All rights reserved. Publications of the World Health Organization can be obtained from WHO Press, World Health Organization, 20 Avenue Appia, 1211 Geneva 27, Switzerland (tel.: +41 22 791 3264; fax: +41 22 791 4857; e-mail: tni.ohw@sredrokoob). Requests for permission to reproduce or translate WHO publications – whether for sale or for noncommercial distribution – should be addressed to WHO Press, at the above address (fax: +41 22 791 4806; e-mail: tni.ohw@snoissimrep).
NCBI Bookshelf. A service of the National Library of Medicine, National Institutes of Health.
Anthrax in Humans and Animals. 4th edition. Geneva: World Health Organization; 2008.
3.1. Host range, susceptibility and infectious dose
Anthrax is primarily a disease of herbivores (chapter 1). However, reports of its occurrence in a wide range of omnivores, carnivores and other vertebrates are not entirely uncommon, although outbreaks affecting large numbers of carnivorous animals are very rare (see section 3.4.7).
It is the common perception that B. anthracis is not an invasive organism. This is reflected in the generally much higher experimental LD50s by inhalational or oral routes as compared with parenteral challenges in susceptible laboratory species, such as the guinea-pig, mouse or rabbit (Druett et al., 1953; Watson & Keir, 1994). In fact, this had been recognized and studied in the early 1900s. Eurich & Hewlett (1930) cite and discuss seven publications between 1921 and 1928 reporting that animals were much more readily infected percutaneously than by other routes. The following quote from Eurich & Hewlett (1930) illustrates the knowledge of the time:
“Sanarelli (1925) found that large numbers of anthrax bacilli or of spores may be given by mouth to laboratory animals without infecting, and that the blood of an infected animal may be injected per anum without harm. Virulent anthrax spores enclosed in small gelatin capsules may be swallowed by mice and guinea-pigs without harm, though virulent spores can be recovered from the faeces for a week (Holman, 1922).”
Aitoff (1922, also cited by Eurich & Hewlett, 1930) demonstrated that anthrax bacilli would not infect via the conjunctiva, even through conjunctival ulcers. Similarly in livestock, Sterne (1966) noted that “cattle, which are notoriously difficult to kill by parenteral injection, can regularly be killed by dosing with virulent spores made up in pellets of food. Whether the infection is by the buccal or pharyngeal route during rumination, or whether invasion occurs from the stomach, is not known”. Experimental support for this is given by Schlingman et al. (1956).
According to Lincoln et al. (1967), each animal species has a characteristic pattern in the development of septicaemia, with death occurring when circulating bacilli reach a characteristic concentration which, in turn, is directly correlated to the toxin level in blood. An inverse relationship was noted between resistance to infection and susceptibility to the anthrax toxin complex. Rats, highly resistant to infection (parenteral LD50 =106 spores), had terminal bacteraemia in the range of 104 to 105.9 bacilli/ml of blood. In contrast mice, very susceptible to infection (parenteral LD50 = 5 spores) had terminal bacteraemia in the range of 107 bacilli/ml of blood. In challenge with toxin, rats died within 2–20 hours of injection with 15–280 units of toxin/kg, whereas the lethal dose for a mouse was 1000 units of toxin/kg. Two decades later, when purified toxin components had become available, Ezzell et al.(1984) confirmed this earlier observation in Fisher rats, which succumbed to 40 µg PA combined with 8 µg LF, mice to 12.5 µg PA with 2.5 µg LF, and guinea-pigs to 250 µg PA with 50 µg LF. This translates approximately to the µg/kg body weight values given in Table 1.
TABLE 1
Susceptibility of laboratory-animal species to lethal toxin (PA in combination with LF).
Ranges of published LD50s for anthrax by the parenteral route (Schlingman et al., 1956; Watson & Keir, 1994) are:
- < 10–50 for guinea-pigs
- < 10–151 for mice
- 100 for sheep
- 3000 for rhesus monkeys
- 5000 for rabbits
- 106 for rats
- 109 for pigs
- 5 x 1010 for dogs.
There was 100% mortality in nine species of wild Utah rodents injected intracutaneously with 80–100 spores of B. anthracis strain M 36, but in two species of kangaroo rats, doses of 20 000 or 40 000 spores killed fewer than half of the animals tested (Marchette et al., 1957).
Ranges of published LD50s by the inhalational routes (Watson & Keir, 1994; Zaucha et al., 1998) are:
- 16 650–40 000 in guinea-pigs
- 14 500 in mice
- 4000–750 000 in monkeys
- 1.8 x 107 in dogs
- 2.7 x 107 in pigs
- 105 in rabbits.
Ingestion LD50s in guinea-pigs and rabbits exceeded 108 spores (Druett et al., 1953).
Minimum infectious dose (MID) estimates are only rarely available, but:
- British biological warfare work in the early 1940s established that the aerosol MID for sheep was 35 000 spores (Fildes, 1943) and that the dose needed to ensure lethal infection by the oral route in sheep, horses and cattle was 5 x 108 spores (Carter & Pearson, 1999).
- The respiratory MID estimate of 35 000 spores for sheep is consistent with the respiratory LD50 of 200 000 cited by Lincoln et al. (1967).
- Schlingman et al. (1956) found that oral administration of 150 million spores proved fatal to most cattle.
- De Vos (1990) and de Vos & Scheepers (1996) recorded that 100–250 spores of a strain isolated from a kudu in the Kruger National Park consistently resulted in death from anthrax when administered parenterally in impala while the oral LD50 with the same strain in these animals was approximately 15 million spores.
- In a study on 50 pigs given doses of 107–1010 spores in feed containing grit (Redmond et al., 1997), the majority showed clinical illness with recovery, and just two died with confirmed anthrax 6 and 8 days respectively after ingestion of the spores; these were estimated to have received 1.6 x 107 and 7.8 x 107 spores respectively.
- In a letter dated 14 August 1971 from Keppie to Sterne, Keppie concludes: “The [parenteral] sheep LD100 dose appears to be approximately 75–225 spores”. Data from the letter appear in Table 2.
TABLE 2
Lethal doses of the Vollum strain in sheep.
The importance of knowing the MID by various exposure routes is apparent when attempting to reconstruct the epidemiology of certain outbreaks. However, the optimal method of computing an MID from experimental exposure data is subject to debate (Haas, 2002). Since it is thought that, in the natural situation, animals generally acquire anthrax by ingestion of spores, and that some sort of lesion is necessary for the establishment of infection (see section 3.3.1), LD50s or MIDs, particularly parenterally determined, only provide a rudimentary guide to likely infection in the field. Extrapolating experimental findings to the natural situation should also be done cautiously, taking into consideration the many factors influencing infectivity, such as the strain of B. anthracis, the route of infection, the species, breed or strain and state of health of the animal concerned, the times and sites at which tests are done, etc. It is difficult to relate the very large oral doses that are apparently needed experimentally to infect species generally regarded as susceptible to anthrax to the levels of environmental contamination that these animals are likely to encounter naturally and that result in infection and outbreaks in the field. One possible explanation for, or contribution to, the seasonality of anthrax (see section 2.2.5) may be that, for reasons of reduced innate immunity, the MID for at least one or two individuals in the community drops sufficiently in the high season for them to contract infection and then become foci of infection for others.
