV1 knockout mice, but thermal nociception is not completely abrogated by the TRPV1 mutation [23,24]. More recently, mice mutant for TRPV3 have also been shown to have altered thermal preference and increased nociception thresholds [25].
MULTIPLE TRPA CHANNELS ARE HEAT ACTIVATED IN FLIES
Drosophila TRPV channels have not been found to be heat activated [8,26]. In contrast, two of the three identified TRPA channels in flies are activated by heat [27–29]. Phylogenetic analysis suggests that more than one TRPA gene existed in a common ancestor of flies and mammals. The presence of a single TRPA gene in modern humans, rats, and mice suggests either a simplification of this gene family in the mammalian lineage or an expansion of the family in the insect lineage. Three TRPA genes in flies have been functionally analyzed and have apparently distinct functions. As mentioned above, painless is required for thermal nociception but not for thermotaxis [29,30]. The painless gene that we identified by forward genetics is not the orthologue of the mammalian TRPA1 channel. Another gene, dTRPA1, is more closely related to mammalian TRPA1 [29,30]. RNAi knockdown of dTRPA1 results in thermotaxis defects without affecting thermal nociception [29]. Mutants of a third Drosophila TRPA gene named pyrexia show reduced tolerance to thermal stress [28].
Although painless is not the Drosophila sequence orthologue of mammalian TRPA1, the expansion of the TRPA family found in flies may have allowed each Drosophila TRPA gene to retain a subfunction of an ancestral TRPA gene. Because painless is expressed in Drosophila nociceptors but dTRPA1 is not, painless can be considered the functional orthologue of mammalian TRPA1 when nociception pathways are under consideration. It is possible that pyrexia may also be involved in nociception because it is coexpressed in multidendritic neurons with painless, but we have not observed nociception defects in pyrexia mutants (W.D. Tracey, unpublished).
EVIDENCE FOR COMBINATORIAL ENCODING OF TEMPERATURE RESPONSE
The painless channel is required for Drosophila sensory neurons to be activated by increasing temperature [1]. However, paradoxically, painless is required in vivo for the heat-dependent activation of two distinct types of thermosensory neurons (Figure 16.3) [1]. The first type (low threshold) activates at approximately 29°C and inactivates at around 39°C (Figure 16.3). The second type (high threshold) activates at 39°C but is silent below this temperature. Several distinct hypotheses can be proposed to explain the firing properties of these two classes of thermosensory neurons. The first possibility is that there are multiple isoforms of painless that show distinct activation thresholds of 29°C and 39°C, respectively. Evidence from expressed sequence tags supports the possibility that there may be multiple painless isoforms. Our preliminary unpublished results suggest that at least one of the painless isoforms, when expressed heterologously, is directly heat activated and has an activation threshold of 29°C. In addition, we identified a second isoform of painless that is a candidate for encoding the putative 39°C threshold isoform. However, the pyrexia thermoTRP also has similar multiple isoforms, and thermal-activation thresholds among these isoforms is similar [28], so it may be unlikely that the two distinct isoforms of painless have dramatically different thermal-activation thresholds.
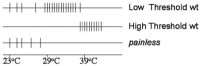
FIGURE 16.3
Schematic representation of Drosophila thermosensory neuron firing patterns. A low-threshold type has spontaneous room temperature activity and increased firing above 29°C and inactivation at 39°C. A high-threshold type activates at approximately (more...)
A second model that would explain the properties of these two types of thermosensory neurons is more likely (Figure 16.4). This model invokes other thermosensory channels. In this model, there is only a single thermosensitive painless channel, which is activated at temperatures >29°C, and this painless channel is expressed in both the low-threshold and high-threshold types of thermosensory neurons. The properties of the high-threshold cell could be explained if there was a coexpressed K+ current in that type of cell that would prevent firing below 39°C. An interesting candidate for such a channel could be a leak channel that showed K+ selectivity and inactivation at >39°C. A second K+-selective thermoTRP channel that opens at >39°C could explain the inactivation of the low-threshold thermosensory neuron at this temperature.
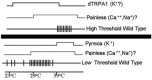
FIGURE 16.4
Hypothesis for combinatorial encoding of thermosensory neuron firing properties. We propose that painless is an “excitatory” thermoTRP and that pyrexia is an inhibitory thermoTRP. This model predicts that the low-threshold type of thermosensory (more...)
A variation of this model is that painless may form heteromultimers with these other channels and the heteromultimer might show a thermal-activation threshold distinct from that of the painless homomultimer.
