NCBI Bookshelf. A service of the National Library of Medicine, National Institutes of Health.
Liedtke WB, Heller S, editors. TRP Ion Channel Function in Sensory Transduction and Cellular Signaling Cascades. Boca Raton (FL): CRC Press/Taylor & Francis; 2007.
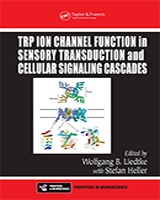
TRP Ion Channel Function in Sensory Transduction and Cellular Signaling Cascades.
Show detailsINTRODUCTION
A subset of sensory neurons is characterized by a unique sensitivity to capsaicin, the piquant ingredient in hot chili peppers [1]. The excitation of these nerves by capsaicin (Figure 6.1) is followed by a lasting and fully reversible refractory state, traditionally referred to as desensitization, or, under certain conditions such as neonatal treatment, by gross neurotoxicity [1]. (Parenthetically, carefully executed studies found no morphologic evidence of neurotoxicity by capsaicin at therapeutic doses [2].) Capsaicin evokes these responses by interacting at a specific membrane recognition site, originally termed the vanilloid receptor [1].
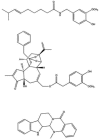
FIGURE 6.1
Typical naturally occurring TRPV1 agonists: capsaicin, resiniferatoxin (RTX), and evodiamine.
The diversity of capsaicin-evoked behavior at the whole animal level has, however, long puzzled scientists. For example, while a clear structure-activity relationship for capsaicin congeners was obtained in the rat eye-wiping assay, it also turned out that pungency was not proportional to the desensitizing effect [3]. Based on these studies, it was postulated that different pharmacophores may be responsible for the excitatory and blocking actions of capsaicinoids [3]. The recognition that resiniferatoxin (RTX; Figure 6.1), a diterpene ester isolated from the latex of the cactuslike plant E. resinifera, functions as an ultrapotent capsaicin analogue with a peculiar spectrum of pharmacological actions has lent further experimental support to this concept [1]. For instance, in the rat RTX can desensitize the pulmonary chemoreflex without any apparent prior excitation, indicating that desensitization may be disconnected from stimulation [4]. This is of great importance, as the initial pain response (excitation) represents the main limitation on the clinical use of vanilloids.
In 1990, specific binding of [3H]RTX provided the first direct proof for the existence of a vanilloid receptor [5]. Structure-activity relationships for binding and 45Ca uptake were, however, found to be dissimilar, giving rise to the concept that these responses were mediated by functionally distinct receptors [6]. The molecular cloning of the rat vanilloid receptor, subsequently renamed as the transient receptor potential vanilloid receptor 1 (TRPV1), provided the opportunity to test this hypothesis [7]. It turned out that binding and 45Ca uptake were both mediated by TRPV1 [8]. With this discovery, the research emphasis has shifted to TRPV1 regulation as the mechanism responsible for the reality of the diversity of vanilloid actions. As discussed below, there is now mounting evidence that TRPV1 regulation is amazingly complex and is manifest at many levels, from gene expression through posttranslational modification and formation of receptor homomers to subcellular compartmentalization and association with regulatory proteins. TRPV1 regulation is still only partially understood. Although this regulation has been reviewed exhaustively, the rapid advances in this field necessitate frequent reevaluation of accepted concepts.
THE VANILLOID RECEPTOR TRPV1 AND ITS ENDOGENOUS LIGANDS, THE “ENDOVANILLOIDS”
TRPV1 is a nonselective cation channel and is a member of the transient release potential (TRP) channel superfamily [7,9]. TRPV1 helps define a subclass of these channels known as TRPV channels, which contain three conserved ankyrin domains. Several conserved protein kinase A (PKA) and protein kinase C (PKC) phosphorylation sites are also observed in TRPV1, and they have important roles in the regulation of receptor functions [7]. In addition to its vanilloid sensitivity, TRPV1 is activated by noxious heat (>43°C), low pH (<6.5), a variety of inflammatory lipid metabolites, and phosphorylation (reviewed in references [10] and [11]). Therefore, the emerging concept is that TRPV1 functions as a general sensor of noxious stimuli (a nociceptor) rather than simply as a specific membrane sensor for compounds carrying a vanillyl moiety [1,9]. (As a matter of fact, this concept is nothing new because this is exactly how N. Jancsó, who almost singlehandedly transformed capsaicin from a pharmacological oddity to a widely used tool to study a specific subset of sensory neurons, had defined the capsaicin receptor [12].)
In a much simplified way, TRPV1 can be thought of as a membrane “sensor” that is gated by heat [7,9]. High noxious heat (>43°C) opens the channel on its own power, whereas some ingredients in the inflammatory “soup” act in concert to lower the heat activation threshold of TRPV1 to (below) body temperature [9,11]. This latter effect is mimicked by piquant agents like capsaicin [7] and piperine (black pepper) [13], thus explaining why we feel these spices as “hot.” Capsaicin acts as a “gating modifier” that shifts activation curves toward physiological membrane potentials [14]. Interestingly, TRPV1 also causes intracellular acidification at neutral pH via a “proton hopping permeation mechanism.” [15] More generally, TRPV1 is simply a channel, and its gating is sensitive to the integrated influence of its large number of regulatory factors.
Moreover, it seems clear that TRPV1’s behavior does not reflect the binary choice between two possible states. Rather, different ligands and regulatory factors shift its behavior in different ways.
Among the newly discovered vanilloids, anandamide (N-arachidonoyl-ethanolamine) [16] is no doubt the most controversial. Anandamide is an endogenous eicosanoid, which preferentially acts on cannabinoid CB1 receptors: that is, an “endocannabinoid.” Anandamide is formed “on demand” from the hydrolysis of phospholipid precursors, catalyzed by phospholipase D. Anandamide was reported to act as a full agonist at both heterologously expressed and native rat [17] and human [18] TRPV1. This was, however, an apparently low affinity interaction, resulting in understandable reluctance in accepting anandamide as a possible “endovanilloid.” New insights, however, enhance the possibility of anandamide being a potent “endovanilloid.” Now it is highly likely that the anandamide-binding site on TRPV1 is intracellular [19]. Anandamide is transported through the membrane via a specific transporter. The potency of anandamide at VR1 is enhanced by coapplication of other TRPV1 agonists such as low pH and increased temperature [20–22]. Agents, like nitric oxide (NO), that stimulate the anandamide membrane transporter enhance the apparent potency of anandamide at TRPV1 [23]. This recognition has exciting implications. Cannabinoid CB1 and vanilloid TRPV1 receptors are coexpressed on a subset of sensory neurons [24]. Generally speaking, agents that activate TRPV1 are excitatory, whereas those acting on CB1 inhibit neuronal activity [25]. The binding site for anandamide on TRPV1 is intracellular; [19] the anandamide recognition domain on CB1 is, in contrast, extracellular. Thus, anandamide may have opposing actions on the very same nerve terminal depending on the functional state of its membrane transporter [25]. Indeed, both excitation (via TRPV1) and inhibition (via CB1) of primary sensory neurons by anandamide have been described [25]. Such a mechanism may account for the bell-shaped dose-response curve for some TRPV1 agonists [26].
Other “endovanilloids” were also identified. The first example is N-arachidonoyl-dopamine (NADA) [27], a brain substance that is similar to capsaicin not only structurally but also with regard to its potency at TRPV1. Certain lipoxygenase metabolites, such as 12-HPETE, have also been identified as putative endogenous TRPV1 agonists [28]. These findings may provide a mechanistic link between TRPV1 and the 5-lipoxygenase products that are important mediators of airway inflammation.