Innate or natural resistance is covered further in chapter 5.
3.1.1. Mixed infections
It was an early observation (Eurich & Hewlett, 1930, cite four publications between 1889 and 1927) that simultaneous injection of other organisms, such as staphylococci, B. coli, B. typhosus and cowpox vaccine, delayed or prevented death from anthrax in guinea-pigs, mice or rabbits. Fuerst apparently suggested that the mutual antagonism of anthrax bacilli and cowpox vaccine might be used to prevent losses in cattle incurred with the anthrax vaccine (then the Pasteur vaccine, which Eurich & Hewlett record as having mortality rates of 0.1% in cattle, 0.19% in horses and 0.62% in sheep).
3.2. Incidence of anthrax in animals1
Thanks to successful national programmes, there has been a progressive global reduction in livestock anthrax cases over the past three decades. This has had many beneficial aspects but has also created some problems. The latter follow from lack of experience of the disease on the part of younger veterinarians, who may fail to recognize or are slow to diagnose cases. The result of this is that single cases become multiple, sometimes with human exposure, whereas previously it would have been limited to the initial deaths. The other problem is public ignorance of the disease, with the consequent sale and slaughter of affected livestock. This is a particular problem with sheep, where help is requested only when several to many are dead. Because of the resulting environmental contamination, this turns a trivial problem into one that will have long-lasting repercussions. There is also the perennial problem of owners failing to vaccinate livestock in the years immediately following an outbreak while the environmental risk is still present. In Europe as in parts of North America, truly sporadic outbreaks follow from soil disturbance – ditch-clearing, laying water lines, bulldozing roads – where there was an unrealized risk from forgotten cattle graves of past cases (see sections 2.1.2.5, 3.3.2, 8.3.3).
As a result of successful prevention, the disease is absent or only sporadic in the middle and higher latitudes of Europe and the Russian Federation. But it is still common in some countries bordering the Mediterranean (Albania, Greece, southern Italy, Spain and Turkey). In Canada, apart from its continued incidence in and around the Wood Bison National Park in northern Alberta, it is sporadic in southern Alberta and Saskatchewan. Recent events have demonstrated that it has been a long-lasting problem in southern Manitoba because of the cost of vaccination. A change in the provincial law whereby ranchers can now buy vaccine on prescription has resulted in vaccination being affordable and thus routine and successful. In the USA, the disease is confined to a few persistent pockets in North and South Dakota, Minnesota, Nebraska, Nevada and Texas. Elsewhere erratic singular cases have occurred. In western Texas, however, a hyperenzootic situation has arisen in an area of white-tailed deer ranching (see section 3.4.7).
The true situation in Latin America is uncertain and awaits proper definition, largely because the disease is frequently ignored, especially in small ruminants, and underreported. There is a lack of diagnostic facilities. It is enzootic in El Salvador, Guatemala and Mexico, with decreasing incidence as one moves further south. It is absent in Belize and in all of the Caribbean, except Haiti. The situation in Colombia is obscured by civil unrest. The disease is well reported in Chile, but with excess human cases indicating control defects. It is enzootic in Argentina, Bolivia and Peru, and sporadic in western Uruguay and in parts of Brazil.
In South Africa it continues at a low sporadic incidence probably as a result of control being now largely dependent on the livestock owners. Outbreaks occur in wildlife of the Kruger National Park, as in the national parks in Botswana, Namibia, Uganda and the United Republic of Tanzania. The efficient control programmes of the past in Zambia and Zimbabwe are a matter of memory and the disease is now hyperendemic, with significant human losses each year. Epidemics occur in Chad and Ethiopia. The endemic situation in West Africa is made worse by civil wars.
In the Middle East, Israel and the West Bank and Gaza Strip are possibly free. Elsewhere the disease is sporadic, and the Islamic Republic of Iran is attempting eradication. Various levels of endemicity occur in nearby Georgia, Kazakhstan, Kyrgyzstan, Tajikistan, Turkmenistan, Uzbekistan and northern Afghanistan and Pakistan.
Anthrax is a severe problem in southern and eastern India, with a significant human incidence because the disease is poorly controlled. Outbreaks in wildlife also occur. It is absent however from the western state because of the low soil pH.
The disease is a continuing problem in western China, but sporadic in the eastern provinces. Thailand, although essentially free, is afflicted by infected animals imported from Myanmar, some of which have reached Bangkok. The disease is enzootic in Cambodia, Viet Nam and a number of Indonesian islands. Malaysia is free. Sporadic outbreaks have occurred in China, Province of Taiwan, Japan, Philippines, and the Republic of Korea; the situation in the Democratic People’s Republic of Korea is unknown. The disease occurs sporadically in limited areas of Australia, although large outbreaks have occurred at about 30-year intervals over the past 60 years; the disease there is associated with grazing animals. Anthrax has not been recorded in New Zealand for more than 60 years.
Unfortunately, the mere absence of reported outbreaks is no proof of absence of the disease. Insufficient examination of unexpected livestock deaths and reporting deficiencies are worldwide surveillance defects. The restrictions on the use of meat and bonemeals in ruminant feed as a result of bovine spongiform encephalopathy (BSE) cases in Europe has played a significant role in stopping the recycling of the pathogen.
3.3. Transmission, exacerbating factors and epidemiology in animals
3.3.1. Acquisition of the disease
Although anthrax has been recognized for centuries, little is known about the disease, and among the most basic questions frequently asked, but not yet answered, is how precisely do grazing and browsing animals acquire it? The epidemiology of anthrax centres around the dose necessary for infection to occur, and this section needs to be read in conjunction with section 3.1.
The sporulated forms shed by an animal dying or dead from anthrax generally provide the source of infection of other animals (Fig. 2). As noted in section 3.1, it has long been the traditionally held belief that ingestion of the spores while grazing is a frequent mode of uptake. Since B. anthracis is apparently noninvasive, it is believed that a lesion is necessary for the initiation of infection. In view of associations between higher incidence and dry hot conditions, theories have arisen that at such times the animal is forced to graze dry spiky grass close to the soil. The spiky grass and grit produce the orogastrointestinal lesions and, if the soil is contaminated with anthrax spores, these may enable infection to occur. The concept that spiky grass and grit produce lesions is, however, not totally consistent with some epidemiological observations that neither length of grass on pasture nor density of cattle grazing affected pastures correlated with the risk of infection (Fox et al., 1973, 1977).
Ingestion of soil may not be necessary for initiation of infection in grazing animals. In one early study of plants grown on contaminated soil, contamination was alleged to be readily demonstrable on a range of farm crops (Williams, 1932). For example, 92% of corn plants were reported to be culture-positive for B. anthracis from the stems to the tips of the leaves. It is possible to be sceptical about this report, and one would want the identities of the isolates to be reconfirmed but, if this pattern does occur on pasture, close grazing would not be essential for infection.
