NCBI Bookshelf. A service of the National Library of Medicine, National Institutes of Health.
Liedtke WB, Heller S, editors. TRP Ion Channel Function in Sensory Transduction and Cellular Signaling Cascades. Boca Raton (FL): CRC Press/Taylor & Francis; 2007.
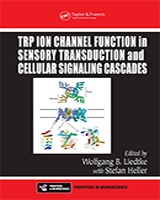
TRP Ion Channel Function in Sensory Transduction and Cellular Signaling Cascades.
Show detailsSTRUCTURAL SCAFFOLD OF TRP CHANNELS
This chapter reviews our knowledge of the three-dimensional structure of TRP channels and how this information relates to channel function and regulation. Sequence analyses have revealed the domain composition of TRP channels. Because no structure has been determined for a complete TRP channel, I will first break down TRP channels into component parts, or building blocks, and then discuss the structural information available for each, using the closest sequence homologues for which structures have been determined.
TRP channels are part of the same channel superfamily as the voltage- and ligand-gated potassium channels [1]. Because the highest sequence similarity between these and TRP channels is found in the transmembrane domain, all TRP channels are expected to form tetramers—homo- or heterotetramers—as functional units, like the voltage- and ligand-gated channels. Biochemical and biophysical analyses have confirmed that several TRP channels are indeed tetrameric (e.g., see reference [2]). The transmembrane domain of each TRP channel subunit is expected to contain six roughly membrane-spanning helical segments, S1 to S6—and therefore both the N-and C- terminal extensions are cytosolic. The transmembrane domain can be divided in two building blocks: the sensor, formed by helices S1–S4, and the pore, formed by helices S5 and S6. The pore forms a hole that spans the lipid membrane and passes ions and other hydrophilic molecules, to which the lipid membrane is otherwise impermeable. The pore contains a selectivity filter, the smallest constriction of pore, which dictates through its stereochemical and electrostatic properties what kind of molecules are allowed through the pore. The sensor perceives the signal(s) and transmits the information to the gate, the channel component that opens or closes the pore.
The cytosolic domains of TRP channels contain regulatory components that can tune the channel opening propensity in a positive or negative fashion. Perhaps some of the most intriguing structural aspects of TRP channels are the diversity of their cytosolic domains. The TRP channel family is divided into seven subfamilies based on sequence similarity and function, and there is little homology in the N- and C-terminal cytosolic regions between subfamilies. Furthermore, while some common protein–protein interaction motifs can be identified in the cytosolic regions, such as ankyrin repeats, calmodulin-binding sites and PDZ domain–binding sites, other cytosolic segments appear novel by sequence analysis. The following sections highlight the current structural information on the TRP channel building blocks within the transmembrane region and the cytosolic domains.
THE TRANSMEMBRANE REGION
The Pore Domain
The putative S5 and S6 segments of TRP channels, upon tetramerization, are expected to form a central ion-conducting pore. The best characterized ion channel pore structure is that of the bacterial KcsA potassium channel [3–5]. Other potassium channels more closely related to TRP channels, including the Kv1.2 Shaker channel, have a central pore structure very similar to that of KcsA [6–8]. Therefore, these structures can be used as a model for the TRP channel pore. The KcsA structure has been described as an inverted teepee [3], with the S5 helices on the outside and inner S6 helices shaping the central pore opening and gate. The S6 helices splay out on the extracellular side of the membrane, leaving space for the S5–S6 linker sequence to shape the selectivity filter by forming a reentrant loop and short pore helix (Figure 25.1A and B). The KcsA selectivity filter sequence, TVGYGD, presents a set of oxygen groups precisely positioned to select for dehydrated K+ ions. Because the transmembrane topology of TRP channels and potassium channels is similar, it can be hypothesized that the S5–S6 linker also forms a reentrant loop shaping the selectivity filter in TRP channels, but that remains an open question requiring structure determination. In fact, the mammalian TRPV1–V4 channels have selectivity filter sequences similar to KcsA: all four channels have a TIG(M/L)GD sequence between their predicted S5 and S6 segments. However, KcsA is much more selective for potassium ions than these TRPV channels. For example, TRPV1 can pass Ca2+ > Mg2+ > Na+ K+ Cs+ and therefore has relatively high permeability for calcium ions (PCa/PNa = 9.60) [9]. How do these TRPV channels achieve the ability to pass diverse cations, both mono- and divalents? One possibility is that their scaffold of oxygens pointing into the pore is similar to KcsA but is more flexible, allowing the size of the cation to vary. Other TRP channels do not have a recognizable selectivity filter sequence homologous to potassium channels, and their linker between the predicted S5 and S6 helices in TRP channels varies greatly in length and sequence (reviewed in reference [10]). Nonetheless, mutagenesis studies on TRPM4 [11], TRPV5 [12], and TRPV6 [13] indicate that the residues in the S5–S6 linker and presumed selectivity filter are important determinants of the ion conductance or selectivity of these channels.