Ethanol has also been added to the list of controversial vanilloids [29]. High concentrations of ethanol activate C-fibers innervating the esophagus and the skin, evoking neuropeptide release and resultant neurogenic inflammation [29]. Ethanol also excites dorsal root ganglion neurons possessing native vanilloid receptors as well as HEK cells transfected with TRPV1 [29]. This excitation by ethanol is abolished by the coadministration of capsazepine (Figure 6.2). Even more interesting, ethanol lowers the heat threshold of TRPV1 activation from 42°C to 34°C [29].
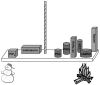
FIGURE 6.2
TRPV1 activation represents the balance between factors favoring its open (active) and closed (resting) states.
TRPV1 IN THE RESTING STATE
There is mounting evidence that TRPV1 is balanced on the edge between open and closed states (Figure 6.2). Agents that promote the open state are nociceptive [11,25]. By contrast, agents that shift TRPV1 toward the closed state are antinociceptive [11]. As of today, phosphatidylinositol(4,5)-bisphosphate (PIP2) is the only known endogenous ligand that helps keep TRPV1 in the closed state [30]. Functional recovery from desensitization of TRPV1 depends on the replenishment of PIP2 [31]. The inhibitory control of PIP2 is facilitated by adenosine [32]. By contrast, ATP activates TRPV1 [33].
Of course, to the degree that TRPV1 is sensitized by phosphorylation by its many regulatory kinases such as PKC or PKA, the activity of protein phosphatases such as calcineurin will exert an inhibitory, antinociceptive effect [34].
MOLECULAR ACTIVATORS OF TRPV1
Agents that favor the open state of TRPV1 are numerous and can be divided into two major categories: (1) agents that increase the probability of channel opening directly, and (2) agents that release TRPV1 from the inhibitory control of PIP2. The first category is exemplified by the enzymes PKC and PKA (reviewed in reference [11]). Factors that stimulate phospholipase C, the enzyme that cleaves PIP2, fall into the second category [30]. However, this category is typically not independent of the first, because the breakdown of PIP2 by phospholipase C is linked to formation of diacylglycerol, the endogenous activator for PKC [35].
Bradykinin (acting on B2 receptors) thus has a dual effect on TRPV1: it stimulates PKC by promoting diacylglycerol formation and, at the same time, it degrades PIP2 by facilitating phospholipase C [30]. It is tempting to speculate that this dual action on TRPV1 gives an important contribution to the profound algesic activity of bradykinin. Nerve growth factor (NGF), a key player in inflammatory hyperalgesia, also releases TRPV1 under the inhibitory control of PIP2 [30].
REGULATION BY NGF OF TRPV1 MAY PLAY A CENTRAL ROLE IN INFLAMMATORY HYPERALGESIA
In addition to sensitizing TRPV1 by cleaving PIP2 [30], NGF may also regulate the transcription of the TRPV1 gene. It has long been known that neuropeptides are depleted from sensory neurons following capsaicin desensitization, and it was speculated that this effect is secondary to the disruption by capsaicin of centripetal axonal NGF transport from the periphery to the perikarya (reviewed in reference [1]). Because the loss and recovery of specific RTX binding sites parallel the changes in neuropeptide expression [36], it is not unlikely that NGF is also required for the transcription of the TRPV1 gene. The action of NGF on TRPV1 expression may even be bidirectional. It is well documented that NGF levels are elevated under inflammatory conditions [37] where TRPV1 is overexpressed both in animal models and human disease states. Studies with TRPV1-deficient (−/−) mice have furnished experimental proof that TRPV1 is instrumental in the development and maintenance of certain types of inflammatory hyperalgesia [38,39]. Thus, it may be speculated that NGF may play a pivotal role in causing inflammatory hyperalgesia by elevating TRPV1 levels [40] and sensitizing the receptor [30] at the same time. If so, the pharmacological manipulation of NGF–TRPV1 interactions may represent a novel therapeutic approach for the relief of inflammatory pain.
MODULATION OF TRPV1 BY PROTEIN KINASES
PKC is central to TRPV1 regulation inasmuch as it couples an array of receptors to TRPV1 (reviewed in reference [11]). Some examples were discussed above. Other notable examples include the chemokine receptor CCR1 [41], the metabotropic puri-nergic receptor P2Y [42], and the prostaglandin receptors EP1 and IP [43].
PKA and PKC are, however, not the only kinases to regulate TRPV1. The Ca2+/calmodulin-dependent kinase II (CaMKII) sensitizes TRPV1 by phosphorylation [44] as does phophatidylinositol 3-kinase (PI3K) via its downstream target AKT [45]. This latter finding links TRPV1 to the ERK (extracellular signal-regulated protein kinase) pathway. The nonreceptor tyrosine kinase Src likewise potentiates capsai-cin-induced currents [46].
SHUFFLING OF TRPV1 AMONG VARIOUS SUBCELLULAR COMPARTMENTS
TRPV1 appears to be mostly sequestered in intracellular compartments where it exists in a homomeric complex, most likely as a tetramer [10]. In fact, a tetramerization domain is present in the C-terminus of TRPV1 [47]. Formation of heteromers with related TRP channels like TRPV2 was also described [48]. Moreover, TRPV1 is believed to be associated with cytoplasmic proteins that can further fine-tune the channel’s activity. An example of this phenomenon is interaction with β-tubulin, for which TRPV1 carries a binding domain on its C-terminus [49].
Upon activation, neurons begin trafficking TRPV1 to the membrane, where this receptor gets activated, desensitized, and then “recycled” to the intracellular compartments. Translocation of TRPV1 to the cell membrane occurs via SNARE (snapin and synaptotagmin IX)-mediated exocytosis [50]. Broadly speaking, activation involves phosphorylation by protein kinases (both PKA and PKC), and desensitization involves dephosphorylation by phosphatases (e.g., calcineurin; reviewed in references [10] and [11]).
REGULATION OF TRPV1 BY MAST CELLS
An added level of TRPV1 regulation is by inflammatory cells such as mast cells. Mast cells release tryptase that, in turn, activates the protease-activated receptor PAR-2; activation of PAR-2 then opens TRPV1 via PKC [51]. In keeping with this, PAR-2 agonists reduce the heat activation threshold of TRPV1 from 42°C to below body temperature [52]. Excited nerve endings release SP that, as a positive feedback, binds to neurokinin NK1 receptors on mast cells. Mast cells also express TRPV1 [53]. A relevant finding is that PAR-2 is upregulated in the bladder during experimental cystitis [54].
HOW CAN A SINGLE RECEPTOR MEDIATE RESPONSES WITH DISSIMILAR STRUCTURE-ACTIVITY RELATIONS?
A combination of pharmacokinetics and differential receptor regulation may explain the strikingly different structure-activity relations for vanilloid-induced Ca2+-uptake versus inhibition of [3H]RTX binding. Recently, no fewer than five parameters (potency, maximal response, latency of response, variability in latency, and desensitization) were identified in which TRPV1 agonists differ even in a simple in vitro assay like calcium response in CHO cells transfected with rTRPV1 [55]. For the most part, the correlation between such in vitro parameters and in vivo responses has not been defined.
The mapping of pharmacologically “hot” points that may account for this differential regulation is in progress. For example, Thr370 is the key residue that is phosphorylated by PKC (sensitization) and dephosphorylated by calcineurin (desensitization) [56]. Other residues phosphorylated by PKC include Ser502 and Ser800 [57]. High affinity [3H]RTX binding has been linked to a single residue, Met547, in the S4 membrane domain, which is believed to form a “binding pocket” with Tyr511 in the S3 domain [58]. It is also possible that binding and Ca2+ uptake detect two distinct populations of TRPV1: binding may predominantly detect receptors sequestered in intracellular compartments, whereas the Ca2+-uptake response reflects activated TRPV1, transported into the plasma membrane. As these receptor populations are in different subcellular milieu, their pharmacology can be distinct.
