Some rights reserved. This work is available under the Creative Commons Attribution-NonCommercial-NoDerivs 3.0 IGO licence (CC BY-NC-ND 3.0 IGO; https://creativecommons.org/licenses/by-nc-nd/3.0/igo/). Under the terms of this licence, you may copy and redistribute the work for non-commercial purposes, provided the work is appropriately cited, as indicated below. In any use of this work, there should be no suggestion that WHO endorses any specific organization, products or services. The use of the WHO logo is not permitted.
NCBI Bookshelf. A service of the National Library of Medicine, National Institutes of Health.
Wild CP, Weiderpass E, Stewart BW, editors. World Cancer Report: Cancer research for cancer prevention. Lyon (FR): International Agency for Research on Cancer; 2020.
Summary
- •
The analysis of tumour-derived products, including circulating cell-free tumour DNA (ctDNA) and related biomarkers, in body fluids is increasingly recognized as an aid in the early diagnosis of malignant disease.
- •
For application in screening or early diagnosis, ctDNA analysis and related techniques require well-validated tests with exceptionally high sensitivity and specificity.
- •
Recent approaches have combined the evaluation of soluble tumour biomarkers with ctDNA analysis of cancer-related mutations in multiple genes.
- •
These technologies face challenges, including low concentrations of ctDNA and other liquid biomarker analytes.
- •
Progress in technology (e.g. next-generation sequencing) is paving the way for the development of diagnostic tests for early detection of cancer and the introduction of precision medicine into clinical practice.
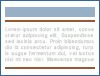
Box
Fundamentals.
The analysis of tumour cells and tumour-derived products detectable in blood and other body fluids, which was introduced by Pantel and Alix-Panabieres and has been referred to as a liquid biopsy [1], has garnered substantial interest in recent years (see Chapter 5.12). The family of liquid biopsy analytes includes circulating tumour cells (CTCs), circulating cell-free tumour DNA (ctDNA), circulating non-coding nucleic acids such as microRNAs (miRNAs) and long non-coding RNAs (lncRNAs), extracellular vesicles, and tumour-educated platelets [2–6].
Over the past 10 years, many liquid biopsy tests have been established and validated [3]. Clinical applications of liquid biopsy in patients with early-stage cancer include early detection of small tumours, improved risk assessment (tumour staging), and monitoring of minimal residual disease [7]. Thus, liquid biopsy is set to become an essential element of personalized medicine (Fig. 6.7.1).
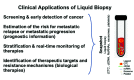
Fig. 6.7.1
Liquid biopsy in cancer. Schematic representation of the liquid biopsy concept as the analysis of circulating tumour cells (CTCs), circulating cell-free tumour DNA (ctDNA), non-coding RNAs (ncRNAs), exosomes, and tumour-educated platelets in the blood (more...)
This chapter discusses some of the recent highlights on the use of ctDNA and CTCs for early detection and monitoring of cancer.
ctDNA for early detection of cancer
Early detection of cancer in the context of a screening programme for healthy individuals at high risk is a much sought-after goal in cancer research. Current therapeutic strategies, in particular surgery, enable many patients with cancer to be cured, provided the disease is detected early in its anticipated clinical course. However, metastatic disease remains largely incurable, with very few exceptions, which specifically include testicular cancer or small liver metastases in colon cancer.
Liquid biopsy, as a minimally invasive and easily repeatable method, seems to offer an attractive alternative to invasive tissue biopsies as the current definitive methodology in tumour diagnostics. However, programmes for early detection or screening require well-validated tests with exceptionally high sensitivity and specificity.
In the context of the TRACERx study, Abbosh et al. investigated the potential of ctDNA analysis for early diagnosis and monitoring in patients with non-small cell lung carcinoma (NSCLC). The sequencing of single-nucleotide variants in resected tumour tissue was used to create a patient-specific panel for next-generation sequencing-based ctDNA analysis of plasma collected before surgery. With the detection threshold of at least two tumour-specific single-nucleotide variants, the sensitivity of personalized tests in pre-surgery plasma samples was 97% for lung squamous cell carcinomas but only 19% for lung adenocarcinomas [8]. The authors calculated that a tumour with a diameter of about 2.7 cm (volume, 10 cm3) would result in a mean ctDNA plasma variant allele frequency of 0.1%. Modern low-dose computed tomography lung screening enables the detection of tumours of diameter 0.4 cm (volume, 0.34 cm3), which would correspond to a plasma variant allele frequency of 1.8 × 10−4%, below the detection limit of most current ctDNA technologies [8].