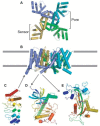
FIGURE 25.1
Structures of TRP channel-building blocks. The transmembrane domain of the Shaker channel (2A79 [8]), viewed from the intracellular side of the membrane (A) and from its side (B). The four subunits have distinct colors, and magenta spheres represent potassium (more...)
The pore of potassium channels is opened by a kink in the S6 helices (Figure 25.1B [8,14]), and this mechanism is likely to be conserved in TRP channels. The TRP box, a short hydrophobic stretch conserved in the TRPC, TRPV, and TRPM subfamilies [15], is located just C-terminal of the putative S6 helix. Secondary structure prediction algorithms often predict that the S6 helix would extend beyond the membrane bilayer in the cytosolic side, including the TRP box. If these predictions are correct, the TRP box could, under some conditions, serve as a coiled-coil zipper that holds the channel in a closed conformation. Although the functional role of the TRP box is unclear, the TRP box regions of TRPM8, TRPV5, and TRPM5 have recently been implicated in sensing phosphatidylinositol 4,5-bisphosphate (PIP2) levels; [16] a depletion of PIP2 caused the channels to close. Following the above structural hypothesis, PIP2 could sensitize channels by destabilizing the coiled coil, allowing other stimuli to stabilize an open channel conformation.
The Sensor Domain
The region encompassing putative transmembrane helices S1–S4 is named the sensor domain by analogy to the Shaker family of ion channels and other related voltage-gated ion channels. The sensor domain of Shaker K+ channels contains multiple buried positively charged residues, which move within the membrane in response to changes in membrane polarization, sensing the voltage difference across the lipid membrane [17–19]. These movements, in turn, are believed to open the gate inside the ion-conducting pore by splaying out the C-terminal and intracellular halves of the four S6 helices [14].
Although there is less information about the location of various sensor modules in TRP channels, the name “sensor” also seems appropriate for the S1–S4 region in TRP channels. In fact, the capsaicin-binding site of TRPV1 has been mapped to the S2 and S3 segments [20], and the icilin-binding site of TRPM8 was mapped to the same region [21]. Capsaicin and icilin induce the opening of the ion-conducting gate of TRPV1 and TRPM8, respectively.
The sensor and pore regions of the potassium channels are clearly separate structural domains within the S1–S6 transmembrane region. This was made apparent in the structure of the bacterial KvAP potassium channel [7] and more recently confirmed in the structure of the Shaker channel [8]. Applying the strict definition of a domain as an independently folding unit is certainly appropriate, because the sensor of KvAP could be expressed, purified, and crystallized as an isolated domain [7]. The observation of two separate transmembrane domains within one protein actually represents a first in membrane protein structural biology and therefore will likely cause a paradigm shift in the way we think about domain units in membrane proteins.
How closely TRP channel sensor domains resemble that of the Shaker channel remains, again, an open question. But considering the sensor as an independent folding unit suggests that it will be possible to determine the structure of isolated sensors in the presence or absence of ligands to explore whether these ligands cause conformational changes within the isolated domain. In analogy to the current model of voltage gating in Shaker channels, it is also possible that the conformational change caused by stimuli consists rather of a rigid body movement of the sensor domain relative to the pore domain.
It has also been reported that some TRP channels, specifically TRPV1, TRPV3, TRPM8, and TRPM4, are quite voltage sensitive, to the point where they could be described as voltage-gated channels with small gating charges [22–24] (reviewed in reference [25]). Voltage-gated potassium channels such as Shaker channels have four positively charged arginines in the S4 segment, which are known to sense transmembrane voltage [17,18]. What are the corresponding voltage-sensitive residues in TRPV1, TRPV3, TRPM8, and TRPM4, and are they located in the sensor domain? TRPV1 does not have a readily identifiable “gating charge” residue in its sensor domain; the predicted S4 segment of TRPV channels does not contain any charged residue. Examining the other predicted transmembrane segments, an arginine-glutamate pair is found in the S2 segment of TRPV1 (R474 and E478 in rat TRPV1). In TRPM channels, however, there are a number of positively and negatively charged residues in the S1–S4 region. Additional studies, including mutational analyses, are required to identify the gating charges in TRP channels and to determine whether they are localized in the S1–S4 region, which is the voltage-sensor domain in other voltage-gated ion channels of this topology.