DIVERSITY OF BEHAVIOR OF EXOGENOUS LIGANDS
The rapid advances in the characterization of TRPV1 have been matched by intense efforts to develop therapeutics targeted to TRPV1. These medicinal chemistry efforts have again revealed great diversity. From an early concept that the vanilloid moiety shared by capsaicin and RTX (compare structures in Figure 6.1) was a critical feature of ligands for the “vanilloid receptor,” [1] it is now apparent that a wide variety of structures can interact with high affinity. Likewise, the initial identification of vanil-loid agonists such as capsaicin, RTX, and evodiamine is now complemented by an impressive variety of potent antagonists for TRPV1, acting competitively with cap-saicin (reviewed in reference [59]; for selected structures, see Figure 6.3). Such antagonists functionally display at least two different behavior patterns. Some are competitive with capsaicin but are less effective for antagonizing TRPV1 activation by other regulators such as pH and temperature. Others block all three responses. A rationale for this difference is that the former may simply occupy the capsaicin-binding site, whereas for the latter class occupancy is coupled to stabilization of the closed conformation of the channel (Figure 6.4).
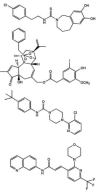
FIGURE 6.3
Selected examples of TRPV1 antagonists: capsazepine, iodo-RTX, BCTC, and N-arylcinnamides (AMG 9810 analogue).
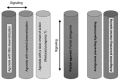
FIGURE 6.4
The diverse mechanisms of action of TRPV1 ligands.
Between antagonists and agonists are positioned another class of ligands: competitive partial agonists/partial antagonists (Figure 6.4). Although this class has received relatively little attention, such compounds may be of particular potential interest because the ratio of agonism to antagonism is not fixed but rather is subject to modulation by the regulatory environment in which TRPV1 is found (Figure 6.5). It may be possible to design ligands that only function as agonists and thereby induce desensitization only in a specific environment. If so, they could represent agents that might be administered systemically to achieve a localized effect.
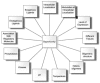
FIGURE 6.5
Opportunities for modulating TRPV1 by interfering with its environmental regulation.
Such compounds illustrate the potential of diversity within the TRPV1 environment to control efficacy of ligands (Figure 6.5). The evidence for different structure-activity relations for different vanilloid responses also implies strongly that the TRPV1 environment can play a major role in determining structure-activity relations for potency. Although this concept does not appear to have been factored into current screening of the output from programs in medicinal chemistry, it again suggests that it may be possible to optimize vanilloid design not simply for TRPV1 but rather for TRPV1 as immersed in a specific regulatory environment (Figure 6.5).
TISSUE AND CELLULAR EXPRESSION OF VR1 AND ITS SPLICE VARIANTS
TRPV1 is expressed in small, unmyelinated sensory nerve fibers called C fibers, which conduct very slow action potentials and contain various neuropeptides including CGRP and SP (reviewed in reference [1]). These TRPV1 immunoreactive positive fibers have been observed innervating the skin, the bladder, and the gastric mucosa, just to cite a few examples. TRPV1 RNA and immunoreactivity are also found in the central nervous system, albeit at much lower levels than found in dorsal root ganglia (reviewed in reference [60]).
There is good evidence for TRPV1 gene expression in other nonneural tissues as well, including human skin (keratinocytes, dermal blood vessels, hair follicles, sebocytes, and sweat glands [53,61]), the bladder (urothelium, smooth muscle, mast cells, and capillaries [62]), and the brain microvasculature endothelium [63]. TRPV1 in these tissues is functional, lending experimental foundation to the novel concept that both squamous and transitional epithelia can play sensory roles [64]. In animals, the tissue distribution of TRPV1 is even broader, encompassing hearts, livers, kidneys, spleens, and lungs [65–68]. Of note, rat cardiomyocytes express TRPV1 in neonates, but not in adults, suggesting that TRPV1 expression is developmentally regulated, at least in the heart [65]. The identification of CNS and peripheral nonneuronal sites of TRPV1 expression negates one of the central dogmas of the field (that is, vanilloid receptor expression is a functional signature of primary sensory neurons) and should revolutionize the ways we think about vanilloid receptors.
POSSIBLE REGULATION OF TRPV1 BY SPLICE VARIANTS
Several splice variants of VR1 have been reported. One that is called the rat TRPV1 5’ splice variant (VR1 5’sv) differs from TRPV1 only in the amino terminal tail region [69]. A similar, but not identical, variant has also been isolated from mice and is called TRPV1b [70]. Neither VR1 5’sv nor TRPV1b is sensitive to capsaicin or low pH; interestingly, TRPV1b may be activated by high temperature [70]. Given the hypothesis that TRPV1 is a tetramer, it is tempting to speculate that these splice variants may form heteromeric channels with wild-type TRPV1, and they may function as dominant negative regulators. VR1 5’sv RNA is expressed at very low levels in sensory neurons relative to TRPV1 but is comparable to TRPV1 gene expression in the CNS [66].
REGULATION OF TRPV1 BY SERUM STEROIDS
Several endogenous steroids can also bind and inhibit TRPV1. For example, dehy-droepiandrosterone (DHEA), a major blood steroid, can reversibly inhibit capsaicin- induced currents in dorsal root ganglion neurons with an EC50 value of 6.7μM [71], which is close to the physiological concentration of this compound. Because DHEA levels climax in the mid-twenties and then decrease with age, elderly people might be more sensitive to capsaicin than young adults. Even more interesting, distinct structure-activity relationships were discovered for steroids: for instance, 3-epiDHEA could potentiate, and not inhibit, capsaicin responses [71], raising the possibility that the steroid framework might provide an interesting platform for the discovery of new TRPV1 antagonists.
At a molecular level, it is not clear if steroids are allosteric modulators of TRPV1 or if they bind directly to the capsaicin-binding domain of the receptor. For example, pregnenolone appears to have a noncompetitive mechanism [72].
Another intriguing observation is that the female sex hormone 17-estradiol could dramatically potentiate capsaicin responses, whereas the male hormone testosterone had marginal inhibitory activity [73]. Sex differences in pain responses have long been known, with women being more sensitive to capsaicin-induced pain than men [74]. The differential modulation of capsaicin responses by female and male hormones might explain this observation.
REGULATION OF TRPV1 BY (NEURO)ENDOCRINE FACTORS
There is a growing body of evidence that TRPV1 is also subject to (neuro)endocrine regulation. An interesting example of this phenomenon is insulin. TRPV1 is expressed on pancreatic islet cells where it is involved in insulin release [75]. Circulating insulin, in turn, may promote translocation of TRPV1 from cytosol to plasma membrane in neurons, leading to sensitization.
TRPV1 MAY SUBSERVE OPPOSING ACTIONS AT THE WHOLE ANIMAL LEVEL
Recently, chronic mechanical hyperalgesia was compared in wild-type (WT) and TRPV1-deficient mice in animal models of human diabetic (streptozoticin-induced) and toxic polyneuropathic (cisplatin-evoked) pain. Surprisingly, TRPV1 knockout mice fared worse: hyperalgesia both developed earlier and was more severe in TRPV1-deficient mice compared to WT animals [76]. By contrast, no difference was found between the two groups following carrageenan treatment or mechanical injury [76]. Two alternative, but not mutually exclusive, mechanisms can account for the protective role of TRPV1 against certain sources of hyperalgesia. First, activation of TRPV1 by endovanilloids may release endogenous analgesic substances like somatostatin [76]. Second, such endovanilloids that possess analgesic actions can be generated [77].