Both dTRPA1 and pyrexia have been shown to function as thermoTRPs and are thus candidates as these hypothetical K+-selective thermoTRPs or as heteromeric partners for painless. dTRPA1 is activated at temperatures greater than 23°C, while pyrexia is activated at temperatures greater than 39°C. Indeed, pyrexia-PA and pyrexia-PB heteromeric channels are somewhat selective for K+ and have been proposed to inhibit neurons from firing under conditions of thermal stress [28]. The expression pattern, thermal-activation threshold, and ion selectivity of pyrexia are consistent with the possibility that pyrexia is a channel that is coexpressed with painless to inactivate the low-threshold type of thermosensory neuron at 39°C. This is because pyrexia is expressed in a subset of the multidendritic neurons that also express painless [28]. A logical extension of this model is that dTRPA1 could be the other putative K+-selective thermoTRP. However, currently available anti-dTRPA1 antibodies have not detected expression in multidendritic neurons. This may be due to levels of expression below the limits of detection or, alternatively, other K+-selective channels must be considered.
A novel and exciting prediction of the second model is that the firing properties of Drosophila thermosensitive neurons might be combinatorially determined by “excitatory” and “inhibitory” thermoTRPs. Based on the thermal thresholds of the known Drosophila thermoTRPs and on the painless mutant phenotypes, we hypothesize that painless is an excitatory TRPA channel and that pyrexia and possibly dTRPA are inhibitory thermoTRPs. A simple combinatorial code could explain the firing properties of the neurons. Namely, coexpression of painless and pyrexia might encode the low-threshold firing pattern and coexpression of dTRPA1 (or another thermo K+ channel), and painless might encode the high-threshold firing pattern (Figure 16.4). This is a theoretical prediction, and it is possible that the inhibitory K+-selective channels may not be TRP channels at all. For example, the seizure gene encodes a K+ channel that when mutant causes neuronal hyperexcitability in flies, specifically at high temperatures.
The simplicity of the Drosophila peripheral nervous system provides a significant advantage to understand neural substrates encoding thermosensory information. Most important, the precise number, identity, and location of individual peripheral neurons are invariant from animal to animal. This allows us to determine the precise expression patterns of painless, dTRPA1, and pyrexia to the resolution of individual identified neurons. Further, using the tools available in Drosophila, we will be able to determine the consequence of altering the activity of these identified neurons on thermal preference and nociception behaviors.
PUTATIVE FUNCTIONS FOR THE HIGH-THRESHOLD AND LOW-THRESHOLD TYPES OF THERMOSENSORY NEURONS
The two types of thermosensory neurons that we discovered in Drosophila suggest a mechanism for the brain to compute the direction of temperature change during a noxious thermal stimulus presentation (Figure 16.5). For example, central neurons receiving input from both the low-threshold type and the high-threshold type could compute that temperature is increasing in instances where firing of the low-threshold type temporally precedes the high-threshold type. The reverse pattern would indicate a temperature decrease. Although intuitively obvious, whether the brain of the fly or any other species actually uses this simple mechanism of comparison is not known. This hypothesis for computation of direction of temperature change makes interesting predictions concerning the effects of removing the low-threshold neuron. For example, an animal lacking a signal from this neuron may show a prolonged rolling response to a noxious heat probe. This would occur because the high-threshold neuron response should be sufficient to initiate the rolling behavior, but the brain may require input from the low-threshold neuron in order to “know” when the thermal danger has passed and to reinitiate normal locomotion. Absence of input from the low-threshold neuron would therefore be predicted to cause a form of hyperalgesia. That human central nervous system pain pathways involve comparison of input from distinct thermosensory neuronal types is suggested from the thermal grill pain illusion. In this illusion, a human hand placed on a grill of alternating cool (18°C) and warm (37°C) bars elicits a sensation of pain [31–33]. This suggests that innocuous thermoreception influences central pain circuits.
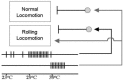
FIGURE 16.5
Hypothetical thermal nociception circuit. We hypothesize that the low-threshold type of thermosensory neuron (top trace) drives inhibition of a rolling locomotion pattern generator. The high-threshold type (bottom trace) should simultaneously activate (more...)