CYTOSOLIC DOMAINS
Ankyrin Repeats
Ankyrin repeats are present in four subfamilies of TRP channels: the TRPA, TRPC, TRPN, and TRPV subfamilies. First identified in a pair of yeast transcription factors (Sw14 and Sw16), ankyrin repeat sequence motifs are found in over 3,600 proteins from all three kingdoms (bacteria, archea, and eukarya) [26,27]. The functions of ankyrin repeat proteins are diverse, ranging from transcription regulation and signaling to cytoskeleton assembly. The unifying characteristic of ankyrin repeats is that they mediate specific protein–protein interactions. In fact, no enzymatic function has been detected for any ankyrin repeat domain.
The high-resolution structures of ankyrin repeat proteins reveal remarkable structural homology and regularity. The structure of each 33-residue ankyrin repeat sequence motif consists of two antiparallel α-helices followed by a long β-hairpin or “finger” loop projecting outward from the helices at a 90° angle. Consecutive repeats stack together to form a hydrophobic core that promotes protein folding and assembly, yielding an L-shaped elongated domain. The ankyrin repeat serves as a versatile scaffold for protein–protein interactions via nonconserved amino acids in the finger loops [28]. No specific sequences or structural motifs, however, are universally recognized by ankyrin repeat proteins.
The TRPA and TRPN channels, expressed in mechanosensitive hair cells, contain a large number of ankyrin repeats (up to 29 in Drosophila TRPN1 [29]), each of which is close to the ankyrin repeat consensus in sequence and in length. Why are there so many repeats in the TRPA and TRPN channels? A simple answer could be that there are multiple interacting protein targets. Another hypothesis is that a domain containing a large number of ankyrin repeats could behave as a physical spring [30]. Molecular dynamics calculations have lent support to this hypothesis, showing that if a long ankyrin repeat domain is stretched in silico, the extension and stiffness of structures formed of 17 or 24 ankyrin repeats match those predicted for the gating spring in hair cells of the ear [31]. This gating spring hypothesis, which elegantly explains the mechanosensitivity of hair cell conductance, can now be tested through further functional and structural studies of the TRPA and TRPN channels.
In contrast to the TRPA and TRPN channels, the TRPC and TRPV channels contain a smaller number of repeats, four to six, each diverging significantly from the ankyrin repeat sequence consensus defined by analyses of ~4,000 repeats [26]. The shorter ankyrin repeat domains of TRPC and TRPV family members may be protein–protein interaction domains mediating the assembly of signaling modules at the plasma membrane—either to relay signals sensed by the channels or to regulate their propensity to respond to signals. The ankyrin repeat domain of a TRPV channel is important to the integrity of the channel, as its deletion impairs assembly and trafficking to the cell membrane [32–34]. Similarly, deletion of the ankyrin repeats of TRPC1 impairs channel activity [35]. Some candidate binding partners have been identified for TRPV ankyrin repeats. The TRPV1 N-terminus is important for calmodulin regulation of TRPV1 [36] and associates in vitro and in vivo with synaptotagmin IX and snapin, two components of SNARE-dependent exocytosis in excitable cells [37]. Two-hybrid screens have identified proteins that interact with the TRPV2 N-terminus, including the recombinase gene activator (RGA) and Acyl CoA binding domain protein 3 (ACBD3) [38,39]. Similarly, several proteins interact with the N-termini of TRPC channels, including a caveolin interaction with TRPC1 [40], PLC-γ1 with TRPC3 [41], and the dynamin superfamily member MxA with several TRPC channels [42].
The ankyrin repeat domains of TRPV or TRPC channels could also interact intramolecularly with other channel regions, such as the C-terminal tail. Although there is no direct evidence of such an interaction, the expression of a TRPC2 splice variant consisting of the N-terminal cytosolic domain interferes with channel activity [43], suggesting that the N-terminal domain either interacts with some region of the full-length channel or displaces critical interaction partners.
We and others have recently determined crystal structures of the ankyrin repeat domain (ARD) of the TRPV1 and TRPV2 ion channels (Figure 25.1C [44,45]). The TRPV1-ARD and TRPV2-ARD consist of six ankyrin repeat structural motifs, although only four of them are readily identifiable by sequence motif searches. There are several small sequence insertions that affect the overall ARD structure: ankyrin repeats one through three have unusually long and flexible fingers, whereas repeats five and six have unusually long outer helices. Furthermore, a large counterclockwise twist was observed in the stacking of repeats four and five. This twist breaks the regularity of the ankyrin repeat domain, altering the shape of surfaces available for interactions with proteins or other cellular ligands. Both solution studies and crystal-packing interactions indicate that the TRPV1- and TRPV2-ARDs do not form homo-oligomers [44], suggesting that the ARDs may be used for interactions with regulatory factors rather than in promoting tetrameric assembly of the ion channels. We predict that the TRPC channels will have similar ankyrin repeat domains of five or six repeats [44], although the structural details are likely to be different.