TRPV1 IS COEXPRESSED WITH ITS RELATIVES ON SENSORY NEURONS WITH OVERLAPPING ACTIVITY
TRPV1 has a number of close relatives (belonging to the extended family of TRP receptors): some (similarly to TRPV1) are involved in sensory reception, whereas others have unknown functions. Curiously, the “cool” menthol receptor transient receptor potential melastatin subfamily member 8 (TRPM8) [78] and the noxious cold receptor transient receptor potential subfamily A (ankyrin-like) member 1 (TRPA1) [79] are close relatives of the “hot” capsaicin receptor TRPV1. It is known that mustard oil, traditionally used as a pungent activator of capsaicin-sensitive nerves, in fact acts through TRPA1 [79], providing a rationale to explain how noxious cold can paradoxically be perceived as burning pain. Of note, both TRPV1 [30] and TRPA1 [79] are downstream targets for bradykinin.
PHARMACOLOGICAL OVERLAP BETWEEN TRPV1 AND ITS RELATIVES
TRPM8 shares many functional and pharmacological properties with TRPV1: for instance, both receptors are under the inhibitory control of PIP2 [30,78]. The pharma-cological overlap between TRPM8 and TRPV1 is extensive [80] and includes the “selective TRPV1 antagonists” N-(4-tertiarybutylphenyl)-4-(3-chloropyridin-2-yl) tetrahydropyrazine-1(2H)-carboxamide (BCTC; Figure 6.3), (2R)-4-(3-chloro-2-pyridinyl)-2-methyl-N-(4-[trifluoromethyl]phenyl)-1-piperazinecarboxamide (CTPC), and N-(2-bromophenyl)-N’-(2-[ethyl{3-methylphenyl}amino]ethyl)-urea (SB-452533). TRPM8 and TRPV1 are colocalized in many tissues, including prostate cancer cells [81]. It was postulated that TRPM8 is required for cell survival [82], whereas TRPV1 promotes apoptosis [83]. If this hypothesis holds true, it is impossible to predict whether an antagonist that blocks both TRPM8 and TRPV1 would inhibit or accelerate prostate cancer growth.
TRPV1 EXPRESSION MAY CHANGE UNDER PATHOLOGICAL CONDITIONS
Several lines of evidence demonstrate that TRPV1 protein levels are regulated. For instance, increased TRPV1 immunoreactive fiber innervation has been observed in inflamed human skin [84], vulvas [85], and gastrointestinal tracts (both in the esophagus [86] and large intestine [87]). This phenomenon is believed to contribute to the pathogenesis of various diseases, such as reflux esophagitis (also known as GERD, or gastroe-sophageal reflux disease), inflammatory bowel disease (both Crohn’s disease and ulcerative colitis), irritable bowel syndrome, vulvar allodynia, and prurigo nodularis (reviewed in reference [88]).
At the cellular level, both up- and downregulation of the TRPV1 gene have been demonstrated. In the rat, reduced levels of TRPV1 mRNA were found following vanilloid desensitization [89] in keeping with the loss of receptor protein (specific [3H]RTX binding) in these animals [36]. By contrast, elevated levels of TRPV1 mRNA and protein were shown in animal models of inflammatory hyperalgesia [90]. The latter finding is in agreement with the increase in TRPV1-like immunoreactivity detected in painful human disease conditions [84–88]. The molecular mediators that regulate the TRPV1 gene are yet to be explored but, as discussed above, NGF is an important candidate molecule.
A new finding with far-reaching implications is the demonstration of “aberrant” TRPV1 expression in cervical carcinoma cells [91], implying a future role for TRPV1 agonists as adjuvant chemotherapeutic drugs in cancer treatment regimens.
The presence of neuronal capsaicin receptors in human airways is long established based on both functional studies and RTX binding experiments, but it is a recent recognition that lung epithelial cells and bronchial smooth muscle also express TRPV1 [92]. Capsaicin evokes the cough reflex in human volunteers, and the cough response is exaggerated in patients with asthma or chronic obstructive pulmonary disease [93]. Recently, increased levels of TRPV1 were found in airways smooth muscle of patients with chronic cough [94].
Interestingly, the increased TRPV1 immunoreactivity in skin nerve fibers of women with breast pain is accompanied by elevated TRPV3 (the camphor receptor) and TRPV4 levels in keratinocytes [84]. TRPV3-deficient mice show a similar phenotype to TRPV1 knockout mice, including lack of inflammatory hyperalgesia to heat [95]. Postulating that epidermal TRPV3 and TRPV4 contribute to mastalgia, an antagonist that blocks all these three receptors may have increased efficacy in relieving breast pain. An experimental support for this hypothesis is provided by anandamide, an analgesic endocannabinoid, that activates TRPV1 directly and TRPV4 indirectly via a metabolite.
CONCLUDING REMARKS
Vanilloid TRPV1 receptor agonists like capsaicin and RTX are drugs with proven therapeutic value; their clinical utility is limited by a combination of the initial pain response that they evoke and their poor bioavailability. The current interest in developing TRPV1 ligands into clinically useful drugs is heavily biased toward antagonists. Whereas, at least in principle, TRPV1 antagonists should be devoid of the undesired side effects of agonists, the recent recognition of functional TRPV1 in brain nuclei as well as in a broad array of nonneuronal tissues predicts that long-term administration of TRPV1 antagonists might also lead to complications. For instance, it was postulated that TRPV1-expressing nerves are instrumental in the maintenance of normal blood pressure [96]. If this hypothesis holds true, prolonged use of TRPV1 antagonists may lead to hypertension or may aggravate preexistent disease. The biochemical regulation of TRPV1 is, however, complex (Figures 6.2 and 6.5). This discovery implies that TRPV1 should be studied in its specific regulatory environment that may be disease state–dependent. The ultimate goal would be the synthesis of ligands that specifically target TRPV1 in diseased (e.g., inflamed) tissues but spare TRPV1 that subserves its physiological functions in healthy tissues.
ACKNOWLEDGMENTS
This research was supported in part by the NIH, National Cancer Institute, Center for Cancer Research.
REFERENCES
- 1.
- Szallasi A, Blumberg PM. Vanilloid (capsaicin) receptors and mechanisms. Pharmacol Rev. 1999;51:159. [PubMed: 10353985]
- 2.
- Avelino A, Cruz F. Peptide immunoreactivity and ultrastructure of rat urinary bladder nerve fibers after topical desensitization by capsaicin or resiniferatoxin. Auton Neurosci. 2000;86:37. [PubMed: 11269923]
- 3.
- Szolcsányi J. Forty years in capsaicin research for sensory pharmacology and physiology. Neuropeptides. 2004;38:377. [PubMed: 15567473]
- 4.
- Szolcsányi J, Szallasi A, Szallasi Z, Joó F, Blumberg PM. Resiniferatoxin, an ultrapotent selective modulator of capsaicin-sensitive primary afferent neurons. J Pharmacol Exp Ther. 1990;255:923. [PubMed: 2243359]
- 5.
- Szallasi A, Blumberg PM. Specific binding of resiniferatoxin, an ultrapotent capsaicin analog, by dorsal root ganglion membranes. Brain Res. 1990;524:106. [PubMed: 2400923]
- 6.
- Ács G, Lee J, Marquez V, Blumberg PM. Distinct structure-activity relations for stimulation of 45Ca uptake and for high affinity binding in cultured rat dorsal root ganglion neurons and dorsal root ganglion membranes. Brain Res Mol Brain Res. 1996;35:173. [PubMed: 8717353]
- 7.