“Barn anthrax” was an earlier term for animal infections acquired from fodder. An incident at a Pennsylvania dairy farm during 1971 is illustrative of such an outbreak (Kaufmann, personal communication, 2004). Five dairy animals, three cows and two heifers, died over a 10-day period in August. The heifers were kept in a barn with a concrete floor, about a kilometre from the barn where the dairy cows were housed. The heifers were never allowed on pasture and subsisted solely on hay; the primary feed source of the cows was hay, although they did some grazing. Both hay and soil from the farm were culture-positive for B. anthracis.
It is difficult to believe that an animal grazing over dry dusty contaminated soil is not also inhaling spores, and inhalation may well be a mode of infection also. Pulmonary anthrax has been reported in cows (McCulloch, 1961; Bell & Laing, 1977). Acquisition of anthrax through fly-bites probably occurs also. The roles of aerosols and flies are discussed further in sections 3.3.4 and 3.3.5.
Reflecting the generally high infectious dose and noninvasive character of B. anthracis in the natural disease (section 3.1), direct animal-to-animal transmission is understood to occur to an insignificant extent, except in the case of carnivores feeding on other victims of the disease.
3.3.2. Transmission as a result of trade in animal products
In economic and public health terms, the importance of the disease lies in its ability to affect large numbers of livestock at one time and to be spread from these to others great distances away. Anthrax carcasses pose a hazard to humans and other animals both in the vicinity and at a distance through their meat, hides, hair, wool or bones. Hides, hair, wool and bones may be transported long distances for use in industries, feedstuffs or handicrafts. Livestock may acquire the disease continents away from the original infection source through contaminated feedstuffs, or from spores that have reached fields in industrial effluent.
In countries with advanced agriculture, feedstuffs with contaminated ingredients are traditionally the primary source of infection, especially for dairy cows. These ingredients can either be improperly-treated locally-produced meat and bonemeals salvaged from moribund stock or, more likely today, infected bones or contaminated meat and bonemeal imported from enzootic regions. Hugh-Jones & Hussaini (1975) noted an association between the incidence of anthrax in England and Wales from 1938 to 1972 with changes in patterns of importation of meat and bones, or of treatment of these. A decline in incidence in the period 1938–1946 was associated with cessation of imports of bones and meat meals during the Second World War. An increase of importation of these again in 1946–1957 was accompanied by an increase in anthrax, but towards the end of that period, some of the large feed compounders replaced untreated bonemeal with steamed bone flour, and there was a concurrent drop in the incidence of the disease. Finally reintroduction of meat and bonemeal between 1962 and 1964 was again associated with an increase of anthrax in cattle.
It was recognized as long ago as 1911 (Stockman, 1911) that crops such as oats and soya beans, which normally would not be expected to contain B. anthracis spores, could become cross–contaminated by bagging in sacks made of infective materials, such as shoddy wool or horsehair, or during shipping together with dry hides. Stockman wrote: “There are outbreaks in which the circumstantial evidence indicates that infection has been introduced with turnips which have been grown on land manured with crushed bones”. Jackson (1930) stated that instances of cross-contamination of feedstuffs of plant origin had occurred as a result of the hulls of cargo ships and other containers not being cleaned out after transporting infective materials, or following the reuse of sacks previously used for movement of contaminated materials of animal origin. Such occurrences are unlikely today due to the BSE restrictions on the feeding of rendered meat and bonemeal to ruminants.
Tanneries have long been associated with transmission of anthrax. Stockman (1911) wrote: “It has been found that the disease is exceptionally prevalent on certain sewage farms which are known to receive tannery or knackery drainage”. In 1916, Higgins similarly recorded: “One of the most commonly reported sources of infection is that through water and other wastes coming from tanneries”. The anecdotal association of outbreaks of anthrax in livestock and disturbance of soil and tannery wastewater was referred to in section 2.1.2.5, and also features in Fig. 3. On occasion, an incident or outbreak can be related directly to disturbance of an anthrax carcass burial site (Turnbull et al., 1996), while at other times it takes the less focused form of disturbance of the environment downstream from the site of an industrial process involving animal products, such as a tannery.
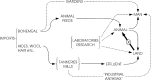
Fig. 3
Importation from endemic countries to other countries, and cycles within the importing country.
3.3.3. Hay, grain, etc
Williams (1932) recounts the problems encountered in controlling anthrax in Texas in the early 20th century, citing a study at the Louisiana Experiment Station demonstrating the potential for anthrax transmission by crops grown on contaminated soil (see also section 3.3.1). In the past, the former Soviet Union has insisted that hay shipped there from the United States be certified as “free of contamination”, presumably reflecting some previous experience of cross-infection. It is unlikely, however, that hay or grain or other crops are significant contributors to the transmission of anthrax today.
3.3.4. Aerosols and wind
It seems reasonable to assume that spores are inhaled by animals grazing over the dry dusty site where another animal died of anthrax at some point in the past (section 3.3.1). On the basis of the experimental findings (see section 5.2) that inhaled spores may remain in ungerminated form in the lungs for months after uptake, it is conceivable that a form of chronic carriage with onset of disease some time after uptake may result (see also section 3.3.8).
As far as long-distance transmission is concerned, basically, anywhere dust can travel, so can anthrax spores. However, the importance of this to transmission of the disease is debatable. Turnbull et al. (1998b) sampled air 6–18 m downwind of old anthrax sites with contamination levels of 2 x 104 to 1.6 x 106 cfu/gram in the semidesert Etosha National Park, Namibia. Sampling was carried out before and after disturbance to simulate animals moving across the site. The highest aerosol concentration found was approximately 2 spores per 100 litres of air. From published respiratory rates, this amounted to a time needed to inhale one spore of 5–7 minutes in sheep and goats, 0.3–0.7 minutes in large animals, and 2.5 minutes in a human. From this, it would seem that the dilution effect of wind spread is too great for wind to contribute greatly to the transmission of the natural disease.
In the event, albeit probably rare, that a case does occur as a result of spores moved by wind, it establishes a potential new focus of infection for other animals. This may have been the case in an instance recorded in Australia many years ago where a storm passing sequentially across an anthrax endemic area and then a clean area was followed by cases of the disease in seven herds in the “clean” area a few weeks later (Henry, 1936).
In contrast to the natural disease, the potential for aerosol spread in a deliberate release event was demonstrated in the accidental release at Sverdlovsk in the former Soviet Union in 1979 (Meselson et al., 1994). Sheep reportedly died of anthrax in a village approximately 60 km distant from the point of release at the research institute, with an estimated 2% death loss overall on affected premises (see also section 4.2.2.3).