The identification of the true structural boundaries of ankyrin repeat domains of TRP channels is important to their functional analyses. Ankyrin repeat domains are known to have highly cooperative folding properties; mutation of a single residue within an individual repeat can affect the entire three-dimensional core structure [26]. Short deletions within a TRP channel ankyrin repeat domain are therefore likely to be deleterious to the folding of the whole domain. In contrast, mutations in predicted finger-loop regions, which are the most common sites of protein–protein interactions for ankyrin repeats, could be very useful in determining binding surfaces and partners.
MHR in TRPM Channels
Unlike the TRPA, TRPC, TRPN, and TRPV channels, which contain ankyrin repeats in their N-terminal cytoplasmic domains, TRPM channels share four regions of high homology (TRPM homology regions—MHRs) in their N-terminal cytoplasmic segments. These MHRs are highly homologous within the TRPM family but have no recognizable sequence homology outside of the TRPM family. Little structural information can be inferred about these regions until their three-dimensional structures are determined. A S141L TRPM6 missense mutation in the MHR1 of TRPM6 causes hereditary hypomagnesemia [46,47] and prevents oligomerization of the channel [48]. Similarly, a splice variant of TRPM1 that contains only the N-terminal segment inhibits the translocation of full-length TRPM1 to the plasma membrane [49]. The MHRs may therefore be involved in the oligomerization of the channels or in regulating transport to the plasma membrane.
Enzymatic Domains
Three TRP channels—TRPM2, TRPM6, and TRPM7—have C-terminal enzymatic domains (recently reviewed in reference [50]). TRPM6 and TRPM7 both feature an α-kinase domain at their C-termini, and the structure of the TRPM7 α-kinase domain has been solved (Figure 25.1D [51]). The role of the kinase domain in the function of TRPM6 and TRPM7 channels and in their regulation by ATP is still a matter of debate [50]. TRPM2 is regulated by ADP ribose through its C-terminal NUDT9 homology domain, which binds ADP ribose and has pyrophosphorylase activity [52]. The structure of human NUDT9 has recently been solved (Figure 25.1E [53]), providing a scaffold for studying the function of the NUDT9 homology domain in TRPM2 [53,54].
The TRPML and TRPP Subfamilies
The TRPP and TRPML channels form distant TRP channel subfamilies, with some homology to TRP channels in their transmembrane domain (recently reviewed in reference [55]). Unlike most TRP channels, the TRPP and TRPML channels do not have very large cytosolic domains and have few recognizable structural motifs in these cytosolic regions. One unique structural feature of the TRPP and TRPML subfamilies is a large extracellular region linking the putative S1 and S2 transmembrane segments. In the TRPP subfamily, this extracellular region contains the “polycystin” motif, although no function has yet been attributed to this conserved region.
OLIGOMERIZATION OF CYTOSOLIC DOMAINS
The structures of cytosolic domains of voltage- and ligand-gated channels have demonstrated that many of these cytosolic domains form oligomers. Most form tetramers with fourfold symmetry, mirroring the tetrameric transmembrane domains. Examples include the T1 domain of Shaker channels [56], the Ca2+-binding domain of Ca2+-gated channels [14], the N- and C-terminal regions of G-protein-coupled inward rectifying potassium channels [57], and the C-terminal cyclic nucleotide-binding domain of a mammalian cyclic nucleotide-gated channel [58]. However, some form dimers instead: the cyclic nucleotide-binding domain of the bacterial cyclic nucleotide-gated channel MlotiK1 is an example of a dimeric domain of a tetrameric potassium channel [59]. In all cases, the oligomerization is thought important for the regulation of the channel, by enabling cooperativity between the subunits through concerted conformational changes.
There are several indications that cytosolic domains of TRP channels may also be oligomers. As stated above, the MHR region of TRPM channels and the N-terminal region of TRPC2 are important for the assembly of the respective channels, suggesting that the regions may themselves tetramerize. Furthermore, the ankyrin repeats of TRPV5 and TRPV6 have also been implicated in oligomerization of the channels [32,33]. Finally, the C-terminal domain of TRPV1 may also oligomerize [60]. In most cases, however, the evidence for—or suggestion of—oligomerization of the cytosolic domains of TRP channels is still only indirect. Studying the oligomerization properties of cytosolic domains can therefore provide important insights into the structure and function of TRP channels and the roles of their incredibly diverse cytosolic domains.
SUMMARY
The structures of TRP channel building blocks or their homologues are proving useful in deciphering the inner workings of TRP channels. However, there is still much to be learned about how these building blocks work together to execute the various physiological functions of this fascinating family of ion channels. This will require the structure determination of complete TRP channels in different conformations representing distinct functional states.