Another aspect is the cost of the patient-tailored next-generation sequencing-based ctDNA approach for the detection of single-nucleotide variants. Abbosh at al. estimated the current cost of targeted ctDNA profiling to be US$ 1750 per patient, which is likely to be too high for routine implementation as a test for population cancer screening [8]. These findings challenge the application of ctDNA analysis for early diagnosis of small cancerous lesions.
The proper choice of markers is also very important. Markers detected and validated in patients with advanced disease, such as CEA, lack specificity and sensitivity for early detection. Concentrations of the marker are lower at early stages of disease than at late stages. In addition, the biology of these two disease states varies; therefore, a late-stage marker may not be suitable to detect small tumours at early stages. Also, blood markers of early lesions may be masked by comorbidities, such as chronic inflammatory diseases [9], as well as by the accumulation of cancer-related mutations with age in healthy individuals [10,11].
These limitations may be illustrated by the recently published work of Cohen et al., who introduced the CancerSeek panel for the detection of the eight most common cancer types [12]. This complex approach combined the evaluation of eight soluble tumour biomarkers, including standard tumour markers such as CEA, with ctDNA analysis of cancer-related mutations in 16 genes. The panel reached an overall median sensitivity of 70%, with specificity of 99% or more, but significant differences in sensitivity were observed among the tumour types analysed, including 98% in ovarian cancer, 60% in lung cancer, and 33% in breast cancer [12]. These findings require validation, ideally in an independent prospectively sampled, pre-diagnostic cohort. Moreover, the study analysed only healthy controls; therefore, the high specificity of the CancerSeek approach requires further validation with non-cancer controls with comorbidities such as inflammatory diseases, which are common in ageing individuals.
ctDNA for monitoring of minimal residual disease in patients with early-stage cancer
Liquid biopsy tests for the detection and monitoring of minimal residual disease in patients with early-stage cancer face similar challenges to tests for early detection, including low concentrations of ctDNA and other liquid biomarker analytes [7].
Tie et al. evaluated the ability of ctDNA analysis to detect minimal residual disease in blood samples obtained from patients with stage II colon cancer after surgical removal of the primary tumour. The method was able to predict recurrence at 36 months with a sensitivity of 48% and a specificity of 100% [13]. In the above-mentioned study of Abbosh et al. in patients with lung cancer, detection of ctDNA mutations that were also present in the respective primary tumour was predictive of relapse in 93% of cases, with a median of 70 days before radiological confirmation [8]. Both of these studies demonstrate the feasibility and potential clinical value of ctDNA analysis for monitoring of minimal residual disease. However, ctDNA detection required knowledge of primary tumour-specific mutations, and the mutational spectrum may change during progression from minimal residual disease to overt metastatic disease.
ctDNA analysis without prior knowledge of the genetics of the primary tumour was applied in a recent study of patients with stage I–III lung cancer. Chaudhuri et al. used the highly sensitive cancer personalized profiling by deep sequencing (CAPP-Seq) approach targeting 128 genes that are recurrently mutated in lung cancer. Detection of ctDNA after the initial treatment of the primary tumour was predictive of progression in 72% of patients, with a median of 5.2 months before radiological evidence of disease recurrence. Remarkably, ctDNA was detectable in 94% of patients experiencing recurrence at the “minimal residual disease landmark” time point, which was defined as the first post-treatment blood draw within 4 months of treatment completion [14].
Goh et al. used in vitro and patient-derived xenograft assays to test whether chromosome 1q23.1 amplification was enriched in tumour-initiating cells from patients with breast cancer. Amplification of the region was detected in ctDNA as the average copy-number ratio of three genes (TUFT1, S100A7, and S100A8) relative to a reference gene by droplet digital polymerase chain reaction (PCR). Detection of the amplification in ctDNA samples already at first diagnosis was predictive of relapse within 5 years in 67% of patients with early-stage breast cancer (stage I or II) and within 3 years in 40% of patients with locally advanced breast cancer (stage II or III), with 100% specificity in both cohorts [15].
Taken together, these results demonstrate the power of ctDNA analysis to predict minimal residual disease in patients with cancer.