3.3.5. Insects
Insects have been incriminated in the transmission of anthrax since at least the 19th century (Budd, 1863; Henning, 1893) and, at the turn of the 20th century, biting flies, particularly Hippobosca and Tabanus species, were considered important as transmitters of the disease (Morris, 1918, 1919, 1920; Sen & Minett, 1944; Sterne, 1959). Davies (1983) believed that tabanids (horseflies) were responsible for the expansion of the huge epidemic in Zimbabwe in 1978–1979 by “hops” from community to community. At the time there were heavy rains which would have encouraged large hatches of tabanids. Rao & Mohiyudeen (1958) also explained the spread of anthrax in India in terms of biting flies. Pangonia spp., biting flies that target hippos, may have played an important role in periodic outbreaks in hippos (Bengis, personal communication, 2005). Non-biting blowflies (Chrysoma albiceps and C. marginalis) have been incriminated as the principal vector of anthrax in browsing wild herbivores in the Kruger National Park, South Africa (Braack & de Vos, 1990; de Vos, 1990; de Vos & Bryden, 1996). Blowflies feed on the body fluids of anthrax carcasses and then deposit highly contaminated faeces or vomit on the leaves of trees and bushes in the vicinity. Browsing herbivores may then contract anthrax when eating the contaminated leaves.
The ability of the biting stable flies (Stomoxys calcitrans) and non-biting houseflies (Musca domestica) or Calliphoridae (bluebottles, blowflies, etc.) to transmit anthrax was demonstrated experimentally by several workers in the early 1900s (reviewed by Sen & Minett, 1944). Transmission by Tabanus rubidus was readily effected in horses and carabao (Kraneveld & Djaenoedin, 1940) and when M. domestica and Calliphora erythrocephala that had fed on incisions in the flanks of goats that had died of anthrax were brought into contact with the cauterized skin of healthy goats (Sen & Minett, 1944). However, the disease was not induced by bringing infected M. domestica into contact with the eyes of healthy goats. Anthrax was also transmitted between infected and healthy guinea-pigs by S. calcitrans and by mosquito species Aedes aegypti and A. taeniorhynchus (Turell & Knudson, 1987). Schuberg & Boing (1913) are cited without reference by McKendrick (1980) as also having demonstrated the ability of mosquitoes to transmit anthrax.
Ticks collected from terminally ill animals have been found to carry B. anthracis (Stiles, 1944; Buriro, 1980; Akhmerov et al., 1982) but they probably do not play a significant role in the transmission of the disease as interhost transfer of adult ticks is rare.
Dose is again pertinent. Small insects may pick up too few B. anthracis from a sick or dead animal to definitely induce infection in another host (Greenberg, 1971, 1973), although this may be countered by the cumulative effect of several or numerous bites. During “epidemics”, such as in Texas or Canada, affected stock have been noted to be “black” with biting flies. However, it is necessary to bear in mind the finding by Schlingman et al. (1956) that lethal infection could not be induced in cattle by parenterally administered doses of up to 6 x 108 spores of the Vollum strain. The Vollum strain is regarded as less virulent than many other strains but, if this is taken as the minimum lethal parenteral dose for cattle, and terminal blood counts in anthrax victims at death are in the order of 108 cfu/ml (see section 3.1), it would imply that 1–2 ml (cumulative) of blood needs to be injected by tabanid mouthparts. In the experimental studies reviewed and carried out by Sen & Minett, the transmission success rate was generally quite low. It can be seen, however, that this mode of transmission could be expected to select out more virulent strains requiring lower volumes of injected blood (Hugh-Jones, personal communication, 2003).
The time between the exposure of the insect vector and the exposure of the second host to that insect was of consequence. The transmission rates in Turell & Knudson’s study were 17% for stable flies and 12% for mosquitoes, and all transmissions occurred in guinea-pigs exposed ≤ 4 hours after uptake of blood by the insect from the bacteraemic animal.
According to Kraneveld & Mansjoer (1939) the bacilli did not multiply in the gut of tabanids; some were excreted in vegetative state, some died and some were able to sporulate. De Vos (de Vos & Turnbull, 2004) states that blowflies may be lifelong carriers, although vegetative B. anthracis disappear from their digestive tracts within two weeks of feeding on a carcass.
The possible association between flies and the seasonality of anthrax is discussed in section 2.2.6.
3.3.6. Other modes of mechanical transmission
Cattle drives in the 19th and early 20th centuries have long been regarded as a major means of the early spread of anthrax in Canada and the USA. A comparison of the distribution of affected counties in the first half of the 20th century (Hugh-Jones, unpublished data) shows that, for the period up to 1932, there was a 1:3.99 chance of a county having livestock anthrax if it was within 10 km of a south-to-north cattle trail (p < 0.0003); for trails going east to west (essentially all these were immigrant trails, such as the Oregon and California trails), the chance was 1:0.60 (p < 0.4175).
Livestock began arriving in large numbers in what became the anthrax-endemic regions of northern Canada as early as 1880 from herds in the north-western American states and southern Alberta, and may have been the source of the disease (Dragon et al., 1999). Alternatively, it has been hypothesized that anthrax in northern Canada originated from Wainwright in southern Alberta, along with brucellosis and tuberculosis, when excess plains bison that had been in contact with local cattle were moved to the north in the mid-1920s. The reflux of B. anthracis bison strain GT5 down into central Alberta probably came from contact between southern cattle grazing in the north at Fort Vermilion during the summer and wandering bison bands out of the Wood Bison National Park (Hugh-Jones, personal communication, 2004).
Livestock movements along the Silk Road probably took anthrax eastwards into western China.
The spread of anthrax by carrion eaters was discussed by Kraneveld & Mansjoer (1941) and vultures and other scavengers have long been suspected of transmitting the disease various distances in southern Africa (Pienaar, 1967; de Vos, 1990; Lindeque & Turnbull, 1994; Lindeque et al., 1996a). Similarly gulls, ravens and possibly black bears were considered as possible vectors in the bison outbreaks in northern Canada (Dragon et al., 1996). Certainly anthrax spores have been found on/in the feathers, faeces or digestive tracts of these animals (Lindeque & Turnbull, 1994; Dragon et al., 1996; Lindeque et al., 1996a), but the numbers are generally low and below what is generally regarded as the “normal” infectious doses of other animals. However, as with aerosols and other modes of mechanical transmission, if and when an occasional case does occur, it may set up a new focus of infection for other susceptible animals in the vicinity.
3.3.7. Age and sex
In general, although reliable evidence is difficult to come by, the incidence of anthrax in wild animals seems to be slightly biased towards adulthood and males (Pienaar, 1961; Moynihan, 1963; Brunsdon, 1968; Ebedes, 1976; de Vos, 1990; Lindeque & Turnbull, 1994; de Vos & Bryden, 1998). However, this may be apparent rather than real, reflecting factors other than resistance to infection, such as differences in behaviour or feeding habits or, in the case of age, the relative ease with which carcasses of adults that have died of anthrax may be detected as compared with those of the young animal. Physiological factors may be involved also. Weinstein (1938) showed that administration of thyroxine, testosterone and pituitary, thymus and pineal gland extracts to mice increased their resistance to anthrax infection while insulin, oestrin, progesterone and adrenal cortex extract did not.