REFERENCES
- 1.
- Harteneck C, Plant TD, Schultz G. From worm to man: three subfamilies of TRP channels. Trends Neurosci. 2000;23(4):159–66. [PubMed: 10717675]
- 2.
- Kedei N, Szabo T, Lile JD, Treanor JJ, Olah Z, Iadarola MJ, Blumberg PM. Analysis of the native quaternary structure of vanilloid receptor 1. J Biol Chem. 2001;276(30):28613–19. [PubMed: 11358970]
- 3.
- Doyle DA, Morais–Cabral J, Pfuetzner RA, Kuo A, Gulbis JM, Cohen SL, Chait BT, MacKinnon R. The structure of the potassium channel: molecular basis of K+ conduction and selectivity. Science. 1998;280(5360):69–77. [PubMed: 9525859]
- 4.
- Zhou Y, Morais-Cabral JH, Kaufman A, MacKinnon R. Chemistry of ion coordination and hydration revealed by a K+ channel—Fab complex at 2.0 Å resolution. Nature. 2001;414(6859):43–48. [PubMed: 11689936]
- 5.
- Morais-Cabral JH, Zhou Y, MacKinnon R. Energetic optimization of ion conduction rate by the K+ selectivity filter. Nature. 2001;414(6859):37–42. [PubMed: 11689935]
- 6.
- Jiang Y, Lee A, Chen J, Cadene M, Chait BT, MacKinnon R. Crystal structure and mechanism of a calcium-gated potassium channel. Nature. 2002;417(6888):515–22. [PubMed: 12037559]
- 7.
- Jiang Y, Lee A, Chen J, Ruta V, Cadene M, Chait BT, MacKinnon R. X-ray structure of a voltage-dependent K+ channel. Nature. 2003;423(6935):33–41. [PubMed: 12721618]
- 8.
- Long SB, Campbell EB, Mackinnon R. Science. 5736. Vol. 309. 2005. Crystal structure of a mammalian voltage-dependent Shaker, family K+ channel; pp. 897–903. [PubMed: 16002581]
- 9.
- Caterina MJ, Schumacher MA, Tominaga M, Rosen TA, Levine JD, Julius D. The capsaicin receptor: a heat-activated ion channel in the pain pathway. Nature. 1997;389(6653):816–24. [PubMed: 9349813]
- 10.
- Voets T, Nilius B. The pore of TRP channels: trivial or neglected. Cell Calcium. 2003;33:5–6. 299–302. [PubMed: 12765676]
- 11.
- Nilius B, Prenen J, Janssens A, Owsianik G, Wang C, Zhu MX, Voets T. The selectivity filter of the cation channel TRPM4. J Biol Chem. 2005;280(24):22899–906. [PubMed: 15845551]
- 12.
- Dodier Y, Banderali U, Klein H, Topalak O, Dafi O, Simoes M, Bernatchez G, Sauve R, Parent L. Outer pore topology of the ECaC–TRPV5 channel by cysteine scan mutagenesis. J Biol Chem. 2004;279(8):6853–62. [PubMed: 14630907]
- 13.
- Voets T, Janssens A, Droogmans G, Nilius B. Outer pore architecture of a Ca2+-selective TRP channel. J Biol Chem. 2004;279(15):15223–30. [PubMed: 14736889]
- 14.
- Jiang Y, Lee A, Chen J, Cadene M, Chait BT, MacKinnon R. The open pore conformation of potassium channels. Nature. 2002;417(6888):523–26. [PubMed: 12037560]
- 15.
- Montell C. Physiology, phylogeny, and functions of the TRP superfamily of cation channels. Science’s STKE. 2001 [PubMed: 11752662]
- 16.
- Rohacs T, Lopes CM, Michailidis I, Logothetis DE. PI(4,5)P2 regulates the activation and desensitization of TRPM8 channels through the TRP domain. Nat Neurosci. 2005;8(5):626–34. [PubMed: 15852009]
- 17.
- Aggarwal SK, MacKinnon R. Neuron. 6. Vol. 16. 1996. Contribution of the S4 segment to gating charge in the Shaker, K+ channel; pp. 1169–77. [PubMed: 8663993]
- 18.
- Seoh SA, Sigg D, Papazian DM, Bezanilla F. Neuron. 6. Vol. 16. 1996. Voltage-sensing residues in the S2 and S4 segments of the Shaker, K+ channel; pp. 1159–67. [PubMed: 8663992]
- 19.
- Tombola F, Pathak MM, Isacoff EY. How far will you go to sense voltage? Neuron. 2005;48(5):719–25. [PubMed: 16337910]
- 20.