CTCs for early detection and monitoring of minimal residual disease
Over the past decade, in addition to the measurement of ctDNA, various methods have been developed to detect CTCs in the peripheral blood of patients with cancer [16]. As for any other liquid biopsy analyte, quantification and characterization of CTCs in the blood of patients with cancer at any particular time provides a snapshot of the actual disease status. It has been shown that regular enumeration of CTCs can be used for disease prognosis, diagnosis of minimal residual disease, and monitoring of effectiveness of therapy [17–19].
Although reliable information can easily be obtained in patients with advanced disease, patients with early-stage cancer usually present with very low concentrations of CTCs. Nonetheless, a pooled analysis including data from 3173 patients with non-metastatic breast cancer (stage I–III) provided strong evidence for CTCs as an independent prognostic factor with regard to poor overall, breast cancer-specific, and disease-free survival [20]. Detection of CTCs in patients with breast cancer receiving neoadjuvant therapy is a significant predictor of outcome independent of the response of the primary tumour to therapy [21,22]. This suggests that the presence of CTCs signals the occurrence of clinically relevant minimal residual disease at distant sites.
Currently, most CTC assays rely on epithelial markers such as EpCAM, and most of the CTCs detected are single isolated cells. Despite the relevance of epithelial–mesenchymal transition to cancer, the presence of these “epithelial” CTCs is associated with an unfavourable prognosis in cancer of the breast, prostate, colon, and lung [23]. The clinical relevance of “mesenchymal” CTCs lacking any epithelial markers as well as of CTC clusters is still under investigation, but the additional detection of these subsets of CTCs may improve the early detection of cancer and minimal residual disease. The sensitivity of current CTC assays seems to be too low to enable them to be used for early cancer detection. Only one report has shown that detection of CTCs in the blood of patients with chronic obstructive pulmonary disease was able to predict the occurrence of lung cancer [24].
It has been shown that the presence of CTCs after completion of adjuvant therapy is a predictor of metastatic relapse and poor survival [18]. Moreover, information provided by CTCs may extend to the proteomic, transcriptomic, and genomic levels. Although single-cell analysis is challenging, investigations of protein expression and genome-wide studies on single cells are becoming the state of the art [25,26]. Molecular characterization of CTCs provides a powerful tool to assess intrapatient heterogeneity and to obtain information about the clonal origin of CTCs and clonal selection under therapy. The identification of clones that are sensitive and resistant to therapy may provide new insights and potential targets for cancer treatment.
Liquid biopsy beyond ctDNA and CTC analyses
In addition, the analysis of circulating non-coding nucleic acids such as miRNAs and lncRNAs (see Chapter 3.8) is a highly promising liquid biopsy approach [4]. miRNAs and lncRNAs were found to provide additional levels of transcriptional and translational regulation and to be strongly involved in cancer development.
Although levels of upregulation and downregulation of individual miRNAs or lncRNAs are probably insufficient for a reliable test to detect cancer, signatures of 3–6 non-coding RNAs may be powerful and sensitive tools for early detection of cancer (reviewed in [4]). For example, a signature of serum miR-21 and miR-155 was reported as a sensitive and specific biomarker for diagnosis of breast cancer; for miR-21 the receiver operating characteristic (ROC) area under the curve (AUC) value was 0.788, the sensitivity was 66.67%, and the specificity was 88.89%, and for miR-155 the ROC AUC value was 0.749, the sensitivity was 100%, and the specificity was 51.02% [27].
lncRNAs can also be successfully used in diagnostic tests. Tang et al. found that three lncRNAs (LINC01627, LINC01628, and ERICH1-AS1) were upregulated in the plasma of patients with NSCLC compared with healthy individuals. The suggested diagnostic signature could identify NSCLC with high accuracy (AUC, 0.942) [28].
Recently, tumour-educated platelets have emerged as new members of the family of liquid biopsy analytes. External stimuli, such as activation of platelet surface receptors and lipopolysaccharide-mediated platelet activation, induce specific splicing of precursor messenger RNAs (mRNAs) in circulating tumour-educated platelets. The combination of specific splice events in response to external signals and the capacity of platelets to directly ingest (spliced) circulating mRNA can provide tumour-educated platelets with a highly dynamic mRNA repertoire, with potential applicability to cancer diagnostics [6,29].