Of particular interest are the bison in an anthrax-enzootic area in northern Canada where the majority of carcasses found are sexually mature bulls. Special surveillance techniques during the 1993 outbreak established that bull-bias in mortality was real and not due to calf carcasses being more difficult to spot (Gates et al., 1995). Except for one outbreak in the summer of 2001, where an equal number of bull and dam carcasses were found, a bull-bias of > 80% has been observed in all known anthrax outbreaks in the Canadian bison herds (Dragon & Elkin, 2001). Explanations for this range from the rutting behaviour of the bulls causing exposure to spore-laden dust, to chronic infection becoming acute from stress-related factors during the rutting season. Bulls use the wallows for dominance displays against each other and generally die within 100 metres of their individual wallows. Bulls generally graze closer to the ground than cows and calves. The next bull adopting the wallow of one that has died and grazing around it becomes relatively exposed to the deposited spores (Hugh-Jones, personal communication, 2004). Also, females rarely wallow and calves, being suckled, do not graze much; they may also have maternal antibody.
Information found on age- and gender-related incidence in livestock is limited. Significantly disparate attack rates were noted between bulls, cows and calves in Louisiana and Texas during outbreaks in the 1970s, with males having the highest attack rates and the incidence in calves being nil (Fox et al., 1973, 1977). In Louisiana, the attack rates in steers and cows were similar, suggesting that a factor unique to intact males resulted in their increased susceptibility to infection. The explanation offered for the age-specific differential attack rate has been that calves, as they are suckling, would ingest less forage than adults on the same pasture (Fox et al., 1973).
No calves were infected in the outbreak in Victoria, Australia, in 1997. This could have been due to movement of the weaned dairy calves to a raising shed away from the herd.
3.3.8. Prolonged incubation, carrier state, chronic and latent infections
A differentiation must be made between prolonged incubation, carrier state, and chronic and latent infection. The lengthy period between exposure and onset of clinical illness that was observed in experimental monkeys exposed to spore aerosols (see section 5.2) and in humans exposed in the Sverdlovsk accidental release incident (Meselson et al., 1994) are examples of prolonged incubation. Another example is the vaccine trial of Lindley (1955) described in the next paragraph. A carrier state, in the sense of animals harbouring the specific organisms of a disease without overt symptoms and being capable of transmitting the infection, has not been demonstrated in anthrax. A chronic infection results in antibody production and deterioration in health of the infected individual. Latent infection implies that spores are lodged in one or more lymph glands or other sites, successfully germinating and initiating infection when the innate immunity is depressed. However, in some of the instances cited below it is difficult to make the distinction between prolonged incubation, carriage, and chronic and latent infection, and the terminology of the original reports has been retained in the following paragraphs.
As an example of prolonged incubation, Lindley (1955) observed anthrax deaths in two goats, 47 days and 51 days post-challenge with the virulent Gwandu strain of B. anthracis administered as a spore suspension in 50% glycerin. Both animals had been housed in an indoor stable, and no infections occurred in unchallenged animals at the facility. The one that died 51 days post-challenge had been vaccinated. The unvaccinated animal, in particular, illustrates a potential long incubation period.
Chronic anthrax infections have been reported in swine (Hutyra et al., 1946). Only a few decades ago, meat inspectors in slaughterhouses periodically observed discrete anthrax lesions in the lymph nodes of the mesentery, pharynx or submandibular region of swine. The lymph nodes were generally enlarged, and the cut surface was brick red or studded with small greyish-yellow necrotic foci, sometimes diffusely necrotic and containing dry caseous material. Lymph nodes of this description were found nine months after the 1952 three-state epidemic in pig herds in Ohio, Indiana and Illinois affecting 490 herds and attributed to contaminated pig feed (Hugh-Jones, personal communication, 2004). Presumably, other resistant scavenger species such as dogs can also develop chronic lesions.
Among susceptible species, Weidlich (1934) reported the case of a cow producing apparently normal milk containing anthrax bacilli for some months. Provost & Trouette (1957) isolated B. anthracis from the abdominal lymph nodes of apparently healthy cattle in an endemic area of Chad. Culture collection strain ASC 65 was from the milk of one of four chronically infected cows in Brazil. The bacterium was isolated from these cows (apparently healthy at the time, although two unvaccinated animals subsequently died) during routine mastitis screening tests on milk samples in 19832 (see also Annex 6, section 3).
Evidence of carriage in susceptible herbivores has been reported. De Moulin & Soemanegara (1937) noted that spores administered subcutaneously in highly immunized animals were still present at the site of inoculation ≥ 7 weeks later in cattle, ≥ 2 months in horses, buffalo and sheep and ≥ 6 weeks in chickens. Dormant spores were found to still be circulating in the blood of black rats at 30 days by Walker et al. (1967). Ferguson (1981) reported a carrier state in pigs that had recovered from the disease.
A serological survey in the enzootic Etosha National Park, Namibia, showed that Park herbivores rarely have natural anti-anthrax antibodies, while most of the Park carnivores have them (Turnbull et al., 1992a). This was interpreted as indicating that infection in herbivores usually leads to death. In contrast, however, it was found that 87% of bison bulls in the Canadian Mackenzie Bison Sanctuary had specific anti-anthrax antibodies when tested eight months after an outbreak in 1993 (Turnbull et al., 2001b). The implication in this instance is that a significant proportion of the bison survived B. anthracis infection or were chronically infected. Passively transferred or partial immunity can delay onset of overt disease by many days, which again represents a form of latent infection (Turnbull, 1990b).
Latent infections may conceivably be the occasional explanation for the long-distance spread of anthrax. However, the success of the application of the OIE 20-day incubation period for anthrax control in livestock over the past 50 years is testimony to the rarity of this, if it occurs at all.
Much has been made in recent years (in relation to formulating post-exposure prophylaxis in humans) of the finding that inhaled spores may lie dormant in the lungs of monkeys for weeks before being cleared by alveolar macrophages, showing no evidence of germination until within the macrophages (Barnes, 1947a; Henderson et al., 1956; Widdicombe et al., 1956; Ross, 1957; Friedlander et al., 1993; see section 5.2). The relevance to the natural disease and its spread in animals is uncertain.
3.3.9. Anomalies and unknowns of anthrax epidemiology
Despite anthrax being a disease that has been so well known for so long, many seemingly simple epidemiological questions remain unanswered. A variety of explanations have been developed on the factors that lead to outbreaks of anthrax. The explanations remain just speculation and vary with respect to different environments, different continents and whether domestic or wild animals are involved.
Examples of continuing unknowns follow.
3.3.9.1. How does an animal acquire anthrax?
How an animal contracts the disease remains in the realm of theory (see section 3.3.1).
3.3.9.2. Not always point-source?
Outbreaks of anthrax are generally considered to be of the point-source type; direct herbivore-to-herbivore transmission is thought not to occur (with the exception of transmission as a result of osteophagia) and certainly does not occur under laboratory conditions (i.e. in animal cages in research studies). However, it is difficult to explain periodic explosive epizootics, or unexpected spread to areas that were previously unaffected, in terms of point-source infection.
The history of outbreaks in Australia is that outbreaks start as a point-source death with 2, 3 or 4 further cases occurring in 5–7 days, and 7–8 deaths in a further 5–7 days. This pattern of spread has been attributed to the social cohort of dead animals licking and sniffing around the carcass, and this was the theory accepted in the large 1997 outbreak in Victoria State.