- Jordt SE, Julius D. Molecular basis for species-specific sensitivity to ‘‘hot’’ chili peppers. Cell. 2002;108(3):421–30. [PubMed: 11853675]
- 21.
- Chuang H-h, Neuhausser WM, Julius D. The super-cooling agent icilin reveals a mechanism of coincidence detection by a temperature-sensitive TRP channel. Neuron. 2004;43(6):859–69. [PubMed: 15363396]
- 22.
- Voets T, Droogmans G, Wissenbach U, Janssens A, Flockerzi V, Nilius B. The principle of temperature-dependent gating in cold- and heat-sensitive TRP channels. Nature. 2004;430(7001):748–54. [PubMed: 15306801]
- 23.
- Chung MK, Guler AD, Caterina MJ. Biphasic currents evoked by chemical or thermal activation of the heat-gated ion channel, TRPV3. J Biol Chem. 2005;280(16):15928–41. [PubMed: 15722340]
- 24.
- Nilius B, Prenen J, Droogmans G, Voets T, Vennekens R, Freichel M, Wissenbach U, Flockerzi V. Voltage dependence of the Ca2+-activated cation channel TRPM4. J Biol Chem. 2003;278(33):30813–20. [PubMed: 12799367]
- 25.
- Nilius B, Talavera K, Owsianik G, Prenen J, Droogmans G, Voets T. Gating of TRP channels: a voltage connection? J Physiol. 2005;567(Pt. 1):35–44. [PMC free article: PMC1474154] [PubMed: 15878939]
- 26.
- Mosavi LK, Minor DL Jr, Peng ZY. Consensus-derived structural determinants of the ankyrin repeat motif. Proc Natl Acad Sci USA. 2002;99(25):16029–34. [PMC free article: PMC138559] [PubMed: 12461176]
- 27.
- Schultz J, Copley RR, Doerks T, Ponting CP, Bork P. SMART: a web-based tool for the study of genetically mobile domains. Nucleic Acids Res. 2000;28(1):231–34. [PMC free article: PMC102444] [PubMed: 10592234]
- 28.
- Kohl A, Binz HK, Forrer P, Stumpp MT, Pluckthun A, Grutter MG. Designed to be stable: crystal structure of a consensus ankyrin repeat protein. Proc Natl Acad Sci USA. 2003;100(4):1700–1705. [PMC free article: PMC149896] [PubMed: 12566564]
- 29.
- Walker RG, Willingham AT, Zuker CS. A Drosophila mechanosensory transduction channel. Science. 2000;287(5461):2229–34. [PubMed: 10744543]
- 30.
- Howard J, Bechstedt S. Hypothesis: a helix of ankyrin repeats of the NOMPC-TRP ion channel is the gating spring of mechanoreceptors. Curr Biol. 2004;14(6):R224–26. [PubMed: 15043829]
- 31.
- Sotomayor M, Corey DP, Schulten K. In search of the hair-cell gating spring elastic properties of ankyrin and cadherin repeats. Structure (Camb). 2005;13(4):669–82. [PubMed: 15837205]
- 32.
- Chang Q, Gyftogianni E, van de Graaf SF, Hoefs S, Weidema FA, Bindels RJ, Hoenderop JG. Molecular determinants in TRPV5 channel assembly. J Biol Chem. 2004;279(52):54304–11. [PubMed: 15489237]
- 33.
- Erler I, Hirnet D, Wissenbach U, Flockerzi V, Niemeyer BA. Ca2+-selective transient receptor potential V channel architecture and function require a specific ankyrin repeat. J Biol Chem. 2004;279(33):34456–63. [PubMed: 15192090]
- 34.
- Jung J, Lee SY, Hwang SW, Cho H, Shin J, Kang YS, Kim S, Oh U. Agonist recognition sites in the cytosolic tails of vanilloid receptor 1. J Biol Chem. 2002;277(46):44448–54. [PubMed: 12228246]
- 35.
- Engelke M, Friedrich O, Budde P, Schafer C, Niemann U, Zitt C, Jungling E, Rocks O, Luckhoff A, Frey J. Structural domains required for channel function of the mouse transient receptor potential protein homologue TRP1beta. FEBS Lett. 2002;523(1-3):193–99. [PubMed: 12123831]
- 36.
- Rosenbaum T, Gordon-Shaag A, Munari M, Gordon SE. Ca2+/calmodulin modulates TRPV1 activation by capsaicin. J Gen Physiol. 2004;123(1):53–62. [PMC free article: PMC2217413] [PubMed: 14699077]
- 37.