The first results on the use of tumour-derived exosomes and other extracellular vesicles [30] are promising, and their potential as cancer biomarkers has been explored in multiple studies. However, the lack of standardization of protocols for pre-analytical handling and analytical workflows limits interstudy comparisons and large international multicentre studies [31]. Moreover, the investigation of extracellular vesicles and their content in combination with other liquid biopsy analytes (e.g. CTCs, ctDNA) may provide new opportunities for the development of diagnostic tests [32].
In addition to ctDNA and CTCs, the biomarkers discussed in this chapter provide information not only on tumour cells but also on the tumour microenvironment – such as stromal and immune cells. This additional information may be helpful to detect the body’s response to the development of small cancerous lesions, which in turn could be used for early cancer detection.
Technologies for detection of ctDNA and CTCs
Circulating cell-free DNA (cfDNA) in blood plasma is highly fragmented DNA derived mainly from apoptotic cells. The concentration of ctDNA in blood may be less than 0.01% of the total cfDNA concentration, in particular during the early stages of cancer that are relevant to early detection programmes.
Researchers have used various targeted DNA sequencing techniques, such as digital PCR (quantitative PCR), BEAMing (beads, emulsion, amplification, magnetics) technology, the safe-sequencing system, CAPP-Seq, and tagged-amplicon deep sequencing [33]. These methods can reach ctDNA detection limits of less than 0.01%. A disadvantage of these technologies is the requirement for detailed prior information on the mutational spectrum of the tumour in the individual patient. This may be a limitation if these techniques are used for cancer screening. Such information is not required if non-targeted next-generation sequencing is applied to investigate ctDNA, enabling the genome-wide analysis of mutations by whole-genome sequencing or whole-exome sequencing. However, the drawbacks of genome-wide ctDNA analyses compared with targeted approaches include the need for higher concentrations of ctDNA and the lower overall assay sensitivity.
In addition to next-generation sequencing-based mutation analysis (see Chapter 3.2), which is the most prominent approach in ctDNA analysis, copy number alteration (CNA) and methylation analyses are garnering substantial interest [34]. Shallow whole-genome sequencing of ctDNA, which enables the cost-effective global assessment of CNAs [35], has introduced the global CNA score as a reliable biomarker associated with active disease and survival in patients with melanoma. Similarly, genome-wide CNA assessment has been used to screen cfDNA for the detection of incipient haematological malignancies in apparently healthy individuals [36].
Epigenomic tumour-specific alterations can be detected in ctDNA and have the potential to serve as biomarkers. Shen et al. demonstrated the ability to identify large-scale tumour-specific ctDNA methylation patterns [37]. The method they established was successfully applied for cancer detection and classification in a patient cohort across several tumour types [37]. Besides large-scale methylation assessment, smaller panels have the benefit of being less expensive and easier to interpret. Thus, methylation of 12 genes investigated by droplet digital methylation-specific PCR in ctDNA was successfully applied to accurately distinguish between patients with breast cancer and healthy volunteers [38].
Furthermore, the physicochemical properties of methylated DNA assessed as the methylation landscape of cfDNA could be used to accurately discriminate between healthy individuals and patients with cancer (accuracy > 70%) [39]. These recent findings demonstrate the high potential as biomarkers of cfDNA CNA and methylome analyses. However, these findings require further validation in larger cohorts and groups of patients with early-stage cancer or benign disease.
Efficient enrichment of CTCs can be achieved by approaches that exploit the differences between tumour cells and blood cells, including the differential expression of cell membrane proteins (e.g. EpCAM, the most widely used marker for the enrichment of CTCs in blood from patients with carcinoma) as well as different sizes, densities, electric charges, and deformabilities [5,16]. After enrichment, the CTCs are still surrounded by hundreds to thousands of leukocytes, and therefore reliable methods are required to identify a CTC at the single-cell level.
CTCs can be detected by antibodies against membrane and cytoplasmic antigens, including epithelial, mesenchymal, tissue-specific, and tumour-associated markers. Most current CTC assays use the same identification step as the system approved by the United States Food and Drug Administration (FDA) for detecting CTCs in patients with metastatic cancer: cells are fluorescently stained for epithelial keratins as a marker of CTCs, and CD45 is used as a leukocyte exclusion marker. Although some antigens are applicable to various different cancer types (e.g. keratins are suitable for cancers of the breast, colon, and prostate and other epithelial tumours), tissue-specific antigens such as prostate-specific antigen or breast-specific mammaglobin are also suitable.