THE MAMMALIAN TRPA1 CHANNEL IS ACTIVATED BY IRRITANTS THAT ELICIT SENSATIONS OF BURNING PAIN
As we characterized the painless mutant, other groups of investigators simultaneously began to investigate the single homologue of the painless gene that has been identified in mammalian genomes (TRPA1) [34]. The available evidence suggests that the TRPA1 gene is in fact an excellent candidate to play an important role in pain signaling. First, TRPA1 was found to be expressed in a subset of TRPV1-expressing neurons, which are likely to be nociceptors [34]. Second, using heterologous expression systems, the TRPA1 gene was found to be activated by a variety of compounds known to elicit a burning sensation of pain such as wasabi (Japanese horseradish), mustard oils, cinnemaldehyde (the active compound of cinnamon and spicy hot candies), and, most recently, raw garlic. [35,36,37],46 Activation of TRPA1 in vitro by these compounds suggests that the psychophysical sensation produced by activating TRPA1-expressing neurons in vivo is that of burning pain. In addition, TRPA1 can be activated downstream of pain-modulating G-protein-coupled receptors such as bradykinin receptors [35,36].
mTRPA1 may function as a thermoTRP. The mouse channel can be activated by noxious cold (<16°C) in heterologous expression systems [38], but this has not been observed in every study of this channel [35,36]. Whether noxious cold activation of mTRPA1 is direct or due to an indirect effect of elevated intracellular Ca++ is not known [35,36]. In culture, noxious cold–responding neurons do not respond to mustard oils, which do activate TRPA1 in heterologous cell types [39]. The observation that compounds that elicit a burning hot sensation activate TRPA1 in heterologous cells may indicate that in vivo functions of TRPA1 are present in neurons that participate in noxious heat detection, or, alternatively, burning cold and burning heat may be psychophysically related cognitive processes [38].
TRP CHANNELS HAVE BEEN IMPLICATED IN MECHANOTRANSDUCTION
The TRP channels osm-9, NOMP-C, Nanchung, Inactive, TRPV1, zNOMP-C, and painless are each genetically required for behavioral or physiological responses to mechanical stimuli [1,6–9,26]. In C. elegans, osm-9 mutants are defective in nose touch avoidance and for other nociceptive functions such as avoidance of high osmotic strength [7]. In Drosophila, no mechanoreceptor potential-C mutants are defective in the response to touch and for the mechanoreceptor potential of neurons that innervate bristles of the fly [6,18]. Drosophila Nanchung and Inactive, and zebrafish NOMP-C, are each required for hearing [40–43]. Finally, painless mutant larvae have an increased threshold for avoiding harsh touch but a normal response to light touch [1].
Perhaps the longest-sought mechanotransducing ion channel is the channel responsible for depolarization of the hair cells of the inner ear in response to mechanical vibrations that are produced by sound. This channel is present in only several hundred copies per cell and thus has resisted biochemical purification. Recent evidence suggests that, in addition to nociceptive sensory neurons, TRPA1 is present in hair cells of the inner ear. Antibodies raised against TRPA1 detect structures at the tips of actin-rich stereovilli in several species and knockdown reduced the receptor potential [10]. Thus, TRPA1 is an excellent candidate for the hair cell mechanotransducer [10].
This emerging evidence suggests that TRPA1 may have distinct functions in distinct cell types. In nociceptors, TRPA1 may elicit burning sensations of pain, and in the ear it is a good candidate to be involved in hair cell mechanotransduction. The function of TRPA1 in nociceptors may be as a “painful pinch” detector as with painless. The exact role of TRPA1 in these cell types awaits the generation of mice mutant in this gene. Nociceptive neurons and hair cells are two seemingly very distinct cell types that express TRPA1. It is likely that cell-type specific cofactors modulate the function of this channel in these distinct cells.
Our Drosophila system is specifically designed to identify peripheral nervous system regulators of nociception. The Drosophila genome contains neither opioid receptors nor cyclooxygenase enzymes. While these pathways are clearly extremely important pathways in pain modulation, it is essential to identify novel pathways that could be modulated independently of them. Because Drosophila lacks these pathways, we have a priori knowledge that the genes we identify will not touch on the well-studied opioid and cyclooxygenase systems. The understanding of peripheral pathways for nociception and identification of new targets that can modulate peripheral pathways is an important area of health research for improved patient care. Although many compounds used in pain management act on central nervous system targets, it is well established that blocking peripheral input to the dorsal horn can prevent wind-up (a sensitized neural circuit in the dorsal horn) [44]. Once wind-up has occurred, pain management for the physician becomes more difficult [45]. Therefore, understanding primary nociception is important to prevent sensitized neural circuits that may occur as a consequence of tissue damage [45]. If the peripheral pathways can be modulated, this may prevent wind-up and the development of central pain syndromes [44,45]. Physicians working on the incredibly complex problem of human pain in many instances simply do not have the pharmacological tools that they need for adequate patient care. By focusing on the simplest model system possible, we hope to contribute to a mechanistic understanding that will assist in providing these tools.