Fly-borne spread (section 3.3.5) is strongly suspected of being the means of spread in such events.
3.3.9.3. Why do outbreaks predominantly affect one species?
Large explosive outbreaks usually affect only one species in the affected area while the incidence in other, equally susceptible species in the vicinity remains sporadic. This has been known for a long time. Higgins (1916) wrote: “In certain outbreaks a single species of animal may show a more marked susceptibility than others which are apparently similarly exposed”. The phenomenon may be explained in terms of the different ecological niches of the various species and that they are, in fact, not at equiprobable risk within the same setting. A simple example might be that if biting flies are responsible for the outbreak, they bite the primary species preferably or exclusively.
Susceptibility data based on experimental results do not correlate well with the occurrence of deaths under natural circumstances (Sterne, 1959). Cattle are quite resistant to experimental infection but are very prone to infection in nature. In contrast, sheep are quite susceptible to experimental infection but may not be so readily infected in nature, even on premises where affected cattle are grazing. Consistent with Sterne’s observation, a large outbreak in California in 1968 resulted in 176 animal deaths (169 cattle, 4 mules and 3 horses), yet sheep in close proximity were not affected (Kaufmann, personal communication, 2004). Sterne also pointed out that goats, despite being even more susceptible than sheep to experimental infection, accounted for even fewer cases than sheep (see below).
Wise & Kennedy (1980) noted that New South Wales, Australia, could be divided into a northern zone in which bovine and ovine anthrax occurred with equal frequency, and a southern zone where bovine anthrax was about four times as common as ovine, with death rates in cattle 13 times greater than in sheep. In the 1997 outbreak in Victoria, some 202 cattle and 4 sheep died. The reasons for such area variations in the two species are unknown, particularly as they are interspersed, often on the same property, across all these areas. Although cattle and sheep do exhibit different grazing behaviours, it is hard to define the reason why one species is favoured over the other for infection in one area or property.
In the wild, anthrax does not affect all herbivorous species equally, and there is an apparent preference by the disease for particular species in any one region. Zebras, for example, are the most commonly affected in the Etosha National Park in northern Namibia, with kudu only occasionally affected. Assessments of all recorded anthrax deaths (uncorrected for total populations) are in the order of 45% for zebra versus 0.8% for kudu (Lindeque & Turnbull, 1994). In the Kruger National Park, South Africa, the kudu is the principal host, accounting for > 50% of all recorded anthrax cases with zebra falling into a relatively small group of “other affected species” (de Vos, 1990). The ecological and behavioural factors responsible for these differences tend to depend on circumstantial evidence, but appear to revolve around blowflies in the Kruger National Park which, after feeding on anthrax carcasses, fly to nearby shrubbery and deposit infected blood on the leaves, which then become the source of infection for the browsing kudus. The analagous situation with goats and sheep is referred to above; goats are allegedly infected less often than sheep under equiprobable risk situations, despite being more susceptible to experimental inoculation. Goats, like kudus, are browsers; sheep, like zebras, are grazers.
Few disagree that grazing, browsing and flies are the main variables in the differing equations; flies are the vectors of spores from dead carcasses to foliage in the vicinity. Other aspects relating to the manner in which plants are eaten are also likely to affect acquisition of anthrax. Bovines pull plants out of the ground when grazing, thereby ingesting a lot of soil. In contrast, sheep and horses bite plants off at ground level, taking in very little soil (Hugh-Jones, personal communication, 2004).
Strain differences come to mind as an obvious explanation in relation to these anomalies. In Texas, goats and white-tailed deer frequent the same areas but, if horseflies are transmitters of the disease, they fail to infect the goats (Hugh-Jones, personal communication, 2004). Is this strain-related? This has yet to be proven (see section 2.3).
3.3.10. Transmission resulting from deliberate release – bioaggression
The realities of transmission of anthrax as a result of deliberate release were made very clear by the anthrax letter events in the USA between September and December 2001, although those were targeted at humans rather than animals. The potential damage that could be inflicted from targeting livestock has, however, been recognized for at least a century. The 1899 Hague Convention, adopting the unratified Brussels Declaration of 1874, prohibited “the spreading ... of diseases on enemy territory”. Although the illegality of biological warfare was spelt out in the 1902 German General Staff handbook on land war, Germany ran an anti-animal sabotage programme from 1915 to 1918 largely targeted at neutral countries (Argentina, Norway, Romania, Spain and the United States) to disrupt their shipments, primarily of horses destined for military use, to the enemy belligerents. Glanders and anthrax, the agents involved, were delivered by crude inoculation with a needle dipped into the cultures. No data exist on the level of damage inflicted by this programme, although it was considered a “success” by the German army (Wheelis, 1999).
It seems that after the First World War, thinking about biological warfare (BW) became focused on antihuman agents, and veterinary sabotage was no longer considered relevant in the German programme (Wheelis, 1999; Geissler, 1999). Possibly owing to a misunderstanding in intelligence coding, perception that Germany had an antilivestock biological warfare programme led to a British development and production capability retaliation-in-kind programme (Carter & Pearson, 1999). Between September 1942 and April 1943, 5 million cattle-cakes were prepared and stockpiled ready for targeting Germany’s wartime agriculture section. Encapsulated within the centre of each cake were 5 x 108 anthrax spores (established MID for cattle – see section 3.1) of the Vollum strain. The cakes were never used and were destroyed shortly after the war.
The possibility that the epidemic of anthrax in Zimbabwe (then Southern Rhodesia) in 1979–1980 was the result of deliberate release has been raised. Epidemiological details of a large proportion of the > 10 000 human cases were published (Davies, 1982, 1983) and “Contact with a dead bovine carcass is always given as the manner in which anthrax is acquired. ... Confirmation of anthrax as the cause of cattle deaths is available from all areas in which (human) anthrax is thought to have occurred” (Davies 1982); “two thirds of the country has been affected by bovine anthrax in a very short time” (Turner, 1980). Although further data on the livestock cases were not published, it was believed that tabanids (horseflies) spread the disease among animals (Davies, 1983; see section 3.3.5), and the breakdown in veterinary services resulting from the insurgency situation occurring at the time prevented the setting up of vaccination campaigns (Davies, 1982) and of education programmes aimed at stopping people from butchering the carcasses. However, some years later, accusations arose in the public press and minor publications that this had been a “deliberate use of anthrax as an agent of BW, directed at African-owned cattle” (Nass, 1992) and that “the use of anthrax spoor to kill off cattle of tribesmen assisting the guerillas ... was carried out” (Martin, 1993). In defence of the official stance that this was a natural outbreak resulting from the breakdown of the veterinary services (commercial farms, where vaccination was still possible, remained virtually free of infection – Davies, 1983), it was pointed out by government officials that other diseases, such as rabies and tick-borne infections, escalated several 100-fold at the same time.
The accidental release of anthrax at Sverdlovsk in the former Soviet Union in 1979 (see also sections 3.3.4, 4.2.1.1, 4.2.1.3, 4.2.2.3) originated from a microbiology laboratory and caused the death of a significant number of livestock up to approximately 60 km from the point of release (Meselson et al., 1994).