From discovery to clinical validation and utility
Currently, only two liquid biopsy tests are approved in the USA by the FDA, but not for the early detection of cancer. The FDA approved the above-mentioned system for detecting CTCs in metastatic cancer in 2018 and an EGFR mutation test for ctDNA analysis in 2016 [3]. The EGFR mutation test can detect EGFR gene mutations in patients with NSCLC. Such mutations are present in about 10–20% of patients with NSCLC. The EGFR mutation test identifies the presence of 42 specific NSCLC mutations in exons 18–21, including the L858R mutation, exon 19 deletions, and the T790M mutation. On the basis of these data, patients who may benefit from treatment with erlotinib or osimertinib may be selected. However, if such mutations are not detected in the blood, then a tumour biopsy should be performed to determine whether the NSCLC mutations are present. Insofar as the test provides positive results, it may benefit patients who may be too ill or are otherwise unable to provide a tumour specimen for EGFR testing.
Blood is a rich source of information through which solid cancers can be detected, classified, and matched to a specific therapy. Different approaches such as ctDNA, non-coding nucleic acids, extracellular vesicles, tumour-educated platelets, or CTC analyses will provide complementary information, depending on the tumour type and the intended clinical use. Despite the potential of individual techniques, each has its own limitations; this leads to the idea of combining different analytes for the early detection of cancer. Technical and clinical validation of assays is very important and can be achieved in independent, international consortia such as the European IMI Cancer-ID network (https://www.cancer-id.eu). Similar to the development of new drugs, the pipeline for the development of new diagnostic tools needs more standardization to bridge the gap between the plethora of published biomarker studies and the paucity of new markers entering clinical practice.
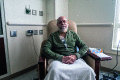
Fig. 6.7.2
A patient receiving chemotherapy in the context of cancer management. The currently available data suggest improved clinical management based on the power of circulating cell-free tumour DNA (ctDNA) analysis to detect and monitor minimal residual disease (more...)
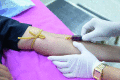
Fig. 6.7.3
A women having blood drawn. Liquid biopsy is recognized as a means of indicating prognosis for patients with cancer, but its potential is also being explored for the purpose of early diagnosis.
References
- 1.
- Pantel K, Alix-Panabières C (2010). Circulating tumour cells in cancer patients: challenges and perspectives. Trends Mol Med. 16(9):398–406. 10.1016/j.molmed.2010.07.001 [PubMed: 20667783] [CrossRef]
- 2.
- Babayan A, Pantel K (2018). Advances in liquid biopsy approaches for early detection and monitoring of cancer. Genome Med. 10(1):21. 10.1186/s13073-018-0533-6 [PMC free article: PMC5861602] [PubMed: 29558971] [CrossRef]
- 3.
- Kwapisz D (2017). The first liquid biopsy test approved. Is it a new era of mutation testing for non-small cell lung cancer? Ann Transl Med. 5(3):46. 10.21037/atm.2017.01.32 [PMC free article: PMC5326656] [PubMed: 28251125] [CrossRef]
- 4.
- Anfossi S, Babayan A, Pantel K, Calin GA (2018). Clinical utility of circulating non-coding RNAs - an update. Nat Rev Clin Oncol. 15(9):541–63. 10.1038/s41571-018-0035-x [PubMed: 29784926] [CrossRef]
- 5.
- Poudineh M, Sargent EH, Pantel K, Kelley SO (2018). Profiling circulating tumour cells and other biomarkers of invasive cancers. Nat Biomed Eng. 2(2):72–84. 10.1038/s41551-018-0190-5 [PubMed: 31015625] [CrossRef]
- 6.
- Best MG, Sol N, In ’t Veld SGJG, Vancura A, Muller M, Niemeijer AN, et al. (2017). Swarm intelligence-enhanced detection of non-small-cell lung cancer using tumor-educated platelets. Cancer Cell. 32(2):238–252.e9. 10.1016/j.ccell.2017.07.004 [PMC free article: PMC6381325] [PubMed: 28810146] [CrossRef]
- 7.
- Bardelli A, Pantel K (2017). Liquid biopsies, what we do not know (yet). Cancer Cell. 31(2):172–9. 10.1016/j.ccell.2017.01.002 [PubMed: 28196593] [CrossRef]
- 8.