REFERENCES
- 1.
- Tracey WD Jr, et al. Painless, a Drosophila gene essential for nociception. Cell. 2003;113(2):261–73. [PubMed: 12705873]
- 2.
- Konopka RJ, Benzer S. Clock mutants of Drosophila melanogaster. Proc Natl Acad Sci USA. 1971;68(9):2112–16. [PMC free article: PMC389363] [PubMed: 5002428]
- 3.
- Papazian DM, et al. Cloning of genomic and complementary DNA from Shaker, a putative potassium channel gene from Drosophila. Science. 1987;237(4816):749–53. [PubMed: 2441470]
- 4.
- Tillman DB, et al. Response of C fibre nociceptors in the anaesthetized monkey to heat stimuli: correlation with pain threshold in humans. J Physiol. 1995;485(Pt. 3):767–74. [PMC free article: PMC1158042] [PubMed: 7562615]
- 5.
- Liu L, et al. Identification and function of thermosensory neurons in Drosophila larvae. Nat Neurosci. 2003;6(3):267–73. [PubMed: 12563263]
- 6.
- Walker RG, Willingham AT, Zuker CS. A Drosophila mechanosensory transduction channel. Science. 2000;287(5461):2229–34. [PubMed: 10744543]
- 7.
- Colbert HA, Smith TL, Bargmann CI. OSM-9, a novel protein with structural similarity to channels, is required for olfaction, mechanosensation, and olfactory adaptation in Caenorhabditis elegans. J Neurosci. 1997;17(21):8259–69. [PMC free article: PMC6573730] [PubMed: 9334401]
- 8.
- Kim J, et al. A TRPV family ion channel required for hearing in Drosophila. Nature. 2003;424(6944):81–84. [PubMed: 12819662]
- 9.
- Sidi S, Friedrich RW, Nicolson T. NompC TRP channel required for vertebrate sensory hair cell mechanotransduction. Science. 2003;301(5629):96–99. [PubMed: 12805553]
- 10.
- Corey DP, et al. TRPA1 is a candidate for the mechanosensitive transduction channel of vertebrate hair cells. Nature. 2004;432(7018):723–30. [PubMed: 15483558]
- 11.
- Caterina MJ, et al. A capsaicin-receptor homologue with a high threshold for noxious heat. Nature. 1999;398(6726):436–41. [PubMed: 10201375]
- 12.
- Caterina MJ, et al. The capsaicin receptor: a heat-activated ion channel in the pain pathway. Nature. 1997;389(6653):816–24. [PubMed: 9349813]
- 13.
- Bassilana F, et al. The acid-sensitive ionic channel subunit ASIC and the mammalian degenerin MDEG form a heteromultimeric H+-gated Na+ channel with novel properties. J Biol Chem. 1997;272(46):28819–22. [PubMed: 9360943]
- 14.
- McKemy DD, Neuhausser WM, Julius D. Identification of a cold receptor reveals a general role for TRP channels in thermosensation. Nature. 2002;416(6876):52–58. [PubMed: 11882888]
- 15.
- Peier AM, et al. A TRP channel that senses cold stimuli and menthol. Cell. 2002;108(5):705–15. [PubMed: 11893340]
- 16.
- Jordt SE, McKemy DD, Julius D. Lessons from peppers and peppermint: the molecular logic of thermosensation. Curr Opin Neurobiol. 2003;13(4):487–92. [PubMed: 12965298]
- 17.
- Clapham DE. TRP channels as cellular sensors. Nature. 2003;426(6966):517–24. [PubMed: 14654832]
- 18.
- Kernan M, Cowan D, Zuker C. Genetic dissection of mechanosensory transduction: mechanoreception-defective mutations of Drosophila. Neuron. 1994;12(6):1195–206. [PubMed: 8011334]
- 19.
- Baker JD, Adhikarakunnathu S, Kernan MJ. Mechanosensory-defective, male-sterile unc mutants identify a novel basal body protein required for ciliogenesis in Drosophila. Development. 2004;131(14):3411–22. [PubMed: 15226257]
- 20.
