NCBI Bookshelf. A service of the National Library of Medicine, National Institutes of Health.
Liedtke WB, Heller S, editors. TRP Ion Channel Function in Sensory Transduction and Cellular Signaling Cascades. Boca Raton (FL): CRC Press/Taylor & Francis; 2007.
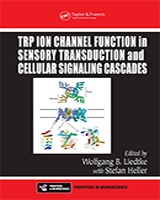
TRP Ion Channel Function in Sensory Transduction and Cellular Signaling Cascades.
Show detailsAbstract
TRPC (canonical transient receptor potential) channels are vertebrate homologues of the Drosophila photoreceptor channel (TRP). Considerable research has been brought to bear on the seven members of this family, especially with regard to their possible roles in calcium entry. Unfortunately, the current literature presents a confusing picture, with different laboratories describing widely differing results and interpretations. It appears that ectopically expressed TRPC channels can be activated as a consequence of phospholipase C activation (by increases in diacylglycerols or by loss of phosphatidylinositol 4,5-bisphosphate), by stimulation of trafficking to the plasma membrane, or by depletion of intracellular Ca2+ stores. These diverse experimental findings arise because TRPC channels can, under both experimental as well as physiological conditions, be activated in three distinct ways, possibly depending on their subunit composition or signaling complex environment. The TRPCs may be unique among ion channel subunit families in having the ability to participate in the assembly and function of multiple types of physiologically important ion channels.
INTRODUCTION
The mammalian TRPC genes were identified from searches for homologues of the Drosophila trp gene [1–10]. The proteins encoded by the TRPC genes, like the parental Drosophila TRP protein, span the membrane six times [11] and contain a short hydrophobic sequence believed to be involved in forming the pore of the channel [12]. By analogy with other similar channels, it is believed that a functional TRPC channel will be formed by the four TRPC proteins coming together [12]; thus channels could be formed as homotetramers, if all four TRPCs are the same, or heterotetramers, if more than one kind of TRPC is involved. There appear to be restrictions as to which TRPCs can come together to form channels, although there is not complete agreement on what these restrictions are [12,13]. Hofmann et al. [12] found that when TRPC channels were exogenously expressed in HEK293 cells, the only permitted combinations were TRPC3, 6, and 7, or TRPC1, 4, and 5. However, a number of reports have found that TRPC1 and 3 can associate, with both exogenous and endogenous expressions [13–15]. Unlike previous studies of ion channel function, investigators attempted to understand the function of mammalian TRPC channels beginning only with knowledge of their coding sequence. There was considerable interest in this group because of the suspicion that they might be the long-sought store-operated or capacitative calcium entry channels [16]. In addition to TRPCs, the TRP superfamily includes two other major families: TRPMs (melastatin-related transient receptor potential channels), related structurally to melastatin, and TRPVs (vanilloid receptor-related transient potential channels), related to the vanilloid receptor, as well as more distantly related genes identified in genetic screens for specific diseases and whose physiological functions are less certain. Considerable progress has been made in our understanding of a number of TRP channels, especially those in the TRPV and TRPM families [17,18], and much of this information is reviewed in other chapters in this book. However, the function and regulation of TRPC channels have been more problematic, plagued with conflicting findings and interpretations. In this review I attempt to organize and rationalize some of these problems. I propose a paradigm that can help to explain the somewhat poorly predictable behavior of these widely expressed cation channels. In addition, I suggest that the apparently poorly predictable behavior of these channels in laboratory experiments might reflect expression of at least three distinct modes of regulation in their native physiological environments. A number of other aspects of TRPC channel regulation and activation mechanisms are reviewed in chapters by Birnbaumer, Gudermann, and Groschner.
TRPCS: MECHANISMS OF REGULATION IN TRANSFECTO
Based on sequence similarities, the seven TRPCs can be conveniently divided into four subgroups: TRPC1, TRPC2, and two additional groups, TRPC3, 6, and 7, and TRPC4 and 5. TRPC1 is most closely related to TRPC4 and 5 and is sometimes considered to be in the same group. TRPC2 is a pseudogene in humans but not in other mammalian species; there is evidence that TRPC2 can function as a store-operated channel in sperm [8,19], and is likely involved in vomeronasal function [20,21]. The body of literature on this channel is small by comparison to other TRPCs, however, and I will not discuss it further here. This topic is covered in the chapter by Dulac and Liman. The majority of research dealing with TRPCs has focused on the TRPC1/4/5 or TRPC3/6/7 groups. To date there is no clear evidence that TRPC5 behaves in a fundamentally different manner from TRPC4, and these two channels appear capable of association with TRPC1. Likewise, when compared side by side, TRPC3, 6, and 7 seem to behave similarly. There are important exceptions to these generalities, however, as noted below.
The TRPCs are so named [22] because this family is most closely related to the original Drosophila channel TRP (i.e., they are the “canonical” TRPs). Because Drosophila TRP was clearly activated downstream of phospholipase C and inositol 1,4,5-trisphosphate (IP3) generation in photoreceptor cells, Hardie and Minke [23] suggested that TRP might be the long-sought store-operated Ca2+ channel. The store-operated or capacitative calcium entry channels are activated by a process triggered by the depletion of intracellular endoplasmic reticulum Ca2+ stores [16,24]. Recent findings indicate that the sensor of intracellular Ca2+ store depletion may be the Ca2+-binding membrane protein, STIM1 [25,26]. Ironically, it is now very clear that store depletion is not the mechanism by which Drosophila TRP is activated in its native environment in photoreceptor cells [27]. The significance of this basic finding to the larger body of work on mammalian TRPCs perhaps should not be overlooked. Yet it is clear from phospholipase C (PLC)-deficient mutants that Drosophila TRP is activated in some manner downstream of PLC. It is most likely, although not proven, that a lipid mediator derived from PLC products activates TRP, perhaps the immediate breakdown product diacylglycerol (DAG), and it is also regulated in complex ways by the substrate of PLC, phosphatidylinositol 4,5-bisphosphate (PIP2) [27].