- Abbosh C, Birkbak NJ, Wilson GA, Jamal-Hanjani M, Constantin T, Salari R, et al.; TRACERx consortium; PEACE consortium (2017). Phylogenetic ctDNA analysis depicts early-stage lung cancer evolution. Nature. 545(7655):446–51. 10.1038/nature22364 [PMC free article: PMC5812436] [PubMed: 28445469] [CrossRef]
- 9.
- Pantel K, Denève E, Nocca D, Coffy A, Vendrell JP, Maudelonde T, et al. (2012). Circulating epithelial cells in patients with benign colon diseases. Clin Chem. 58(5):936–40. 10.1373/clinchem.2011.175570 [PubMed: 22205690] [CrossRef]
- 10.
- Krimmel JD, Schmitt MW, Harrell MI, Agnew KJ, Kennedy SR, Emond MJ, et al. (2016). Ultra-deep sequencing detects ovarian cancer cells in peritoneal fluid and reveals somatic TP53 mutations in noncancerous tissues. Proc Natl Acad Sci U S A. 113(21):6005–10. 10.1073/pnas.1601311113 [PMC free article: PMC4889384] [PubMed: 27152024] [CrossRef]
- 11.
- Fernandez-Cuesta L, Perdomo S, Avogbe PH, Leblay N, Delhomme TM, Gaborieau V, et al. (2016). Identification of circulating tumor DNA for the early detection of small-cell lung cancer. EBioMedicine. 10:117–23. 10.1016/j.ebiom.2016.06.032 [PMC free article: PMC5036515] [PubMed: 27377626] [CrossRef]
- 12.
- Cohen JD, Li L, Wang Y, Thoburn C, Afsari B, Danilova L, et al. (2018). Detection and localization of surgically resectable cancers with a multi-analyte blood test. Science. 359(6378):926–30. 10.1126/science.aar3247 [PMC free article: PMC6080308] [PubMed: 29348365] [CrossRef]
- 13.
- Tie J, Wang Y, Tomasetti C, Li L, Springer S, Kinde I, et al. (2016). Circulating tumor DNA analysis detects minimal residual disease and predicts recurrence in patients with stage II colon cancer. Sci Transl Med. 8(346):346ra92. 10.1126/scitranslmed.aaf6219 [PMC free article: PMC5346159] [PubMed: 27384348] [CrossRef]
- 14.
- Chaudhuri AA, Chabon JJ, Lovejoy AF, Newman AM, Stehr H, Azad TD, et al. (2017). Early detection of molecular residual disease in localized lung cancer by circulating tumor DNA profiling. Cancer Discov. 7(12):1394–403. 10.1158/2159-8290.CD-17-0716 [PMC free article: PMC5895851] [PubMed: 28899864] [CrossRef]
- 15.
- Goh JY, Feng M, Wang W, Oguz G, Yatim SMJM, Lee PL, et al. (2017). Chromosome 1q21.3 amplification is a trackable biomarker and actionable target for breast cancer recurrence. Nat Med. 23(11):1319–30. 10.1038/nm.4405 [PubMed: 28967919] [CrossRef]
- 16.
- Alix-Panabières C, Pantel K (2016). Clinical applications of circulating tumor cells and circulating tumor DNA as liquid biopsy. Cancer Discov. 6(5):479–91. 10.1158/2159-8290.CD-15-1483 [PubMed: 26969689] [CrossRef]
- 17.
- Fehm T, Müller V, Alix-Panabières C, Pantel K (2008). Micrometastatic spread in breast cancer: detection, molecular characterization and clinical relevance. Breast Cancer Res. 10(S1) Suppl 1:S1. 10.1186/bcr1869 [PMC free article: PMC2605098] [PubMed: 19091005] [CrossRef]
- 18.
- Pantel K, Alix-Panabières C, Riethdorf S (2009). Cancer micrometastases. Nat Rev Clin Oncol. 6(6):339–51. 10.1038/nrclinonc.2009.44 [PubMed: 19399023] [CrossRef]
- 19.
- Pantel K, Alix-Panabières C (2013). Real-time liquid biopsy in cancer patients: fact or fiction? Cancer Res. 73(21):6384–8. 10.1158/0008-5472.CAN-13-2030 [PubMed: 24145355] [CrossRef]
- 20.