3.4. Clinical manifestations; incubation periods
The incubation period in susceptible laboratory animals ranges from about 36 to 72 hours. In susceptible livestock, it may be a little longer. Schlingman et al. (1956) found an incubation period of 3–7 days following oral challenge. The normal incubation period in naturally-infected cattle is stated to be 1–14 days or more (Kaufmann, personal communication, 2004). For trade purposes, the OIE incubation period for anthrax is 20 days (Annex 4). The requirement that animals for export will not have been in contact with an infected animal or premises in the previous 20 days appears to have been effective in controlling the spread of anthrax through international trade and also seems to work in controlling dissemination of infection through domestic trade (Turner, personal communication, 2004).
In an experimental study on pigs, the majority of 45 pigs challenged orally with high doses of the Ames strain of B. anthracis showed nonspecific clinical signs of illness (fever, anorexia, lethargy, dullness, shivering, constipation, loose faeces, blood in the faeces and ataxia) between 1 and 8 days after challenge; rectal temperatures increased at 24 hours, peaking at 48 hours and returning to normal by 7 days. Two of the animals died suddenly on days 6 and 8 (Redmond et al., 1997).
A dose-response curve in rhesus monkeys challenged with 105 to 5 x 1010 spores showed that death occurred in progressively shorter times from 50 to 20 hours (Klein et al., 1962).
The first signs of an anthrax outbreak are one or more sudden deaths in affected livestock, although farmers may reflect retrospectively that the animals had shown signs such as having been off their food or having produced less milk than usual. During the systemic phase, the animals become distressed, appear to have difficulty breathing and cease eating and drinking. Swellings in the submandibular fossa may be apparent; temperatures may remain normal for most of the period or may rise. The animal can remain responsive to treatment well into this period, but if treatment fails it lapses into coma followed by death from shock. In highly susceptible species, the period between onset of visible symptoms and death may be just a few hours; the course of these events is more protracted in more resistant species or in immunized animals when the protective effect of the vaccine is wearing off. In studies on immunized laboratory animals, this has been shown to be associated with reduced terminal bacteraemia and reduced circulating toxin (Turnbull, 1990b).
The history is of major importance to immediate suspicion of anthrax. In particular, if deaths occur unexpectedly in areas known in the past to have experienced anthrax, whether recently or not, then the diagnosis of anthrax should be regarded as a possibility. This is even more the case if the deaths follow recent disturbance of the land such as digging, ploughing, dredging of watercourses and so on (see sections 2.1.2.5, 3.3.2). Anthrax should always be considered in the event of sudden deaths in zoos or other captive facilities where meat from knackeries or the equivalent are regularly fed to animals.
Cutaneous lesions, analogous to the eschars of human cutaneous anthrax (section 4.4), are rarely reported in animals. Maculopapular swellings with vesicle formation and oedema were reported in intracutaneous infections in wild Utah rodents (Marchette et al., 1957) and carbuncular lesions are referred to in sections 3.4.1, 3.4.5 and 3.4.6.
3.4.1. Bovines
In the bovine, anthrax often occurs as an acute febrile disease without obvious localization, although subacute disease with throat swellings may be encountered in tropical countries, possibly through buccal lesions from chewing infected bones (Sterne, 1959). A steep rise in temperature is a typical sign, and irritability may be followed by dullness. If no treatment is given, death usually occurs in 2–3 days with the animal showing cramplike symptoms and shivering. The urine may be blood-stained and blood may exude from the rectum and other natural openings. Often advanced signs are minimal, and the apparently healthy animal may fall in an apoplectic seizure and die within a few minutes to a few hours. Earlier clinical opinions that recovery is not an infrequent event (Sterne, 1959, 1966) are supported by more recent serological evidence (Turnbull et al., 1992a). According to Sterne (1959), oedematous or carbuncular lesions are associated with immunized animals when their resistance is waning.
Oedematous swellings along the neck, flanks or lumbar region have been described in cattle with experimental anthrax infections (Jackson et al., 1957).
The situation regarding milk is discussed in Annex 6, section 3. A single report of B. anthracis-associated bovine abortion was seen (Gibbons & Hussaini, 1974).
3.4.2. Sheep and goats
Anthrax usually takes the form of a peracute apoplectic infection. This may be modified in animals that have been immunized.
3.4.3. Horses
Horses may show acute symptoms and die in 2–3 days. Intestinal lesions may result in colic and diarrhoea. Sterne (1959) associated large oedemas on the breast, abdomen, neck and shoulders with cases transmitted by biting flies. It is to be assumed he observed this in southern Africa. Recovery sometimes occurs.
3.4.4. Pigs
Pigs are regarded as more resistant to anthrax than cattle, sheep, goats and horses, but herd outbreaks with significant mortality can occur. Their greater resistance is reflected in the greater evidence of local signs, such as swellings of the throat and pharyngeal and cervical lymph glands. As reviewed by Redmond et al. (1997), outbreaks in pigs were recorded with particular frequency in the 1950s and may have reflected postwar husbandry practices of feeding food waste which inadvertently included meat and bones from anthrax carcasses. At that time also, it was accepted that there were two principal manifestations of porcine anthrax: the pharyngeal and intestinal forms.
The pharyngeal form, not frequently reported now, was related to scavenging or purposeful feeding of carcasses to swine and characterized by an ulcerative stomatitis, laryngitis, and markedly oedematous swelling of the throat that could interfere mechanically with respiration, feeding and drinking. There is reference in Nieberle & Cohrs (1967) to demonstration of invasion via the epithelium overlying the tonsils. One theory is that it was associated with lesions from bone chips in food waste, but Kaufmann (personal communication, 2004) recalls that major outbreaks in the United States in the early 1950s were due to contaminated bonemeal which was finely ground and did not contain jagged chips.
The intestinal form, thought to be associated with contaminated mineral supplements, is less obvious than the pharyngeal form, lacking the obvious swellings and associated effects. Because the animals frequently recover, its occurrence may often have gone undiagnosed. Anorexia, vomiting, diarrhoea (sometimes bloody) or constipation have been reported. In an experimental study on 50 pigs (Redmond et al., 1997), anorexia, lethargy, dullness, shivering, constipation or loose faeces, blood in the faeces and ataxia were the symptoms variously noted in 33 animals showing symptoms. In 45 of the animals, rectal temperatures indicating pyrexia rose in 24–48 hours after ingestion of anthrax spores, decreasing to a minimum at about 8 days. Marked seroconversion occurred in all but 4 of the animals, indicating that infection with recovery was the common outcome (only 2 pigs died). The induced infection took exclusively the intestinal form.
It is possible that the two manifestations of the disease in pigs relates to whether vegetative cells are being consumed in addition to spores. Infection resulting from consumption of meat and bones from infected carcasses, which would contain both vegetative cells and spores, may be associated with the pharyngeal form of gastrointestinal anthrax. The intestinal form is associated with consumption of spore-contaminated mineral supplements which would lack the vegetative forms (see also section 4.2.2.4).
The number of circulating B. anthracis in the blood of pigs at death is very low compared with most other species. This has practical consequences for diagnosis (see section 3.5.1).