For the seven mammalian TRPCs, there is evidence that these channels are also activated in some manner downstream of PLC. Although initial studies suggested that TRPC1 and 3 were activated by store depletion [3], it was subsequently shown that activation resulted from the constitutive activity of the channels [28] (however, see reference [29]). One report suggested that IP3 and the IP3 receptor were involved in activating TRPC3 [30], but other laboratories failed to reproduce these results [7,31]. Likewise, the original studies on TRPC4 and TRPC5 presented evidence for activation by store depletion [5,6], but other researchers failed to reproduce these findings [32,33] (but see reference [34]). For the TRPC3/6/7 subfamily, the vast majority of published results suggests that DAG, produced upon phospholipase C activation, is the signal activating these channels [7,9,15,31,35–38]. For TRPC4 and 5, the case is not so clear. TRPC4 and 5 are highly sensitive to inhibition by protein kinase C, making it difficult to obtain evidence that DAG can activate. Indeed, while TRPC3/6/7 channels can be activated by exogenous DAG (usually oleyl acetyl glycerol, or OAG), OAG inhibits the activation of TRPC4 or 5, and this inhibition is blocked by inhibitors of protein kinase C [36]. Thus, if DAG is involved in the activation of TRPC4 or 5, there must be considerable compartmentalization of the signaling pathway to prevent concomitant inhibition by protein kinase C. An interesting possibility is that TRPC4 and 5 may be tonically inhibited by PIP2, such that PLC-mediated degradation of PIP2 relieves this inhibition, resulting in channel activation. Normally, PIP2 is thought to provide a positive regulation of TRPs [39–41]; however, for TRPV1, PIP2 appears to regulate negatively, although this is not thought to constitute a primary mechanism for channel activation [42].
As mentioned above, TRPC1 is often considered with TRPC4 and 5, as it is somewhat similar in sequence and is known to associate with TRPC4 and 5 to form heteromultimeric channels [12,13]. The actions of TRPC1 when expressed on its own are controversial; some researchers demonstrate activated ion channel behavior following expression of TRPC1 [3,4,43–45], while others find that the channel does not traffic to the plasma membrane correctly unless coexpressed with TRPC4 or 5 [12]. Interestingly, and important for arguments to be advanced below, Strübing et al. [46] found that heteromultimers of TRPC1 and TRPC5 had different electrophysiological properties from homotetramers of TRPC5.
A second mechanism that is important for activating TRPC channels involves regulation of their trafficking to the plasma membrane [47–49]. The published reports on this topic are not consistent with one another. However, it is clear from the study of Bezzerides et al. [49] that growth factors (EGF) activate the insertion of TRPC5 channels into the plasma membrane. Once the channels reach the membrane, subsequent maneuvers that activate the channels result in larger currents. Reports from other laboratories indicate that for other TRPCs, the translocation event per se leads to increased currents [47,48]. In either case, either constitutive or stimulated currents would be increased simply by increasing the number of channels and would not necessarily involve any further increase in open probability. The mechanism by which TRPC5 secretion is signaled does not seem to involve activation of PLC; instead it involves a pathway requiring phosphoinositide 3-kinase, Rac1, and phosphatidylinositol 4-kinase [49]. Finally, it is significant that only TRPC5 homotetrameric channels were activated in this manner. Heterotetramers containing TRPC5 and 1 did not translocate to the plasma membrane in response to EGF. This mechanism of regulation may apply to members of the broader TRP superfamily; there is at least one example of a TRPV channel that appears to be regulated in this way [50].
The third major mechanism that has been described for TRPC channel activation is the store-depletion or capacitative calcium entry mechanism. A number of studies have reported activation of TRPC channels by store depletion [3–6,30,38,51–61], and knockout or knock-down of TRPCs often reduces store-operated calcium entry [44,61–69]. Yet in many instances these channels clearly are not store operated [7,9,31,32,35,37,60,70,71]. It is becoming increasingly clear that the basis for the different behaviors is expression conditions. In DT40 B-lymphocytes, TRPC3 formed a store-operated channel at low levels of expression and formed a DAG-activated channel at higher levels of expression [60]. The loss of store-operated behavior at higher expression levels may result from inappropriate stoichiometry among members of a signaling complex [10], as has been argued previously for scaffolding proteins [72,73]. Significantly, the pharmacology of the channels differed in these two modes; the store-operated TRPC3 channels were much more sensitive to block by Gd3+ than the nonstore-operated channels [59]. Both TRPC7 [38] and TRPC5 [34] are store operated when stably expressed in HEK293 cells; in this case, the store-operated channels are either capable of activation by alternative, nonstore-operated mechanisms, or they coexist with channels that are activated by the nonstore-operated mechanisms. For TRPC7, it was demonstrated that this store-operated behavior is only seen with stable transfection. Transient transfection of HEK293 cells with TRPC7 results in channels that can only be activated by receptor activation or OAG, not by store depletion [38].
Thus, the available data demonstrate that, when ectopically expressed, TRPCs can be activated by one of three mechanisms: store depletion, phospholipase C (or its products), or channel translocation to the plasma membrane. The mode of activation seems to depend on the environment in which the subunit finds itself, including perhaps the nature of other subunits that compose the channel pore. This principle is most clearly illustrated by the case of translocation of TRPC5, which only occurs with TRPC5 homotetramers, not with TRPC5/1 heterotetramers [49]. It is also a logical interpretation of the observed difference in Gd3+ sensitivity of TRPC3 channels in store-operated as compared to phospholipase C-activated mode [59]. The question then arises: do these different modes of regulation occur with TRPC subunits when expressed in their native environments in untransfected cells?