- Caterina MJ, Schumacher MA, Tominaga M, Rosen TA, Levine JD, Julius D. The capsaicin receptor: a heat-activated ion channel in the pain pathway. Nature. 1997;389:816. [PubMed: 9349813]
- 8.
- Szallasi A, Blumberg PM, Annicelli LL, Krause JE, Cortright DN. The cloned vanilloid receptor VR1 mediates both R-type binding and C-type calcium response in dorsal root ganglion neurons. Mol Pharmacol. 1999;56:581. [PubMed: 10462546]
- 9.
- Caterina M, Julius D. The vanilloid receptor: a molecular gateway to the pain pathway. Annu Rev Neurosci. 2001;24:487. [PubMed: 11283319]
- 10.
- Cortright DN, Szallasi A. Biochemical pharmacology of the vanilloid receptor TRPV1. An update. Eur J Biochem. 2004;271:1814. [PubMed: 15128291]
- 11.
- Di Marzo V, Blumberg PM, Szallasi A. Endovanilloid signaling in pain. Curr Opin Neurobiol. 2000;12:372. [PubMed: 12139983]
- 12.
- Jancsó M. Desensitization of sensory nerve endings (in Hungarian). Kísèrletes Orvostudomány (Experimental Medicine). 1949;2:15. [English translation in Ref. 1]
- 13.
- McNamara FN, Randall A, Gunthorpe MJ. Effects of piperine, the pungent component of black pepper, at the human vanilloid receptor VR1 (TRPV1). Br J Pharmacol. 2005;144:781. [PMC free article: PMC1576058] [PubMed: 15685214]
- 14.
- Voets T, Droogmans G, Wissenbach U, Janssens A, Flockerzi V, Nilius B. The principle of temperature-dependent gating in cold- and heat-sensitive TRP channels. Nature. 2002;430:748. [PubMed: 15306801]
- 15.
- Hellwig N, Plant TD, Janson W, Schafer M, Schultz G, Schaefer M. TRPV1 acts as proton channel to induce acidification in nociceptive neurons. J Biol Chem. 2004;279:34553. [PubMed: 15173182]
- 16.
- Zygmunt PM, Petersson J, Andersson DA, Chuang HH, Sørgård M, DiMarzo V, Julius D, Högestatt T. Vanilloid receptors on sensory nerves mediate the vasodilator action of anandamide. Nature. 1999;400:452. [PubMed: 10440374]
- 17.
- Tognetto M, Amadesi S, Harrison S, Creminon C, Trevisani M, Carreras M, Matera M, Geppetti P, Bianchi A. Anandamide excites central terminals of dorsal root ganglion neurons via vanilloid receptor-1. J Neurosci. 2001;21:1104. [PMC free article: PMC6762236] [PubMed: 11160380]
- 18.
- Smart D, Gunthorpe MJ, Jerman JC, Nasir S, Gray J, Muir AI, Chambers JK, Randall AD, Davis JB. The endogenous lipid anandamide is a full agonist at the human vanilloid receptor (hVR1). Br J Pharmacol. 2000;129:227. [PMC free article: PMC1571834] [PubMed: 10694225]
- 19.
- Gavva NR, Klionsky L, Qu Y, Shi L, Tamir R, Edenson S, Zhang TJ, Viswanadhan VN, Toth A, Pearce LV, Vanderah TW, Porreca F, Blumberg PM, Lile J, Sun Y, Wild K, Louis JC, Treanor JJS. Molecular determinants of vanilloid sensitivity in TRPV1. J Biol Chem. 2004;279:20283. [PubMed: 14996838]
- 20.
- Premkumar LS, Ahern GP. Induction of vanilloid receptor channel activity by protein kinase C. Nature. 2000;408:985. [PubMed: 11140687]
- 21.
- Vellani V, Mapplebeck S, Moriondo A, Davis JB, McNaughton PA. Protein kinase C activation potentiates gating of the vanilloid receptor VR1 by capsaicin, protons, heat, and anandamide. J Physiol. 2001;534:813. [PMC free article: PMC2278732] [PubMed: 11483711]
- 22.
- Olah Z, Karai I, Iadarola M. Anandamide activates vanilloid receptor (VR1) at acidic pH in dorsal root ganglia and cells ectopically expressing VR1. J Biol Chem. 2001;276:31163. [PubMed: 11333266]
- 23.
- De Petrocellis L, Bisogno T, Macarrone M, Davis JB, Finazzi-Agro A, DiMarzo V. The activity of anandamide at vanilloid VR1 receptors requires facilitated transport across the cell membrane and is limited by intracellular metabolism. J Biol Chem. 2001;276:12856. [PubMed: 11278420]
- 24.
- Ahluwalia J, Urban L, Capogna M, Bevan S, Nagy I. Cannabinoid 1 receptors are expressed on nociceptive primary sensory neurons. Neuroscience. 2000;100:685. [PubMed: 11036202]
- 25.
- DiMarzo V, Bisogno T, De Petrocellis L. Anandamide: some like it hot. Trends Pharmacol Sci. 2001;22:346. [PubMed: 11431028]
- 26.
- Nèmeth H, Helyes Z, Than M, Jakab B, Pintèr E, Szolcsányi J. Concentration-dependent dual effect of anandamide on sensory neuropeptide release from isolated rat tracheae. Neurosci Lett. 2003;336:89. [PubMed: 12499047]
- 27.
- Huang SM, Bisogno T, Trevisani M, Al-Hayani A, De Petrocellis L, Fezza F, Tognetto M, Petros TJ, Krey JF, Chu CI, Miller JD, Davies SN, Geppetti P, Walker JM, DiMarzo V. An endogenous capsaicin-like substance with high potency at recombinant and native vanilloid VR1 receptors. Proc Natl Acad Sci USA. 2002;99:8400. [PMC free article: PMC123079] [PubMed: 12060783]
- 28.
- Hwang SW, Cho J, Kwak L, Lee SY, Kang J, Jung S, Cho KH, Min YG, Suh D, Kim U, Oh U. Direct activation of capsaicin receptors by products of lipoxygenases: endogenous capsaicin-like substances. Proc Natl Acad Sci USA. 2000;97:6155. [PMC free article: PMC18574] [PubMed: 10823958]
- 29.
- Trevisani M, Smart D, Gunthorpe MJ, Tognetto M, Barbieri M, Campi B, Amadesi S, Gray J, Jerman JC, Brough SJ, Owen D, Smith GD, Randall AD, Harrison S, Bianchi A, Davis JB, Geppetti P. Ethanol elicits and potentiates nociceptor responses via the vanilloid receptor-1. Nat Neurosci. 2002;5:546. [PubMed: 11992116]
- 30.
- Chuang HH, Prescott ED, Kong H, Shields S, Jordt SE, Basbaum A, Chao MV, Julius D. Bradykinin and nerve growth factor release the capsaicin receptor from PtdIns(4,5)P2-mediated inhibition. Nature. 2001;411:957. [PubMed: 11418861]
- 31.
- Liu B, Zhang C, Qin F. Functional recovery from desensitization of vanilloid receptor TRPV1 requires resynthesis of phosphatidylinositol 4,5-bisphosphate. J Neurosci. 2005;25:4835. [PMC free article: PMC6724779] [PubMed: 15888659]
- 32.
- Puntambekar P, Van Buren J, Raisinghani M, Premkumar LS, Ramkumar V. Direct interaction of adenosine with the TRPV1 channel protein. J Neurosci. 2004;24:3663. [PMC free article: PMC6729738] [PubMed: 15071115]
- 33.