- Chung YD, et al. nompA encodes a PNS-specific, ZP domain protein required to connect mechanosensory dendrites to sensory structures. Neuron. 2001;29(2):415–28. [PubMed: 11239432]
- 21.
- Smith GD, et al. TRPV3 is a temperature-sensitive vanilloid receptor-like protein. Nature. 2002;418(6894):186–90. [PubMed: 12077606]
- 22.
- Guler AD, et al. Heat-evoked activation of the ion channel, TRPV4. J Neurosci. 2002;22(15):6408–14. [PMC free article: PMC6758176] [PubMed: 12151520]
- 23.
- Caterina MJ, et al. Impaired nociception and pain sensation in mice lacking the capsaicin receptor. Science. 2000;288(5464):306–13. [PubMed: 10764638]
- 24.
- Davis JB, et al. Vanilloid receptor-1 is essential for inflammatory thermal hyperalgesia. Nature. 2000;405(6783):183–87. [PubMed: 10821274]
- 25.
- Moqrich A, et al. Impaired thermosensation in mice lacking TRPV3, a heat and camphor sensor in the skin. Science. 2005;307(5714):1468–72. [PubMed: 15746429]
- 26.
- Gong Z, et al. Two interdependent TRPV channel subunits, inactive and Nanchung, mediate hearing in Drosophila. J Neurosci. 2004;24(41):9059–66. [PMC free article: PMC6730075] [PubMed: 15483124]
- 27.
- Viswanath V, et al. Opposite thermosensor in fruitfly and mouse. Nature. 2003;423(6942):822–23. [PubMed: 12815418]
- 28.
- Lee Y, et al. Pyrexia is a new thermal transient receptor potential channel endowing tolerance to high temperatures in Drosophila melanogaster. Nat Genet. 2005;37(3):305–10. [PubMed: 15731759]
- 29.
- Rosenzweig M, et al. The Drosophila ortholog of vertebrate TRPA1 regulates thermotaxis. Genes Dev. 2005;19(4):419–24. [PMC free article: PMC548941] [PubMed: 15681611]
- 30.
- Tracey WD Jr, et al. Quantitative analysis of gene function in the Drosophila embryo. Genetics. 2000;154(1):273–84. [PMC free article: PMC1460918] [PubMed: 10628987]
- 31.
- Bouhassira D, et al. Investigation of the paradoxical painful sensation (‘‘illusion of pain’’) produced by a thermal grill. Pain. 2005;114(1–2):160–67. [PubMed: 15733641]
- 32.
- Craig AD, et al. Functional imaging of an illusion of pain. Nature. 1996;384(6606):258–60. [PubMed: 8918874]
- 33.
- Craig AD, Bushnell MC. The thermal grill illusion: unmasking the burn of cold pain. Science. 1994;265(5169):252–55. [PubMed: 8023144]
- 34.
- Story GM, et al. ANKTM1, a TRP-like channel expressed in nociceptive neurons, is activated by cold temperatures. Cell. 2003;112(6):819–29. [PubMed: 12654248]
- 35.
- Bandell M, et al. Noxious cold ion channel TRPA1 is activated by pungent compounds and bradykinin. Neuron. 2004;41(6):849–57. [PubMed: 15046718]
- 36.
- Jordt SE, et al. Mustard oils and cannabinoids excite sensory nerve fibres through the TRP channel ANKTM1. Nature. 2004;427(6971):260–65. [PubMed: 14712238]
- 37.
- Macpherson LJ, et al. The pungency of garlic: activation of TRPA1 and TRPV1 in response to allicin. Curr Biol. 2005;15(10):929–34. [PubMed: 15916949]
- 38.
- Darboux I, et al. A new member of the amiloride-sensitive sodium channel family in Drosophila melanogaster peripheral nervous system. Biochem Biophys Res Commun. 1998;246(1):210–16. [PubMed: 9600094]
- 39.
- Babes A, Zorzon D, Reid G. Two populations of cold-sensitive neurons in rat dorsal root ganglia and their modulation by nerve growth factor. Eur J Neurosci. 2004;20(9):2276–82. [PubMed: 15525269]
- 40.
- Mogil JS, et al. One or two genetic loci mediate high opiate analgesia in selectively bred mice. Pain. 1995;60(2):125–35. [PubMed: 7784097]
- 41.
- Marek P, Panocka I, Sadowski B. Selective breeding of mice for high and low swim analgesia: differential effect on discrete forms of footshock analgesia. Pain. 1987;29(3):393–98. [PubMed: 3614973]
- 42.