- Morenilla-Palao C, Planells-Cases R, Garcia-Sanz N, Ferrer-Montiel A. Regulated exocytosis contributes to protein kinase C potentiation of vanilloid receptor activity. J Biol Chem. 2004;279(24):25665–72. [PubMed: 15066994]
- 38.
- Barnhill JC, Stokes AJ, Koblan-Huberson M, Shimoda LM, Muraguchi A, Adra CN, Turner H. RGA protein associates with a TRPV ion channel during biosynthesis and trafficking. J Cell Biochem. 2004;91(4):808–20. [PubMed: 14991772]
- 39.
- Stokes AJ, Wakano C, Del Carmen KA, Koblan-Huberson M, Turner H. Formation of a physiological complex between TRPV2 and RGA protein promotes cell surface expression of TRPV2. J Cell Biochem. 2005;94(4):669–83. [PubMed: 15547947]
- 40.
- Brazer SC, Singh BB, Liu X, Swaim W, Ambudkar IS. Caveolin-1 contributes to assembly of store-operated Ca2+ influx channels by regulating plasma membrane localization of TRPC1. J Biol Chem. 2003;278(29):27208–15. [PMC free article: PMC3621139] [PubMed: 12732636]
- 41.
- van Rossum DB, Patterson RL, Sharma S, Barrow RK, Kornberg M, Gill DL, Snyder SH. Phospholipase C[gamma]1 controls surface expression of TRPC3 through an intermolecular PH domain. Nature. 2005;434(7029):99–104. [PubMed: 15744307]
- 42.
- Lussier MP, Cayouette S, Lepage PK, Bernier CL, Francoeur N, St-Hilaire M, Pinard M, Boulay G. MxA, a member of the dynamin superfamily, interacts with the ankyrin-like repeat domain of TRPC. J Biol Chem. 2005;280(19):19393–19400. [PubMed: 15757897]
- 43.
- Chu X, Tong Q, Wozney J, Zhang W, Cheung JY, Conrad K, Mazack V, Stahl R, Barber DL, Miller BA. Identification of an N-terminal TRPC2 splice variant which inhibits calcium influx. Cell Calcium. 2005;37(2):173–82. [PubMed: 15589997]
- 44.
- Jin X, Touhey J, Gaudet R. Structure of the N-terminal ankyrin repeat domain of the TRPV2 ion channel. J Biol Chem. 2006 [PubMed: 16809337]
- 45.
- McCleverty C, Koesema E, Patapoutian A, Lesley SA, Kreusch A. Crystal structure of the human TRPV2 channel ankyrin repeat domain. Protein Science. 2006 [PMC free article: PMC2242602] [PubMed: 16882997]
- 46.
- Walder RY, Landau D, Meyer P, Shalev H, Tsolia M, Borochowitz Z, Boettger MB, Beck GE, Englehardt RK, Carmi R, Sheffield VC. Mutation of TRPM6 causes familial hypomagnesemia with secondary hypocalcemia. Nat Genet. 2002;31(2):171–74. [PubMed: 12032570]
- 47.
- Schlingmann KP, Weber S, Peters M, Niemann Nejsum L, Vitzthum H, Klingel K, Kratz M, Haddad E, Ristoff E, Dinour D, Syrrou M, Nielsen S, Sassen M, Waldegger S, Seyberth HW, Konrad M. Hypomagnesemia with secondary hypocalcemia is caused by mutations in TRPM6, a new member of the TRPM gene family. Nat Genet. 2002;31(2):166–70. [PubMed: 12032568]
- 48.
- Chubanov V, Waldegger S, Mederos y Schnitzler M, Vitzthum H, Sassen MC, Seyberth HW, Konrad M, Gudermann T. Disruption of TRPM6/TRPM7 complex formation by a mutation in the TRPM6 gene causes hypomagnesemia with secondary hypocalcemia. Proc Natl Acad Sci USA. 2004;101(9):2894–99. [PMC free article: PMC365716] [PubMed: 14976260]
- 49.
- Xu XZ, Moebius F, Gill DL, Montell C. Regulation of melastatin, a TRP-related protein, through interaction with a cytoplasmic isoform. Proc Natl Acad Sci USA. 2001;98(19):10692–97. [PMC free article: PMC58528] [PubMed: 11535825]
- 50.
- Scharenberg A. TRPM2 and TRPM7: channel/enzyme fusions to generate novel intracellular sensors. Pflügers Arch. 2005;451(1):220–27. [PubMed: 16001276]
- 51.
- Yamaguchi H, Matsushita M, Nairn AC, Kuriyan J. Crystal structure of the atypical protein kinase domain of a TRP channel with phosphotransferase activity. Mol Cell. 2001;7(5):1047–57. [PubMed: 11389851]
- 52.