- Janni WJ, Rack B, Terstappen LWMM, Pierga J-Y, Taran F-A, Fehm T, et al. (2016). Pooled analysis of the prognostic relevance of circulating tumor cells in primary breast cancer. Clin Cancer Res. 22(10):2583–93. 10.1158/1078-0432.CCR-15-1603 [PubMed: 26733614] [CrossRef]
- 21.
- Riethdorf S, Müller V, Loibl S, Nekljudova V, Weber K, Huober J, et al. (2017). Prognostic impact of circulating tumor cells for breast cancer patients treated in the neoadjuvant “Geparquattro” trial. Clin Cancer Res. 23(18):5384–93. 10.1158/1078-0432.CCR-17-0255 [PubMed: 28679772] [CrossRef]
- 22.
- Bidard FC, Michiels S, Riethdorf S, Mueller V, Esserman LJ, Lucci A, et al. (2018). Circulating tumor cells in breast cancer patients treated by neoadjuvant chemotherapy: a meta-analysis. J Natl Cancer Inst. 110(6):560–7. 10.1093/jnci/djy018 [PubMed: 29659933] [CrossRef]
- 23.
- Alix-Panabières C, Mader S, Pantel K (2017). Epithelial-mesenchymal plasticity in circulating tumor cells. J Mol Med (Berl). 95(2):133–42. 10.1007/s00109-016-1500-6 [PubMed: 28013389] [CrossRef]
- 24.
- Ilie M, Hofman V, Long-Mira E, Selva E, Vignaud J-M, Padovani B, et al. (2014). “Sentinel” circulating tumor cells allow early diagnosis of lung cancer in patients with chronic obstructive pulmonary disease. PLoS One. 9(10):e111597. 10.1371/journal.pone.0111597 [PMC free article: PMC4216113] [PubMed: 25360587] [CrossRef]
- 25.
- Wang D, Bodovitz S (2010). Single cell analysis: the new frontier in ‘omics’. Trends Biotechnol. 28(6):281–90. 10.1016/j.tibtech.2010.03.002 [PMC free article: PMC2876223] [PubMed: 20434785] [CrossRef]
- 26.
- Babayan A, Alawi M, Gormley M, Müller V, Wikman H, McMullin RP, et al. (2016). Comparative study of whole genome amplification and next generation sequencing performance of single cancer cells. Oncotarget. 8(34):56066–80. 10.18632/oncotarget.10701 [PMC free article: PMC5593545] [PubMed: 28915574] [CrossRef]
- 27.
- Han JG, Jiang YD, Zhang CH, Yang YM, Pang D, Song YN, et al. (2017). A novel panel of serum miR-21/miR-155/miR-365 as a potential diagnostic biomarker for breast cancer. Ann Surg Treat Res. 92(2):55–66. 10.4174/astr.2017.92.2.55 [PMC free article: PMC5309178] [PubMed: 28203552] [CrossRef]
- 28.
- Tang Q, Ni Z, Cheng Z, Xu J, Yu H, Yin P (2015). Three circulating long non-coding RNAs act as biomarkers for predicting NSCLC. Cell Physiol Biochem. 37(3):1002–9. 10.1159/000430226 [PubMed: 26393913] [CrossRef]
- 29.
- Nilsson RJ, Balaj L, Hulleman E, van Rijn S, Pegtel DM, Walraven M, et al. (2011). Blood platelets contain tumor-derived RNA biomarkers. Blood. 118(13):3680–3. 10.1182/blood-2011-03-344408 [PMC free article: PMC7224637] [PubMed: 21832279] [CrossRef]
- 30.
- Hoshino A, Costa-Silva B, Shen TL, Rodrigues G, Hashimoto A, Tesic Mark M, et al. (2015). Tumour exosome integrins determine organotropic metastasis. Nature. 527(7578):329–35. 10.1038/nature15756 [PMC free article: PMC4788391] [PubMed: 26524530] [CrossRef]
- 31.
- Lane RE, Korbie D, Hill MM, Trau M (2018). Extracellular vesicles as circulating cancer biomarkers: opportunities and challenges. Clin Transl Med. 7(1):14. 10.1186/s40169-018-0192-7 [PMC free article: PMC5981152] [PubMed: 29855735] [CrossRef]
- 32.