- Bier E, et al. Searching for pattern and mutation in the Drosophila genome with a P- lacZ vector. Genes Dev. 1989;3(9):1273–87. [PubMed: 2558049]
- 43.
- Pastor J, Soria B, Belmonte C. Properties of the nociceptive neurons of the leech segmental ganglion. J Neurophysiol. 1996;75(6):2268–79. [PubMed: 8793740]
- 44.
- Seltzer Z, et al. The role of injury discharge in the induction of neuropathic pain behavior in rats. Pain. 1991;46(3):327–36. [PubMed: 1758712]
- 45.
- Arendt-Nielsen L, Petersen-Felix S. Wind-up and neuroplasticity: is there a correlation to clinical pain? Eur J Anaesthesiol Suppl. 1995;10:1–7. [PubMed: 7641635]
- A DROSOPHILA MODEL FOR NOCICEPTION
- MUTATIONS IN A TRPA ION CHANNEL INCREASE MECHANICAL AND THERMAL NOCICEPTION THRESHOLDS
- DISTINCT CELLULAR AND MOLECULAR PATHWAYS FOR INNOCUOUS AND NOXIOUS TOUCH DETECTION IN DROSOPHILA
- THERMO TRP CHANNELS ARE TEMPERATURE SENSORS IN MAMMALS
- MULTIPLE TRPA CHANNELS ARE HEAT ACTIVATED IN FLIES
- EVIDENCE FOR COMBINATORIAL ENCODING OF TEMPERATURE RESPONSE
- PUTATIVE FUNCTIONS FOR THE HIGH-THRESHOLD AND LOW-THRESHOLD TYPES OF THERMOSENSORY NEURONS
- THE MAMMALIAN TRPA1 CHANNEL IS ACTIVATED BY IRRITANTS THAT ELICIT SENSATIONS OF BURNING PAIN
- TRP CHANNELS HAVE BEEN IMPLICATED IN MECHANOTRANSDUCTION
- REFERENCES
- Review Molecular mechanism and cellular distribution of insect circadian clocks.[Annu Rev Entomol. 2000]Review Molecular mechanism and cellular distribution of insect circadian clocks.Giebultowicz JM. Annu Rev Entomol. 2000; 45:769-93.
- Review Engineering Aspects of Olfaction.[Neuromorphic Olfaction. 2013]Review Engineering Aspects of Olfaction.Persaud KC. Neuromorphic Olfaction. 2013
- Opioids and the management of chronic severe pain in the elderly: consensus statement of an International Expert Panel with focus on the six clinically most often used World Health Organization Step III opioids (buprenorphine, fentanyl, hydromorphone, methadone, morphine, oxycodone).[Pain Pract. 2008]Opioids and the management of chronic severe pain in the elderly: consensus statement of an International Expert Panel with focus on the six clinically most often used World Health Organization Step III opioids (buprenorphine, fentanyl, hydromorphone, methadone, morphine, oxycodone).Pergolizzi J, Böger RH, Budd K, Dahan A, Erdine S, Hans G, Kress HG, Langford R, Likar R, Raffa RB, et al. Pain Pract. 2008 Jul-Aug; 8(4):287-313. Epub 2008 May 23.
- Drosophila free-running rhythms require intercellular communication.[PLoS Biol. 2003]Drosophila free-running rhythms require intercellular communication.Peng Y, Stoleru D, Levine JD, Hall JC, Rosbash M. PLoS Biol. 2003 Oct; 1(1):E13. Epub 2003 Sep 15.
- Review Control of Metabolism by Central and Peripheral Clocks in Drosophila.[A Time for Metabolism and Horm...]Review Control of Metabolism by Central and Peripheral Clocks in Drosophila.Sehgal A. A Time for Metabolism and Hormones. 2016
- Genetics Can Be Painless: Molecular Genetic Analysis of Nociception in Drosophil...Genetics Can Be Painless: Molecular Genetic Analysis of Nociception in Drosophila - TRP Ion Channel Function in Sensory Transduction and Cellular Signaling Cascades
- The Ca2+-Activated TRP Channels: TRPM4 and TRPM5 - TRP Ion Channel Function in S...The Ca2+-Activated TRP Channels: TRPM4 and TRPM5 - TRP Ion Channel Function in Sensory Transduction and Cellular Signaling Cascades
Your browsing activity is empty.
Activity recording is turned off.
See more...