- Lakshmi S, Joshi PG. Co-activation of P2Y2 receptor and TRPV channel by ATP: implications for ATP-induced pain. Cell Mol Neurobiol. 2005;25:819. [PubMed: 16133936]
- 34.
- Jung J, Shin JS, Lee SY, Hwang SW, Koo J, Cho H, Oh U. Phospho-rylation of vanilloid receptor 1 by Ca2+/calmodulin-dependent kinase II regulates its vanilloid binding. J Biol Chem. 2004;279:7048. [PubMed: 14630912]
- 35.
- Yang C, Kazanietz MG. Divergence and complexities in DAG signaling: looking beyond PKC. Trends Pharmacol Sci. 2003;24:602. [PubMed: 14607084]
- 36.
- Szallasi A, Blumberg PM. Vanilloid receptor loss in rat sensory ganglia associated with long-term desensitization to resiniferatoxin. Neurosci Lett. 1992;136:51. [PubMed: 1407700]
- 37.
- Shu XQ, Mendell LM. Neurotrophins and hyperalgesia. Proc Natl Acad Sci USA. 1999;96:7693. [PMC free article: PMC33603] [PubMed: 10393882]
- 38.
- Davis JB, Gray J, Gunthorpe MJ, Hatcher JP, Davey PT, Harries P, Harries MH, Latcham J, Clapham C, Atkinson K, Rance SA, Grau E, Harper AJ, Pugh PL, Rogers DC, Randall S, Randall A, Sheardown SA. Vanilloid receptor-1 is essential for inflammatory thermal hyperalgesia. Nature. 2000;405:183. [PubMed: 10821274]
- 39.
- Caterina MJ, Leffler A, Malmberg AN, Martin WJ, Trafton J, Petersen-Zeitz KR, Koltzenburg M, Basbaum AI, Julius D. Impaired nociception and pain sensation in mice lacking the capsaicin receptor. Science. 2000;288:306. [PubMed: 10764638]
- 40.
- Amaya F, Shimosato G, Nagano M, Ueda M, Hashimoto S, Tanaka Y, Suzuki M, Tanaka M. NGF and GDNF differentially regulate TRPV1 expression that contributes to development of inflammatory hyperalgesia. Eur J Neurosci. 2004;20:2303. [PubMed: 15525272]
- 41.
- Zhang N, Inan S, Cowan A, Sun R, Wang JM, Rogers TJ, Caterina M, Oppenheim JJ. A proinflammatory chemokine, CCL3, sensitizes the heat- and capsaicin-gated ion channel TRPV1. Proc Natl Acad Sci USA. 2005;102:4536. [PMC free article: PMC555471] [PubMed: 15764707]
- 42.
- Tominaga M, Numazaki M, Iida T, Moriyama T, Togashi K, Higashi T, Murayama N, Tominaga T. Regulation mechanisms of vanilloid receptors. Novartis Found Symp. 2004;261:4. [PubMed: 15469041]
- 43.
- Moriyama T, Higashi T, Togashi K, Iida T, Segi E, Sugimoto Y, Tominaga T, Naruyima S, Tominaga M. Sensitization of TRPV1 by EP1 and IP reveals peripheral nociceptive mechanisms of prostaglandins. Mol Pain. 2005;1:3. [PMC free article: PMC1074353] [PubMed: 15813989]
- 44.
- Jung J, Shin JS, Lee SY, Hwang SW, Koo J, Cho H, Oh U. Phospho-rylation of vanilloid receptor 1 by Ca2+/calmodulin-dependent kinase II regulates its vanilloid binding. J Biol Chem. 2004;279:7048. [PubMed: 14630912]
- 45.
- Zhuang ZY, Xu H, Clapham DE, Ji RR. Phosphatidylinositol 3-kinase activates ERK in primary sensory neurons and mediates inflammatory hyperalgesia through TRPV1 sensitization. J Neurosci. 2004;24:8300. [PMC free article: PMC6729698] [PubMed: 15385613]
- 46.
- Jin X, Morsy N, Winston J, Pasricha PJ, Garrett K, Akbarali HI. Modulation of TRPV1 by nonreceptor kinase, c-Src kinase. Am J Physiol Cell Physiol. 2004;287:558. [PubMed: 15084474]
- 47.
- Garcia-Sanz N, Fernandez-Carjaval A, Morenilla-Palao C, Planells-Cases R, Fajardo-Sanchez E, Fernandez-Ballaster G, Ferrer-Montiel A. Identification of a tetramerization domain in the C-terminus of the vanilloid receptor. J Neurosci. 2004;24:5307. [PMC free article: PMC6729306] [PubMed: 15190102]
- 48.
- Rutter AR, Ma QP, Leveridge M, Bonnert TP. Heteromerization and colo-calization of TrpV1 and TrpV2 in mammalian cell lines and rat dorsal root ganglia. NeuroReport. 2005;16:1735. [PubMed: 16237318]
- 49.
- Goswami C, Dreger M, Jahnel R, Bogen O, Gillen C, Hucho F. Identification and characterization of a Ca2+-sensitive interaction of the vanilloid receptor TRPV1 with tubulin. J Neurochem. 2004;91:1092. [PubMed: 15569253]
- 50.
- Morenilla-Palao C, Planells-Cases R, Garcia-Sanz N, Ferrer-Montiel A. Regulated exocytosis contributes to protein kinase C potentiation of vanilloid receptor activity. J Biol Chem. 2004;279:25665. [PubMed: 15066994]
- 51.
- Amadesi S, Nie J, Vergnolle N, Cottrell GS, Grady EF, Trevisani M, Manni C, Geppetti P, McRoberts JA, Ennes H, Davis JB, Mayer EA, Bunnett NW. Protease activated receptor 2 sensitizes the capsaicin receptor transient receptor potential vanilloid receptor 1 to induce hyperalgesia. J Neurosci. 2004;24:4300. [PMC free article: PMC6729438] [PubMed: 15128844]
- 52.
- Dai Y, Moriyama T, Higashi T, Togashi K, Kobayashi K, Yamanaka H, Tominaga M, Noguchi K. Proteinase activated receptor 2-mediated potentiation of transient receptor potential vanilloid subfamily 1 activity reveals a mechanism for proteinase-induced inflammatory pain. J Neurosci. 2004;24:4293. [PMC free article: PMC6729433] [PubMed: 15128843]
- 53.
- Stander S, Moorman C, Schumacher M, Buddenkotte J, Artuc M, Shpacovitch V, Brzoska T, Lippert U, Henz BM, Luger TA, Metze D, Steinhoff M. Expression of vanilloid receptor subtype-1 in cutaneous sensory nerve fibers, mast cells, and epithelial cells of appendage structure. Exp Dermatol. 2004;13:129. [PubMed: 14987252]
- 54.
- Dattilio A, Vizzard MA. Up-regulation of protease activated receptors in bladder after cyclophosphamide-induced cystitis and colocalization with capsaicin receptor (VR1) in bladder nerve fibers. J Urol. 2005;173:635. [PubMed: 15643279]
- 55.
- Toth A, Wang Y, Kedei N, Tran R, Pearce LV, Kang SU, Jin MK, Choi HK, Lee J, Blumberg PM. Different vanilloid agonists cause different patterns of calcium responses in CHO cells heterogenously expressing rat TRPV1. Life Sci. 2005;76:2921. [PubMed: 15820503]
- 56.
- Mohapatra DP, Nau C. Regulation of Ca2+-dependent desensitization in the vanilloid receptor TRPV1 by calcineurin and cAMP-dependent protein kinase. J Biol Chem. 2005;280:13424. [PubMed: 15691846]
- 57.