- Bracht JWP, Mayo-de-Las-Casas C, Berenguer J, Karachaliou N, Rosell R (2018). The present and future of liquid biopsies in non-small cell lung cancer: combining four biosources for diagnosis, prognosis, prediction, and disease monitoring. Curr Oncol Rep. 20(9):70. 10.1007/s11912-018-0720-z [PubMed: 30030656] [CrossRef]
- 33.
- Heitzer E, Haque IS, Roberts CES, Speicher MR (2019). Current and future perspectives of liquid biopsies in genomics-driven oncology. Nat Rev Genet. 20(2):71–88. 10.1038/s41576-018-0071-5 [PubMed: 30410101] [CrossRef]
- 34.
- Heitzer E, Ulz P, Geigl JB, Speicher MR (2016). Non-invasive detection of genome-wide somatic copy number alterations by liquid biopsies. Mol Oncol. 10(3):494–502. 10.1016/j.molonc.2015.12.004 [PMC free article: PMC5528970] [PubMed: 26778171] [CrossRef]
- 35.
- Silva S, Danson S, Teare D, Taylor F, Bradford J, McDonagh AJG, et al. (2018). Genome-wide analysis of circulating cell-free DNA copy number detects active melanoma and predicts survival. Clin Chem. 64(9):1338–46. 10.1373/clinchem.2018.290023 [PubMed: 29941468] [CrossRef]
- 36.
- Lenaerts L, Vandenberghe P, Brison N, Che H, Neofytou M, Verheecke M, et al. (2019). Genomewide copy number alteration screening of circulating plasma DNA: potential for the detection of incipient tumors. Ann Oncol. 30(1):85–95. 10.1093/annonc/mdy476 [PubMed: 30371735] [CrossRef]
- 37.
- Shen SY, Singhania R, Fehringer G, Chakravarthy A, Roehrl MHA, Chadwick D, et al. (2018). Sensitive tumour detection and classification using plasma cell-free DNA methylomes. Nature. 563(7732):579–83. 10.1038/s41586-018-0703-0 [PubMed: 30429608] [CrossRef]
- 38.
- Uehiro N, Sato F, Pu F, Tanaka S, Kawashima M, Kawaguchi K, et al. (2016). Circulating cell-free DNA-based epigenetic assay can detect early breast cancer. Breast Cancer Res. 18(1):129. 10.1186/s13058-016-0788-z [PMC free article: PMC5168705] [PubMed: 27993161] [CrossRef]
- 39.
- Sina AA, Carrascosa LG, Liang Z, Grewal YS, Wardiana A, Shiddiky MJA, et al. (2018). Epigenetically reprogrammed methylation landscape drives the DNA self-assembly and serves as a universal cancer biomarker. Nat Commun. 9(1):4915. 10.1038/s41467-018-07214-w [PMC free article: PMC6279781] [PubMed: 30514834] [CrossRef]
- Summary
- ctDNA for early detection of cancer
- ctDNA for monitoring of minimal residual disease in patients with early-stage cancer
- CTCs for early detection and monitoring of minimal residual disease
- Liquid biopsy beyond ctDNA and CTC analyses
- Technologies for detection of ctDNA and CTCs
- From discovery to clinical validation and utility
- References
- Circulating DNA and other biomarkers for early diagnosis - World Cancer ReportCirculating DNA and other biomarkers for early diagnosis - World Cancer Report
- NdhD (plastid) [Solanum megistacrolobum]NdhD (plastid) [Solanum megistacrolobum]gi|1610633065|ref|YP_009587736.1|Protein
- N-alpha-acetyltransferase 15, NatA auxiliary subunit b [Danio rerio]N-alpha-acetyltransferase 15, NatA auxiliary subunit b [Danio rerio]gi|830260240|ref|NP_976066.2|Protein
- Solanum andreanum COSII_At3g10920 gene, partial cdsSolanum andreanum COSII_At3g10920 gene, partial cdsgi|224980683|gb|FJ599357.1|Nucleotide
- PREDICTED: double-stranded RNA-binding protein Staufen homolog 2 isoform X16 [Ce...PREDICTED: double-stranded RNA-binding protein Staufen homolog 2 isoform X16 [Cercocebus atys]gi|795591738|ref|XP_011914183.1|Protein
Your browsing activity is empty.
Activity recording is turned off.
See more...