MECHANISMS OF TRPC ACTIVATION IN SITU
The answer to that question seems to be, yes. There is now evidence that each of these modes of TRPC function in native, untransfected cells. In the case of store-operated channels, I have already mentioned the considerable number of examples of disrupted behavior of native store-operated channels by knock-down of TRPCs. However, a sticking point has been the failure of ectopically expressed TRPCs (or as yet any expressed ion channel) to faithfully reproduce the electrophysiological characteristics of Icrac (first described in detail in references [74] and [75]; for an example, see also reference [76]). However, it is clear that there are a variety of different kinds of store-operated channels [77–84], some of which have properties reminiscent of TRPCs. It is realistic to expect that TRPCs may contribute to the composition of store-operated channels in such instances. It is not out of the question to consider that TRPCs might play a role in Icrac channels, given the possibility of heteromultimers with drastically altered electrophysiological properties. Two reports have provided evidence for a role for TRPC1 [68] and TRPC3 [85] in Icrac.
There are examples of native channels that appear to be activated by diacylglycerols under physiological conditions [70,71,86–90]. In a particularly thorough study, Inoue et al. [87] investigated the role of TRPC6 in α-adrenergic-activated cation channels. These authors examined the electrophysiological and pharmacological characteristics of the endogenous cation entry controlled by α1-adrenoceptors in rabbit portal vein smooth muscle cells and found this cation entry to be reminiscent of that of the TRPC6 current when transiently expressed in HEK293 cells. Both the endogenous current and the current due to expressed TRPC6 could be activated by OAG in a PKC-independent fashion. Furthermore, TRPC6 mRNA and protein expression in the smooth muscle cells and knock-down of TRPC6 with antisense RNA in smooth muscle cells resulted in almost complete abrogation of the α1-adrenergic response. Similarly, Jung et al. [71] showed that vasopressin activates a non-CCE, nonselective cation current in the smooth muscle cell line, A7r5, resembling that seen with expression of TRPC6 in HEK293 cells. The DAG analog, OAG, activated the endogenous, agonist-sensitive current in A7r5 cells, and those cells were shown to express mRNA for TRPC1 and TRPC6 but not for other TRPC proteins. The authors then concluded that TRPC6 is likely a component of the endogenous agonist-activated channel in the A7r5 cell line.
There are far fewer examples of stimulated translocation of native TRPC channels, likely due to the rather recent discovery of this mode of activation. However, Bezzerides et al. [49] reported that native TRPC5 channels were localized to active growth cones in cultured hippocampal neurons (see also reference [91]). Surface expression of TRPC5 was increased by several different growth factors. TRPC1 was expressed in the cell soma and processes, and not in growth cones, consistent with the observation that only TRPC5 homotetramers undergo regulation through translocation.
SUMMARY
I previously suggested that TRPCs could form distinct types of channels, being regulated by either phospholipase C-dependent or store-depletion mechanisms [10]. Now the number of possible modes of regulation has expanded to three [92] (see Figure 2.1), and we cannot know that others will not be discovered. It is also becoming increasingly clear that this multiplicity of regulatory mechanisms does not simply reflect (at least in all cases) aberrant behavior due to overexpression but rather is indicative of true diversity of channel function in vivo. At least one of the factors that determines the function and regulation of TRPC channels appears to be the subunit composition of the assembled tetrameric channel. Additional factors may include partners in a signaling complex, such as regulatory subunits or scaffolding structures. The propensity of TRPC channels to intereact with the Ca2+ sensor, STIM1 [25,26], may be important for their regulation by Ca2+ store depletion. As argued previously [10], the ability of cells to utilize TRPCs in diverse ways may have significance beyond the ion channel field; such a multiplicity offers a means by which the complex mammalian organism can be assembled from what has turned out to be a surprisingly limited genome.
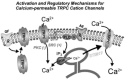
FIGURE 2.1
Activation mechanisms for calcium-permeable TRPC cation channels. TRPC channels can be activated in any of three distinct ways. (From left) Channels sequestered in a vesicular compartment can be translocated to the plasma membrane in response to growth (more...)
ACKNOWLEDGMENTS
The author gratefully acknowledges ideas and criticisms from Mohamed Trebak, Christian Erxleben, and Steve Shears.
REFERENCES
- 1.
- Zhu X, Chu PB, Peyton M, Birnbaumer L. Molecular cloning of a widely expressed human homologue for the Drosophila trp gene. FEBS Letters. 1995;373:193. [PubMed: 7589464]
- 2.
- Wes PD, Chevesich J, Jeromin A, Rosenberg C, Stetten G, Montell C. TRPC1, a human homolog of a Drosophila store-operated channel. Proc Nat Acad Sci USA. 1995;92:9652. [PMC free article: PMC40860] [PubMed: 7568191]
- 3.
- Zhu X, Jiang M, Peyton M, Boulay G, Hurst R, Stefani E, Birnbaumer L. trp, a novel mammalian gene family essential for agonist-activated capacitative Ca2+ entry. Cell. 1996;85:661. [PubMed: 8646775]
- 4.
- Zitt C, Zobel A, Obukhov AG, Harteneck C, Kalkbrenner F, Lückhoff A, Schultz G. Cloning and functional expression of a human Ca2+-permeable cation channel activated by calcium store depletion. Neuron. 1996;16:1189. [PubMed: 8663995]
- 5.