- Mandadi S, Numazaki M, Tominaga M, Bhat MB, Armati PJ, Roufogalis BD. Activation of protein kinase C reverses capsaicin-induced calcium-dependent desensitization of TRPV1 ion channels. Cell Calcium. 2004;35:471. [PubMed: 15003856]
- 58.
- Chou MZ, Mtui T, Gao YD, Kohler M, Middleton RE. Resiniferatoxin binds to the capsaicin receptor (TRPV1) near the extracellular side of the S4 trans-membrane domain. Biochem. 2004;43:2501. [PubMed: 14992587]
- 59.
- Appendino G, Szallasi A. Clinically useful vanilloid receptor TRPV1 antagonists: just around the corner (or too early to tell). Progress Med Chem. 2006;44:145. [PubMed: 16697897]
- 60.
- Szallasi A, DiMarzo V. New perspectives on enigmatic vanilloid receptors. Trends Neurosci. 2000;23:491. [PubMed: 11006466]
- 61.
- Southall MD, Li T, Gharibova LS, Pei Y, Nicol GD, Travers JB. Activation of epidermal vanilloid receptor-1 induced release of proinflammatory mediators in human keratinocytes. J Pharmacol Exp Ther. 2003;304:217. [PubMed: 12490594]
- 62.
- Lazzeri M, Vannucchi MG, Zardo C, Spinelli M, Beneforti P, Turini D, Faussone-Pellegrini MS. Immunohistochemical evidence of vanilloid receptor 1 in normal human urinary bladder. Eur Urol. 2004;46:792. [PubMed: 15548449]
- 63.
- Golech SA, McCarron RM, Chen Y, Bembry J, Lenz F, Mechoulam R, Shohami E, Spatz M. Human brain endothelium: coexpression and function of vanilloid and endocannabinoid receptors. Brain Res Mol Brain Res. 2004;132:87. [PubMed: 15548432]
- 64.
- Kim JC, Beckel JM, Birder LA, Kiss S, Washabaugh C, Kanai A, Reynolds I, Dineley K, de Groat WC, Caterina MJ. Identification of functional vanilloid receptors in human bladder urothelial cells using a nitric oxide microsensor technique and reverse transcriptase polymerase chain reaction. J Urol. 2001;165:34.
- 65.
- Dvorakova M, Kummer W. Transient expression of vanilloid receptor subtype 1 in rat cardiomyocytes during development. Histochem Cell Biol. 2001;116:223. [PubMed: 11685550]
- 66.
- Sanchez JF, Krause JE, Cortright DN. The distribution and regulation of vanilloid receptor VR1 and VR1 5’ splice variant RNA expression in rat. Neurosci. 2001;107:373. [PubMed: 11718993]
- 67.
- Reilly CA, Taylor JL, Lanza DL, Carr BA, Crouch DJ, Yost GS. Capsaicinoids cause inflammation and epithelial cell death through activation of vanilloid receptors. Toxicol Sci. 2003;73:170. [PMC free article: PMC2423488] [PubMed: 12721390]
- 68.
- Tian W, Fu Y, Wang DH, Cohen DM. Regulation of TRPV1 by a novel renally expressed rat TRPV1 splice variant. Am J Physiol Renal Physiol. 2005;290:F117. [PubMed: 16091583]
- 69.
- Schumacher MA, Moff I, Sudanagunta SP, Levine JD. Molecular cloning of an N-terminal domain suggests functional divergence among capsaicin receptor subtypes. J Biol Chem. 2000;275:2756. [PubMed: 10644739]
- 70.
- Wang C, Hu HZ, Colton CK, Wood JD, Zhu MX. An alternative splicing product of the murine trpv1 gene dominant negatively modulates the activity of TRPV1 channels. J Biol Chem. 2004;279:37423. [PubMed: 15234965]
- 71.
- Chen SC, Chang TJ, Wu FS. Competitive inhibition of the capsaicin receptor-mediated current by dehydroepiandrosterone in rat dorsal root ganglion neurons. J Pharmacol Exp Ther. 2004;311:529. [PubMed: 15201344]
- 72.
- Chen SC, Wu FS. Mechanisms underlying inhibition of the capsaicin receptor-mediated current by pregnenolone sulfate in rat dorsal root ganglion neurons. Brain Res. 2004;1027:196. [PubMed: 15494172]
- 73.
- Peroni RN, Orliac ML, Becu-Villalobos D, Huidobro-Toro JP, Adler-Graschin-sky E, Celuch SM. Sex-linked differences in the vasorelaxant effects of anan-damide in vascular mesenteric beds: role of oestrogens. Eur J Pharmacol. 2004;493:151. [PubMed: 15189776]
- 74.
- Frot M, Feine JS, Bushnell MC. Sex differences in pain perception and anxiety. A psychophysical study with topical capsaicin. Pain. 2004;108:230. [PubMed: 15030942]
- 75.
- Akiba Y, Kato S, Katsube K, Nakamura M, Takeuchi K, Ishii H, Hibi T. Transient receptor potential vanilloid subfamily 1 expressed in pancreatic islet beta cells modulates insulin secretion in rats. Biochem Biophys Res Comm. 2004;321:219. [PubMed: 15358238]
- 76.
- Bölcskei K, Helyes Zs, Szabó Á, Sándor K, Elekes K, Nèmeth J, Almási R, Pintèr E, Pethö G, Szolcsányi J. Investigation of the role of TRPV1 receptors in acute and chronic nociceptive processes using gene-deficient mice. Pain. 2005;117:368. [PubMed: 16150543]
- 77.
- Baamonde A, Lastra A, Juarez L, Hidalgo A, Menendez L. TRPV1 desensitization and endogenous vanilloid involvement in the enhanced analgesia induced by capsaicin in inflamed tissues. Brain Res Bull. 2005;67:476. [PubMed: 16216696]
- 78.
- Peier AM, Moqrich A, Hergarden AC, Reeve AJ, Andersson DA, Story GM, Earley TJ, Dragoni I, McIntyre P, Bevan S, Patapoutian A. A TRP channel that senses cold stimuli and menthol. Cell. 2002;108:705. [PubMed: 11893340]
- 79.
- Bandell M, Story GM, Hwang SW, Viswanath V, Eid SR, Petrus MJ, Earley TJ, Patapoutian A. Noxious cold ion channel TRPA1 is activated by pungent compounds and bradykinin. Neuron. 2004;41:849. [PubMed: 15046718]
- 80.
- Weil A, Moore SE, Waithe NJ, Randall A, Gunthorpe MJ. Conservation of functional and pharmacological properties in the distantly related temperature sensors TRPV1 and TRPM8. Mol Pharmacol. 2005;68:518. [PubMed: 15911692]
- 81.
- Sanchez MG, Sanchez AM, Collado B, Malagarie-Cazenave S, Olea N, Car-mena MJ, Prieto JC, Diaz-Laviada II. Expression of the transient receptor potential vanilloid 1 (TRPV1) in LNCaP and PC-3 prostate cancer cells in human prostate tissue. Eur J Pharmacol. 2005;515:20. [PubMed: 15913603]
- 82.
- Zhang L, Barritt GJ. Evidence that TRPM8 is an androgen-dependent Ca2+ channel required for the survival of prostate cancer cells. Cancer Res. 2004;64:8365. [PubMed: 15548706]
- 83.
- Surh YJ. More than spice: capsaicin in hot chili peppers makes tumor cells commit suicide. J Natl Cancer Inst. 2002;94:1263. [PubMed: 12208886]
- 84.