- Perraud AL, Fleig A, Dunn CA, Bagley LA, Launay P, Schmitz C, Stokes AJ, Zhu Q, Bessman MJ, Penner R, Kinet JP, Scharenberg AM. ADP-ribose gating of the calcium-permeable LTRPC2 channel revealed by Nudix motif homology. Nature. 2001;411(6837):595–99. [PubMed: 11385575]
- 53.
- Shen BW, Perraud AL, Scharenberg A, Stoddard BL. The crystal structure and mutational analysis of human NUDT9. J Mol Biol. 2003;332(2):385–98. [PubMed: 12948489]
- 54.
- Kolisek M, Beck A, Fleig A, Penner R. Cyclic ADP-ribose and hydrogen peroxide synergize with ADP-ribose in the activation of TRPM2 channels. Mol Cell. 2005;18(1):61–69. [PubMed: 15808509]
- 55.
- Qian F, Noben-Trauth K. Cellular and molecular function of mucolipins (TRPML) and polycystin 2 (TRPP2). Pflügers Arch. 2005;451(1):277–85. [PubMed: 15971078]
- 56.
- Kreusch A, Pfaffinger PJ, Stevens CF, Choe S. Nature. 6679. Vol. 392. 1998. Crystal structure of the tetramerization domain of the Shaker, potassium channel; pp. 945–48. [PubMed: 9582078]
- 57.
- Nishida M, MacKinnon R. Structural basis of inward rectification: cytoplasmic pore of the G protein-gated inward rectifier GIRK1 at 1.8 A resolution. Cell. 2002;111(7):957–65. [PubMed: 12507423]
- 58.
- Zagotta WN, Olivier NB, Black KD, Young EC, Olson R, Gouaux E. Structural basis for modulation and agonist specificity of HCN pacemaker channels. Nature. 2003;425(6954):200–205. [PubMed: 12968185]
- 59.
- Clayton GM, Silverman WR, Heginbotham L, Morais-Cabral JH. Structural basis of ligand activation in a cyclic nucleotide-regulated potassium channel. Cell. 2004;119(5):615–27. [PubMed: 15550244]
- 60.
- Garcia-Sanz N, Fernandez-Carvajal A, Morenilla-Palao C, Planells-Cases R, Fajardo-Sanchez E, Fernandez-Ballester G, Ferrer-Montiel A. Identification of a tetramerization domain in the C-terminus of the vanilloid receptor. J Neurosci. 2004;24(23):5307–14. [PMC free article: PMC6729306] [PubMed: 15190102]
- Review TRP Channels: What Do They Look Like?[Neurobiology of TRP Channels. ...]Review TRP Channels: What Do They Look Like?Rosasco MG, Gordon SE. Neurobiology of TRP Channels. 2017
- Review Determining the Crystal Structure of TRPV6.[Calcium Entry Channels in Non-...]Review Determining the Crystal Structure of TRPV6.Saotome K, Singh AK, Sobolevsky AI. Calcium Entry Channels in Non-Excitable Cells. 2018
- Review TRP Channels and Pain.[Neurobiology of TRP Channels. ...]Review TRP Channels and Pain.González-Ramírez R, Chen Y, Liedtke WB, Morales-Lázaro SL. Neurobiology of TRP Channels. 2017
- Review Molecular properties of brain sodium channels: an important target for anticonvulsant drugs.[Adv Neurol. 1999]Review Molecular properties of brain sodium channels: an important target for anticonvulsant drugs.Catterall WA. Adv Neurol. 1999; 79:441-56.
- Integration of Shaker-type K+ channel, KAT1, into the endoplasmic reticulum membrane: synergistic insertion of voltage-sensing segments, S3-S4, and independent insertion of pore-forming segments, S5-P-S6.[Proc Natl Acad Sci U S A. 2002]Integration of Shaker-type K+ channel, KAT1, into the endoplasmic reticulum membrane: synergistic insertion of voltage-sensing segments, S3-S4, and independent insertion of pore-forming segments, S5-P-S6.Sato Y, Sakaguchi M, Goshima S, Nakamura T, Uozumi N. Proc Natl Acad Sci U S A. 2002 Jan 8; 99(1):60-5. Epub 2001 Dec 26.
- Structural Insights into the Function of TRP Channels - TRP Ion Channel Function...Structural Insights into the Function of TRP Channels - TRP Ion Channel Function in Sensory Transduction and Cellular Signaling Cascades
- TRP Channel Trafficking - TRP Ion Channel Function in Sensory Transduction and C...TRP Channel Trafficking - TRP Ion Channel Function in Sensory Transduction and Cellular Signaling Cascades
Your browsing activity is empty.
Activity recording is turned off.
See more...