- Philipp S, Cavalié A, Freichel M, Wissenbach U, Zimmer S, Trost C, Marguart A, Murakami M, Flockerzi V. A mammalian capacitative calcium entry channel homologous to Drosophila TRP and TRPL. EMBO J. 1996;15:6166. [PMC free article: PMC452437] [PubMed: 8947038]
- 6.
- Philipp S, Hambrecht J, Braslavski L, Schroth G, Freichel M, Murakami M, Cavalié A, Flockerzi V. A novel capacitative calcium entry channel expressed in excitable cells. EMBO J. 1998;17:4274. [PMC free article: PMC1170761] [PubMed: 9687496]
- 7.
- Hofmann T, Obukhov AG, Schaefer M, Harteneck C, Gudermann T, Schultz G. Direct activation of human TRPC6 and TRPC3 channels by diacylglycerol. Nature. 1999;397:259. [PubMed: 9930701]
- 8.
- Vannier B, Peyton M, Boulay G, Brown D, Qin N, Jiang M, Zhu X, Birnbaumer L. Mouse trp2, the homologue of the human trpc2 pseudogene, encodes mTrp2, a store depletion–activated capacitative Ca2+ channel. Proc Nat Acad Sci USA. 1999;96:2060. [PMC free article: PMC26736] [PubMed: 10051594]
- 9.
- Okada T, Inoue R, Yamazaki K, Maeda A, Kurosaki T, Yamakuni T, Tanaka I, Shimizu S, Ikenaka K, Imoto K, Mori Y. Molecular and functional characterization of a novel mouse transient receptor potential protein homologue TRP7. Ca2+-permeable cation channel that is constitutively activated and enhanced by stimulation of G protein-coupled receptor. J Biol Chem. 1999;274:27359. [PubMed: 10488066]
- 10.
- Putney JW Jr. The enigmatic TRPCs: multifunctional cation channels. Trends Cell Biol. 2004;14:282. [PubMed: 15183184]
- 11.
- Vannier B, Zhu X, Brown D, Birnbaumer L. The membrane topology of human transient receptor potential 3 as inferred from glycosylation-scanning mutagenesis and epitope immunocytochemistry. J Biol Chem. 1998;273:8675. [PubMed: 9535843]
- 12.
- Hofmann T, Schaefer M, Schultz G, Gudermann T. Subunit composition of mammalian transient receptor potential channels in living cells. Proc Nat Acad Sci USA. 2002;99:7461. [PMC free article: PMC124253] [PubMed: 12032305]
- 13.
- Strübing C, Krapivinsky G, Krapivinsky L, Clapham DE. Formation of novel TRPC channels by complex subunit interactions in embryonic brain. J Biol Chem. 2003;278:39014. [PubMed: 12857742]
- 14.
- Xu X-ZS, Li H-S, Guggino WB, Montell C. Coassembly of TRP and TRPL produces a distinct store-operated conductance. Cell. 1997;89:1155. [PubMed: 9215637]
- 15.
- Lintschinger B, Balzer-Geldsetzer M, Baskaran T, Graier WF, Romanin C, Zhu MX, Groschner K. Coassembly of Trp1 and Trp3 proteins generates diacylglycerol- and Ca2+-sensitive cation channels. J Biol Chem. 2000;275:27799. [PubMed: 10882720]
- 16.
- Putney JW Jr. Capacitative Calcium Entry. Landes Biomedical Publishing; Austin, TX: 1997.
- 17.
- Gunthorpe MJ, Benham CD, Randall A, Davis JB. The diversity in the vanilloid (TRPV) receptor family of ion channels. Trends Pharmacol Sci. 2002;23:183. [PubMed: 11931994]
- 18.
- Clapham DE. TRP channels as cellular sensors. Nature. 2003;426:517. [PubMed: 14654832]
- 19.
- Jungnickel MK, Marreo H, Birnbaumer L, Lémos JR, Florman HM. Trp2 regulates entry of Ca2+ into mouse sperm triggered by egg ZP3. Nature Cell Biol. 2001;3:499. [PubMed: 11331878]
- 20.
- Liman ER, Corey DP, Dulac C. TRP2: A candidate transduction channel for mammalian pheromone sensory signaling. Proc Nat Acad Sci USA. 1999;96:5791. [PMC free article: PMC21939] [PubMed: 10318963]
- 21.
- Stowers L, Holy TE, Meister M, Dulac C, Koentges G. Loss of sex discrimination and male-male aggression in mice deficient for TRP2. Science. 2002;295:1493. [PubMed: 11823606]
- 22.
- Montell C, Birnbaumer L, Flockerzi V, Bindels RJ, Bruford EA, Caterina MJ, Clapham DE, Harteneck C, Heller S, Julius D, Kojima I, Mori Y, Penner R, Prawitt D, Scharenberg AM, Schultz G, Shimizu N, Zhu MX. A unified nomenclature for the superfamily of TRP cation channels. Mol Cell. 2002;9:229. [PubMed: 11864597]
- 23.
- Hardie RC, Minke B. Novel Ca2+ channels underlying transduction in Drosophila photoreceptors: implications for phosphoinositide-mediated Ca2+ mobilization. Trends Neurosci. 1993;16:371. [PubMed: 7694408]
- 24.
- Parekh AB, Penner R. Store depletion and calcium influx. Physiol Rev. 1997;77:901. [PubMed: 9354808]
- 25.
- Roos J, DiGregorio PJ, Yeromin AV, Ohlsen K, Lioudyno M, Zhang S, Safrina O, Kozak JA, Wagner SL, Cahalan MD, Velicelebi G, Stauderman KA. STIM1, an essential and conserved component of store-operated Ca2+channel function. J Cell Biol. 2005;169:435. [PMC free article: PMC2171946] [PubMed: 15866891]
- 26.