- Gopinath P, Wan E, Holdcroft A, Facer P, Davis JB, Smith GD, Bountra C, Anand P. Increased capsaicin receptor TRPV1 in skin nerve fibers and related vanilloid receptors TRPV3 and TRPV4 in keratinocytes in human breast pain. BMC Women’s Health. 2005;5:2. [PMC free article: PMC554997] [PubMed: 15755319]
- 85.
- Tympanidis P, Casula MA, Yiangou Y, Terenghi G, Dowd P, Anand P. Increased vanilloid receptor VR1 innervation of vulvodynia. Eur J Pain. 2004;8:129. [PubMed: 14987622]
- 86.
- Matthews PJ, Aziz Q, Facer P, Davis JB, Thompson DG, Anand P. Increased capsaicin receptor TRPV1 nerve fibers in the inflamed human oesophagus. Eur J Gastroenterol Hepatol. 2004;16:897. [PubMed: 15316415]
- 87.
- Yiangou Y, Facer P, Dyer NH, Fowler CJ, Anand P. Vanilloid receptor 1 immunoreactivity in inflamed human bowel. Lancet. 2001;357:1338. [PubMed: 11343743]
- 88.
- Szallasi A. Vanilloid (capsaicin) receptors in health and disease. Am J Clin Pathol. 2002;118:110. [PubMed: 12109845]
- 89.
- Donnerer J, Liebmann I, Schicho R. Differential regulation of 3-beta-hydrox-ysteroid dehydrogenase and vanilloid receptor TRPV1 mRNA in sensory neurons by capsaicin and NGF. Pharmacol. 2005;73:97. [PubMed: 15492487]
- 90.
- Carlton SM, Coggeshall RE. Peripheral capsaicin receptors increase in the inflamed rat hindpaw: a possible mechanism for peripheral sensitization. Neurosci Lett. 2001;310:53. [PubMed: 11524156]
- 91.
- Contassot E, Tenan M, Schnuriger V, Pelte MF, Dietrich PY. Arachidonyl ethanolamine induces apoptosis of uterine cervix cancer cells via aberrantly expressed vanilloid receptor-1. Gynecol Oncol. 2004;93:182. [PubMed: 15047233]
- 92.
- Johansen ME, Reilly CA, Yost GS. TRPV1 antagonists elevate cell surface populations of receptor protein and exacerbate TRPV1-mediated toxicities in human lung epithelial cells. Tox Sci. 2006;89:278. [PMC free article: PMC2291391] [PubMed: 16120755]
- 93.
- Doherty MJ, Mister R, Pearson MG, Calverley PMC. Capsaicin responsiveness and cough in asthma and chronic obstructive pulmonary disease. Thorax. 2000;55:643. [PMC free article: PMC1745828] [PubMed: 10899239]
- 94.
- Mitchell JE, Campbell AP, New NE, Sadofsky LR, Kastelik JA, Mulrennan SA, Compton SJ, Morice AH. Expression and characterization of the intra-cellular vanilloid receptor (TRPV1) in bronchi from patients with chronic cough. Exp Lung Res. 2005;31:295. [PubMed: 15962710]
- 95.
- Moqrich A, Hwang SW, Earley TJ, Petrus MJ, Murray AN, Spencer KS, Andahazy M, Stoey GM, Patapoutian A. Impaired thermosensation in mice lacking TRPV3, a heat and camphor sensor in the skin. Science. 2005;307:1468. [PubMed: 15746429]
- 96.
- Vaishnava P, Wang DH. Capsaicin sensitive sensory nerves and blood pressure regulation. Curr Med Chem Cardiovasc Hematol Agents. 2003;1:177. [PubMed: 15320697]
- INTRODUCTION
- THE VANILLOID RECEPTOR TRPV1 AND ITS ENDOGENOUS LIGANDS, THE “ENDOVANILLOIDS”
- TRPV1 IN THE RESTING STATE
- MOLECULAR ACTIVATORS OF TRPV1
- REGULATION BY NGF OF TRPV1 MAY PLAY A CENTRAL ROLE IN INFLAMMATORY HYPERALGESIA
- MODULATION OF TRPV1 BY PROTEIN KINASES
- SHUFFLING OF TRPV1 AMONG VARIOUS SUBCELLULAR COMPARTMENTS
- REGULATION OF TRPV1 BY MAST CELLS
- HOW CAN A SINGLE RECEPTOR MEDIATE RESPONSES WITH DISSIMILAR STRUCTURE-ACTIVITY RELATIONS?
- DIVERSITY OF BEHAVIOR OF EXOGENOUS LIGANDS
- TISSUE AND CELLULAR EXPRESSION OF VR1 AND ITS SPLICE VARIANTS
- POSSIBLE REGULATION OF TRPV1 BY SPLICE VARIANTS
- REGULATION OF TRPV1 BY SERUM STEROIDS
- REGULATION OF TRPV1 BY (NEURO)ENDOCRINE FACTORS
- TRPV1 MAY SUBSERVE OPPOSING ACTIONS AT THE WHOLE ANIMAL LEVEL
- TRPV1 IS COEXPRESSED WITH ITS RELATIVES ON SENSORY NEURONS WITH OVERLAPPING ACTIVITY
- PHARMACOLOGICAL OVERLAP BETWEEN TRPV1 AND ITS RELATIVES
- TRPV1 EXPRESSION MAY CHANGE UNDER PATHOLOGICAL CONDITIONS
- CONCLUDING REMARKS
- ACKNOWLEDGMENTS
- REFERENCES
- Review Resiniferatoxin and its analogs provide novel insights into the pharmacology of the vanilloid (capsaicin) receptor.[Life Sci. 1990]Review Resiniferatoxin and its analogs provide novel insights into the pharmacology of the vanilloid (capsaicin) receptor.Szallasi A, Blumberg PM. Life Sci. 1990; 47(16):1399-408.
- Autoradiographic visualization and pharmacological characterization of vanilloid (capsaicin) receptors in several species, including man.[Acta Physiol Scand Suppl. 1995]Autoradiographic visualization and pharmacological characterization of vanilloid (capsaicin) receptors in several species, including man.Szallasi A. Acta Physiol Scand Suppl. 1995; 629:1-68.
- Review The vanilloid (capsaicin) receptor: receptor types and species differences.[Gen Pharmacol. 1994]Review The vanilloid (capsaicin) receptor: receptor types and species differences.Szallasi A. Gen Pharmacol. 1994 Mar; 25(2):223-43.
- Antinociceptive desensitizing actions of TRPV1 receptor agonists capsaicin, resiniferatoxin and N-oleoyldopamine as measured by determination of the noxious heat and cold thresholds in the rat.[Eur J Pain. 2010]Antinociceptive desensitizing actions of TRPV1 receptor agonists capsaicin, resiniferatoxin and N-oleoyldopamine as measured by determination of the noxious heat and cold thresholds in the rat.Bölcskei K, Tékus V, Dézsi L, Szolcsányi J, Petho G. Eur J Pain. 2010 May; 14(5):480-6. Epub 2009 Oct 1.
- Review Vanilloid receptors: new insights enhance potential as a therapeutic target.[Pain. 1996]Review Vanilloid receptors: new insights enhance potential as a therapeutic target.Szallasi A, Blumberg PM. Pain. 1996 Dec; 68(2-3):195-208.
- Complex Regulation of TRPV1 by Vanilloids - TRP Ion Channel Function in Sensory ...Complex Regulation of TRPV1 by Vanilloids - TRP Ion Channel Function in Sensory Transduction and Cellular Signaling Cascades
- TRNAD-GUC [Hibiscus syriacus]TRNAD-GUC [Hibiscus syriacus]Gene ID:120198676Gene
Your browsing activity is empty.
Activity recording is turned off.
See more...