- Liou J, Kim ML, Heo WD, Jones JT, Myers JW, Ferrell JE Jr, Meyer T. STIM is a Ca2+ sensor essential for Ca2+-store-depletion–triggered Ca2+ influx. Curr Biol. 2005;15:1235. [PMC free article: PMC3186072] [PubMed: 16005298]
- 27.
- Hardie RC. Regulation of trp channels via lipid second messengers. Ann Rev Physiol. 2003;65:735. [PubMed: 12560473]
- 28.
- Zhu X, Jiang M, Birnbaumer L. Receptor-activated Ca2+ influx via human Trp3 stably expressed in human embryonic kidney (HEK)293 cells. Evidence for a non-capacitative calcium entry. J Biol Chem. 1998;273:133. [PubMed: 9417057]
- 29.
- Yildirim E, Kawasaki BT, Birnbaumer L. Molecular cloning of TRPC3a, an N-terminally extended, store-operated variant of the human C3 transient receptor potential channel. Proc Natl Acad Sci USA. 2005;102:3307. [PMC free article: PMC552946] [PubMed: 15728370]
- 30.
- Kiselyov K, Xu X, Mozhayeva G, Kuo T, Pessah I, Mignery G, Zhu X, Birnbaumer L, Muallem S. Functional interaction between InsP3, receptors and store-operated Htrp3 channels. Nature. 1998;396:478. [PubMed: 9853757]
- 31.
- Trebak M, Bird GJ, McKay RR, Birnbaumer L, Putney JW Jr. Signaling mechanism for receptor-activated TRPC3 channels. J Biol Chem. 2003;278:16244. [PubMed: 12606542]
- 32.
- Schaefer M, Plant TD, Obukhov AG, Hofmann T, Gudermann T, Schultz G. Receptor-mediated regulation of the nonselective cation channels TRPC4 and TRPC5. J Biol Chem. 2000;275:17517. [PubMed: 10837492]
- 33.
- McKay RR, Szmeczek-Seay CL, Lièvremont J-P, Bird GJ, Zitt C, Jüngling E, Lückhoff A, Putney JW Jr. Cloning and expression of the human transient receptor potential 4 (TRP4) gene: localization and functional expression of human TRP4 and TRP3. Biochem J. 2000;351:735. [PMC free article: PMC1221414] [PubMed: 11042129]
- 34.
- Zeng F, Xu SZ, Jackson PK, McHugh D, Kumar B, Fountain SJ, Beech DJ. Human TRPC5 channel activated by a multiplicity of signals in a single cell. J Physiol. 2004;559:739. [PMC free article: PMC1665180] [PubMed: 15254149]
- 35.
- Trebak M, Vazquez G, Bird GJ, Putney JW Jr. The TRPC3/6/7 subfamily of cation channels. Cell Calcium. 2003;33:451. [PubMed: 12765690]
- 36.
- Venkatachalam K, Zheng F, Gill DL. Regulation of canonical transient receptor potential (TRPC) channel function by diacylglycerol and protein kinase C. J Biol Chem. 2003;278:29031. [PubMed: 12721302]
- 37.
- Zhang L, Saffen D. Muscarinic acetylcholine receptor regulation of TRP6 Ca2+channel isoforms. J Biol Chem. 2001;276:13331. [PubMed: 11278449]
- 38.
- Lièvremont JP, Bird GS, Putney JW Jr. Canonical transient receptor potential TRPC7 can function as both a receptor- and store-operated channel in HEK-293 cells. Am J Physiol Cell Physiol. 2004;287:C1709. [PubMed: 15342342]
- 39.
- Runnels LW, Yue L, Clapham DE. The TRPM7 channel is inactivated by PIP2 hydrolysis. Nature Cell Biol. 2002;4:329. [PubMed: 11941371]
- 40.
- Liu B, Qin F. Functional control of cold- and menthol-sensitive TRPM8 ion channels by phosphatidylinositol 4,5-bisphosphate. J Neurosci. 2005;25:1674. [PMC free article: PMC6725927] [PubMed: 15716403]
- 41.
- Rohacs T, Lopes CM, Michailidis I, Logothetis DE. PI(4,5)P2 regulates the activation and desensitization of TRPM8 channels through the TRP domain. Nat Neurosci. 2005;8:626. [PubMed: 15852009]
- 42.
- Liu B, Zhang C, Qin F. Functional recovery from desensitization of vanilloid receptor TRPV1 requires resynthesis of phosphatidylinositol 4,5-bisphosphate. J Neurosci. 2005;25:4835. [PMC free article: PMC6724779] [PubMed: 15888659]
- 43.
- Sinkins WG, Estacion M, Schilling WP. Functional expression of TrpC1: a human homologue of the Drosophila Trp channel. Biochem J. 1998;331:331. [PMC free article: PMC1219356] [PubMed: 9512497]
- 44.
- Liu X, Wang W, Singh BB, Lockwich T, Jadlowiec J, O’Connell B, Wellner R, Zhu MX, Ambudkar IS. Trp1, a candidate protein for the store-operated Ca2+ influx mechanism in salivary gland cells. J Biol Chem. 2000;275:3403. [PubMed: 10652333]
- 45.
- Chen J, Barritt GJ. Evidence that TRPC1 (transient receptor potential canonical 1) forms a Ca(2+)-permeable channel linked to the regulation of cell volume in liver cells obtained using small interfering RNA targeted against TRPC1. Biochem J. 2003;373:327. [PMC free article: PMC1223516] [PubMed: 12720547]
- 46.
- Strübing C, Krapivinsky G, Krapivinsky L, Clapham DE. TRPC1 and TRPC5 form a novel cation channel in mammalian brain. Neuron. 2001;29:645. [PubMed: 11301024]
- 47.
- Cayouette S, Lussier MP, Mathieu EL, Bousquet SM, Boulay G. Exocy-totic insertion of TRPC6 channel into the plasma membrane upon Gq protein-coupled receptor activation. J Biol Chem. 2004;279:7241. [PubMed: 14662757]
- 48.
- Singh BB, Lockwich TP, Bandyopadhyay BC, Liu X, Bollimuntha S, Brazer SC, Combs C, Das S, Leenders AG, Sheng ZH, Knepper MA, Ambudkar SV, Ambudkar IS. VAMP2-dependent exocytosis regulates plasma membrane insertion of TRPC3 channels and contributes to agonist-stimulated Ca(2+) influx. Mol Cell. 2004;15:635. [PubMed: 15327778]
- 49.
- Bezzerides VJ, Ramsey IS, Kotecha S, Greka A, Clapham DE. Rapid vesicular translocation and insertion of TRP channels. Nat Cell Biol. 2004;6:709. [PubMed: 15258588]
- 50.
- Kanzaki M, Zhang Y-Q, Mashima H, Li L, Shibata H, Kojima I. Translocation of a calcium-permeable cation channel induced by insulin-like growth factor-I. Nature Cell Biol. 1999;1:165. [PubMed: 10559903]
- 51.
- Vaca L, Sinkins WG, Hu Y, Kunze DL, Schilling WP. Activation of recombinant trp by thapsigargin in Sf9 insect cells. Am J Physiol. 1994;267:C1501. [PubMed: 7977711]
- 52.
- Birnbaumer L, Zhu X, Jiang M, Boulay G, Peyton M, Vannier B, Brown D, Platano D, Sadeghi H, Stefani E, Birnbaumer M. On the molecular basis and regulation of cellular capacitative calcium entry: roles for Trp proteins. Proc Nat Acad Sci USA. 1996;93:15195. [PMC free article: PMC26380] [PubMed: 8986787]
- 53.
- Tomita Y, Kaneko S, Funayama M, Kondo H, Satoh M, Akaike A. Intracellular Ca2+ store-operated influx of Ca2+ through TRP-R, a rat homolog of TRP, expressed in Xenopus oocytes. Neurosci Letters. 1998;248:195. [PubMed: 9654342]
- 54.
- Groschner K, Hingel S, Lintschinger B, Balzer M, Romanin C, Zhu X, Schreibmayer W. Trp proteins form store-operated cation channels in human vascular endothelial cells. FEBS Lett. 1998;437:101. [PubMed: 9804180]
- 55.
- Kiselyov K, Mignery GA, Zhu MX, Muallem S. The N-terminal domain of the IP3 receptor gates store-operated hTrp3 channels. Mol Cell. 1999;4:423. [PubMed: 10518223]
- 56.
- Kinoshita M, Akaike A, Satoh M, Kaneko S. Positive regulation of capacitative Ca2+ entry by intracellular Ca2+ in Xenopus oocytes expressing rat TRP4. Cell Calcium. 2000;28:151. [PubMed: 11020377]
- 57.
- Vazquez G, Lièvremont J-P, Bird GJ, Putney JW Jr. Human Trp3 forms both inositol trisphosphate receptor-dependent and receptor-independent store-operated cation channels in DT40 avian B-lymphocytes. Proc Nat Acad Sci USA. 2001;98:11777. [PMC free article: PMC58806] [PubMed: 11553786]
- 58.
- Riccio A, Mattei C, Kelsell RE, Medhurst AD, Calver AR, Randall AD, Davis JB, Benham CD, Pangalos MN. Cloning and functional expression of human short TRP7, a candidate protein for store-operated Ca2+ influx. J Biol Chem. 2002;277:12302. [PubMed: 11805119]
- 59.
- Trebak M, Bird GJ, McKay RR, Putney JW Jr. Comparison of human TRPC3 channels in receptor-activated and store-operated modes. Differential sensitivity to channel blockers suggests fundamental differences in channel composition. J Biol Chem. 2002;277:21617. [PubMed: 11943785]
- 60.
- Vazquez G, Wedel BJ, Trebak M, Bird GJ, Putney JW Jr. Expression level of TRPC3 channel determines its mechanism of activation. J Biol Chem. 2003;278:21649. [PubMed: 12686562]
- 61.
- Liu X, Singh BB, Ambudkar IS. TRPC1 is required for functional store-operated Ca2+ channels. Role of acidic amino acid residues in the S5-S6 region. J Biol Chem. 2003;278:11337. [PubMed: 12536150]
- 62.
- Brough GH, Wu S, Cioffi D, Moore TM, Li M, Dean N, Stevens T. Contribution of endogenously expressed Trp1 to a Ca2+-selective, store-operated Ca2+ entry pathway. FASEB J. 2001;15:1727. [PubMed: 11481220]
- 63.
- Vandebrouck C, Martin D, Colson-Van Schoor M, Debaix H, Gailly P. Involvement of TRPC in the abnormal calcium influx observed in dystrophic (mdx) mouse skeletal muscle fibers. J Cell Biol. 2002;158:1089. [PMC free article: PMC2173225] [PubMed: 12235126]
- 64.
- Baldi C, Vazquez G, Calvo JC, Boland R. TRPC3-like protein is involved in the capacitative cation entry induced by 1alpha,25-dihydroxy-vitamin D3 in ROS 17/2.8 osteoblastic cells. J Cell Biochem. 2003;90:197. [PubMed: 12938168]
- 65.
- Wang X, Pluznick JL, Wei P, Padanilam BJ, Sansom SC. TRPC4 forms store-operated Ca2+ channels in mouse mesangial cells. Am J Physiol Cell Physiol. 2004;287:C357. [PubMed: 15044151]
- 66.
- Philipp S, Trost C, Warnat J, Rautmann J, Himmerkus N, Schroth G, Kretz O, Nastainczyk W, Cavalié A, Hoth M, Flockerzi V. Trp4 (CCE1) protein is part of native calcium release-activated Ca2+-like channels in adrenal cells. J Biol Chem. 2000;275:23965. [PubMed: 10816590]
- 67.
- Freichel M, Suh SH, Pfeifer A, Schweig U, Trost C, Weißgerber P, Biel M, Philipp S, Freise D, Droogmans G, Hofmann F, Flockerzi V, Nilius B. Lack of an endothelial store-operated Ca2+ current impairs agonist-dependent vasore-laxation in TRP4−/− mice. Nature Cell Biol. 2001;3:121. [PubMed: 11175743]
- 68.
- Mori Y, Wakamori M, Miyakawa T, Hermosura M, Hara Y, Nishida M, Hirose K, Mizushima A, Kurosaki M, Mori E, Gotoh K, Okada T, Fleig A, Penner R, Iino M, Kurosaki T. Transient receptor potential 1 regulates capacitative Ca2+ entry and Ca2+ release from endoplasmic reticulum in B lymphocytes. J Exp Med. 2002;195:673. [PMC free article: PMC2193746] [PubMed: 11901194]
- 69.
- Zagranichnaya TK, Wu X, Villereal ML. Endogenous TRPC1, TRPC3, and TRPC7 proteins combine to form native store-operated channels in HEK-293 cells. J Biol Chem. 2005 [PubMed: 15972814]
- 70.
- Tesfai Y, Brereton HM, Barritt GJ. A diacylglycerol-activated Ca2+ channel in PC12 cells (an adrenal chromaffin cell line) correlates with expression of the TRP-6 (transient receptor potential) protein. Biochem J. 2001;358:717. [PMC free article: PMC1222105] [PubMed: 11535132]
- 71.
- Jung S, Strotmann R, Schultz G, Plant TD. TRPC6 is a candidate channel involved in receptor-stimulated cation currents in A7r5 smooth muscle cells. Am J Physiol. 2002;282:C347. [PubMed: 11788346]
- 72.
- Levchenko A, Bruck J, Sternberg PW. Scaffold proteins may biphasically affect the levels of mitogen-activated protein kinase signaling and reduce its threshold properties. Proc Nat Acad Sci USA. 2000;97:5818. [PMC free article: PMC18517] [PubMed: 10823939]
- 73.
- Burack WR, Shaw AS. Signal transduction: hanging on a scaffold. Current Opinion in Cell Biology. 2000;12:211. [PubMed: 10712921]
- 74.
- Hoth M, Penner R. Depletion of intracellular calcium stores activates a calcium current in mast cells. Nature. 1992;355:353. [PubMed: 1309940]
- 75.
- Hoth M, Penner R. Calcium release-activated calcium current in rat mast cells. J Physiol (Lond ). 1993;465:359. [PMC free article: PMC1175434] [PubMed: 8229840]
- 76.
- Voets T, Prenen J, Fleig A, Vennekens R, Watanabe H, Hoenderop JGJ, Bindels RJM, Droogmans G, Penner R, Nilius B. CaT1 and the calcium release-activated calcium channel manifest distinct pore properties. J Biol Chem. 2001;276:47767. [PubMed: 11687570]
- 77.
- Zhang H, Inazu M, Weir B, Buchanan M, Daniel E. Cyclopiazonic acid stimulates Ca2+ influx through nonspecific cation channels in endothelial cells. Eur J Pharmacol. 1994;251:119. [PubMed: 7512038]
- 78.
- Krause E, Pfeiffer F, Schmid A, Schulz I. Depletion of intracellular calcium stores activates a calcium-conducting nonselective cation current in mouse pancreatic acinar cells. J Biol Chem. 1996;271:32523. [PubMed: 8955076]
- 79.
- Wayman CP, Wallace P, Gibson A, McFadzean I. Correlation between store-operated cation current and capacitative Ca2+ influx in smooth muscle cells from mouse anococcygeus. Eur J Pharmacol. 1999;376:325. [PubMed: 10448895]
- 80.
- Trepakova ES, Gericke M, Hirakawa Y, Weisbrod RM, Cohen RA, Bolotina VM. Properties of a native cation channel activated by Ca2+ store depletion in vascular smooth muscle cells. J Biol Chem. 2001;276:7782. [PubMed: 11113149]
- 81.
- McDaniel S, Platoshyn O, Wang J, Yu Y, Sweeney M, Krick S, Rubin LJ, Yuan JXJ. Capacitative Ca2+ entry in agonist-induced pulmonary vasoconstric-tion. Am J Physiol. 2001;280:L870. [PubMed: 11290510]
- 82.
- Albert AP, Large WA. A Ca2+-permeable nonselective cation channel activated by depletion of internal Ca2+ stores in single rabbit portal vein myocytes. J Physiol (Lond ). 2002;538:717. [PMC free article: PMC2290110] [PubMed: 11826160]
- 83.
- Liu X, Groschner K, Ambudkar IS. Distinct Ca(2+)-permeable cation currents are activated by internal Ca(2+)-store depletion in RBL-2H3 cells and human salivary gland cells, HSG and HSY. J Membr Biol. 2004;200:93. [PubMed: 15520907]
- 84.
- Gusev K, Glouchankova L, Zubov A, Kaznacheyeva E, Wang Z, Bezprozvanny I, Mozhayeva GN. The store-operated calcium entry pathways in human carcinoma A431 cells: functional properties and activation mechanisms. J Gen Physiol. 2003;122:81. [PMC free article: PMC2234469] [PubMed: 12835472]
- 85.
- Philipp S, Strauss B, Hirnet D, Wissenbach U, Mery L, Flockerzi V, Hoth M. TRPC3 mediates T-cell receptor-dependent calcium entry in human T-lymphocytes. J Biol Chem. 2003;278:26629. [PubMed: 12736256]
- 86.
- Hassock SR, Zhu MX, Trost C, Flockerzi V, Authi KS. Expression and role of TRPC proteins in human platelets: evidence that TRPC6 forms the store-independent calcium entry channel. Blood. 2002;100:2801. [PubMed: 12351388]
- 87.
- Inoue R, Okada T, Onoue H, Hara Y, Shimizu S, Naitoh S, Ito Y, Mori Y. The transient receptor potential protein homologue TRP6 is the essential component of vascular α1-adrenoceptor-activated Ca2+-permeable cation channel. Circ Res. 2001;88:325. [PubMed: 11179201]
- 88.
- Gamberucci A, Giurisato E, Pizzo P, Tassi M, Giunti R, McIntosh DP, Benedetti A. Diacylglycerol activates the influx of extracellular cations in T-lymphocytes independently of intracellular calcium-store depletion and possibly involving endogenous TRP6 gene products. Biochem J. 2002;364:245. [PMC free article: PMC1222567] [PubMed: 11988098]
- 89.
- Albert AP, Large WA. Synergism between inositol phosphates and diacylg-lycerol on native TRPC6-like channels in rabbit portal vein myocytes. J Physiol. 2003;552:789. [PMC free article: PMC2343454] [PubMed: 12972630]
- 90.
- Thebault S, Zholos A, Enfissi A, Slomianny C, Dewailly E, Roudbaraki M, Parys J, Prevarskaya N. Receptor-operated Ca(2+) entry mediated by TRPC3/TRPC6 proteins in rat prostate smooth muscle (PS1) cell line. J Cell Physiol. 2005 [PubMed: 15672411]
- 91.
- Greka A, Navarro B, Oancea E, Duggan A, Clapham DE. TRPC5 is a regulator of hippocampal neurite length and growth cone morphology. Nat Neurosci. 2003;6:837. [PubMed: 12858178]
- 92.
- Putney JW Jr. Physiological mechanisms of TRPC activation. Pflugers Arch. 2005 [PubMed: 16133266]
- 93.
- Vazquez G, Wedel BJ, Kawasaki BT, Bird GS, Putney JW Jr. Obligatory role of Src kinase in the signaling mechanism for TRPC3 cation channels. J Biol Chem. 2004;279:40521. [PubMed: 15271991]
- 94.
- Trebak M, Hempel N, Wedel BJ, Smyth JT, Bird GS, Putney JW Jr. Negative regulation of TRPC3 channels by protein kinase C–mediated phosphorylation of serine 712. Mol Pharmacol. 2005;67:558. [PubMed: 15533987]
- 95.
- Ahmmed GU, Mehta D, Vogel S, Holinstat M, Paria BC, Tiruppathi C, Malik AB. Protein kinase C alpha phosphorylates the TRPC1 channel and regulates store-operated Ca2+ entry in endothelial cells. J Biol Chem. 2004;279:20941. [PubMed: 15016832]
- Review Physiological mechanisms of TRPC activation.[Pflugers Arch. 2005]Review Physiological mechanisms of TRPC activation.Putney JW. Pflugers Arch. 2005 Oct; 451(1):29-34. Epub 2005 Aug 18.
- Review Phospholipase C-coupled receptors and activation of TRPC channels.[Handb Exp Pharmacol. 2007]Review Phospholipase C-coupled receptors and activation of TRPC channels.Trebak M, Lemonnier L, Smyth JT, Vazquez G, Putney JW Jr. Handb Exp Pharmacol. 2007; (179):593-614.
- Review The TRPC family of TRP channels: roles inferred (mostly) from knockout mice and relationship to ORAI proteins.[Handb Exp Pharmacol. 2014]Review The TRPC family of TRP channels: roles inferred (mostly) from knockout mice and relationship to ORAI proteins.Liao Y, Abramowitz J, Birnbaumer L. Handb Exp Pharmacol. 2014; 223:1055-75.
- Review Role of TRPC Channels in Store-Operated Calcium Entry.[Adv Exp Med Biol. 2016]Review Role of TRPC Channels in Store-Operated Calcium Entry.Ong HL, de Souza LB, Ambudkar IS. Adv Exp Med Biol. 2016; 898:87-109.
- Review Protein–Protein Interactions in TRPC Channel Complexes.[TRP Ion Channel Function in Se...]Review Protein–Protein Interactions in TRPC Channel Complexes.Eder P, Schindl R, Romanin C, Groschner K. TRP Ion Channel Function in Sensory Transduction and Cellular Signaling Cascades. 2007
- Multiple Mechanisms of TRPC Activation - TRP Ion Channel Function in Sensory Tra...Multiple Mechanisms of TRPC Activation - TRP Ion Channel Function in Sensory Transduction and Cellular Signaling Cascades
- TRPC2 and the Molecular Biology of Pheromone Detection in Mammals - TRP Ion Chan...TRPC2 and the Molecular Biology of Pheromone Detection in Mammals - TRP Ion Channel Function in Sensory Transduction and Cellular Signaling Cascades
Your browsing activity is empty.
Activity recording is turned off.
See more...