3.4.5. Dogs and cats
Dogs are considered very resistant to anthrax, but reports in dogs that have scavenged anthrax carcasses are not exceptionally rare. In the United Kingdom, within living memory, retired foxhounds scavenging when dead cows were collected from the hunt area acquired the disease (Hugh-Jones, personal communication, 2004). Villagers in endemic countries of at least sub-Saharan Africa recognize anthrax in their cattle and, although they will eat meat from the carcass (sections 4.1 and 9.7), they will not eat the enlarged spleen; instead, they will frequently feed these to dogs and cats. Several dogs and cats were reported to have died of presumed anthrax during an epidemic involving some 500 cattle and 50 humans in the Zambezi plains area of Western Province, Zambia, in 1990–1991. Sera from 3 of 3 dogs that had eaten meat from suspected livestock anthrax victims had measurable antibody titres (Muyoyeta, Bbalo & Turnbull, unpublished data, 1994).
Severe inflammation and oedematous swelling of the throat, stomach, intestine and of the lips, jowls, tongue and gums may be seen. Carbuncular lesions of the jowls occur. Infection may generally not be lethal. A few reports of anthrax in domestic cats allegedly exist also (Eurich & Hewlett, 1930; Whitford, 1979).
3.4.6. Birds
Sterne (1959) referred to “epizootics amongst birds”, but without reference or details. References found to cases of anthrax in avian species are largely confined to birds in captivity or domesticated state. While there is some logic to reports of the disease in eagles in captivity following inadvertent feeding with an anthrax carcass (Keymer, 1972; Turnbull, 1990), more surprising are the citations found to the disease in not overtly carnivorous birds including ducks, a hen, geese, artificially-infected poultry and pigeons, and even a crested crane. Again without reference or detail, Sterne (1959) stated that domestic ostriches readily contract anthrax. A recent case (June 2005) in an ostrich in the Etosha National Park, Namibia (Lindeque, Versfeld & Turnbull, personal communication, 2005) reflects the report of cases in 5 wild ostriches in the 1970s in the Park by Ebedes (1976). Other early references exist of the disease in ostriches (Viljoen et al., 1928; Ebedes, 1976; Hugh-Jones & de Vos, 2002). Pasteur (cited by Klemm & Klemm, 1959) attributed resistance to anthrax in birds to their body temperature being higher than that of a mammal, and Higgins (1916) stated that anthrax may be contracted by birds “providing ... their normal body temperature is lowered”. Pasteur’s experiments with hens, attributing resistance against anthrax in birds to their high body temperature, are described in detail by Vallery-Radot (1923).
According to Sterne (1959), the apoplectic type of death is usual, although less acute infection, with carbuncular lesions on the comb or extremities, also occurs. As reviewed by Snoeyenbos (1965), the lesions of anthrax in the ostrich, duck and eagle are similar, with haemorrhagic enteritis and oedematous swellings, particularly in the neck. Haemorrhages may be present on the surface of many organs, such as the heart. Excessive fluid is commonly present in the thoracic and abdominal cavities. The spleen, liver and kidneys are swollen and congested.
Reference to a vulture belonging to a travelling menagerie that became very sick during an outbreak of anthrax affecting many of the animals is made in section 3.4.7. De Vos (personal communication, 2006) recalls confirming death from anthrax in one vulture by blood smear and subsequent culture from the blood. It was, however, the only bird found dead out of a very large number feeding on carcasses during a large outbreak of the disease in the Kruger Park, South Africa.
3.4.7. Other animals
Anthrax is well known as a disease of wild mammals. The largest number of reports comes from national parks in southern and central Africa, where a wide variety of species are affected. It has also been reported for many years in white-tailed deer in the southern USA (Marburger & Thomas, 1965; Yu et al., 2002) and has been observed to be enzootic in free-ranging bison in northern Canada for over four decades (Gainer & Saunders, 1989; Gates et al., 1995; Dragon & Elkin, 2001). In these areas, occasional secondary cases occur in other species (including carnivores) making contact with the primary species. Although the disease is enzootic in other parts of the world, there seems to be very little information on its occurrence in wildlife in these parts, presumably as a result of lack of resources to investigate causes of death in the wild.
Carnivores are, in general, significantly more resistant than herbivores (section 3.1) but can die from infection. in enzootic areas, resistance in wild carnivores is enhanced by the humoral immunity acquired naturally as a result of frequent exposure to scavenging carcasses of anthrax victims (Turnbull et al., 1992a). The antibody titres in the carnivores reflect (i) the level of anthrax activity in an area, and (ii) the habits of those carnivores. it seems likely that the carnivores depend on the antibodies to protect them from lethal infection. The increasing incidence of canine distemper in lions in African parks may be behind increased numbers of observed cases of anthrax in lions in recent years; a side-effect of the distemper is to damage the immune system.
Cheetah appear to represent an unusual group among carnivores in relation to anthrax. it has recently been observed in Namibia that cheetah suffer a high mortality from anthrax (Lindeque et al., 1998). Cheetah do not normally scavenge and may not, therefore, get the same chance as other carnivores to build up acquired immunity. Their unique genetic constitution may also play a role in susceptibility to infection through its effect on their immune system.
Among other animals, anthrax has been recorded in camels (Eurich & Hewlett 1930; Punskii & Zheglova, 1958; Musa et al., 1993), carabao (Steele, 1954), mink (Greener, 1947; Sterne, 1959; Hugh-Jones & Husseini, 1975), badgers, ferrets and racoons (Somers, 1911; Greener, 1947) and foxes (Eurich & Hewlett, 1930; Greener, 1947). A 1945 handbook (Anon., 1945) states: “in the domestic animals, sheep (excepting Algerian sheep which are immune), cattle, camels and horses are susceptible in the order named”. Whitford (1979) tells us that Gayot (1952) showed that, in fact, Algerian sheep were as susceptible as other sheep. Cases of the disease in moose have been seen in both Canada and the USA (Dragon & Elkin, 2001; Kaufmann, personal communication, 2004).
The list of species that have experienced anthrax in captivity (zoos, capture and release facilities, wildlife orphanages, etc.) is wide-ranging (Hugh-Jones & de Vos, 2002). Mostly these have been carnivores fed on abattoir or knackery offal or, inadvertently, on portions of carcasses from animals that died of anthrax (Lyon, 1973; Hugh-Jones & Husseini, 1975; Orr et al., 1978; Turnbull, 1990b; Tubbesing, 1997; Hugh-Jones & de Vos, 2002). A particularly interesting very early report (Somers, 1911) describes an outbreak occurring in Bolton in the United Kingdom in a travelling menagerie that resulted in the deaths of two racoons, a coypu, an English badger, two pumas, a leopard, an ant-eater, two polar bears and a lioness. Two lions and a vulture became very sick but recovered. The animals had been fed raw meat from several sources and the particular source was not traced. A very recent report is that of Grigoryan (2002) in which a silver puma, a serval and a black lynx died of anthrax in an Armenian zoo after being fed contaminated meat purchased at a local market. Reference was made in section 3.1 to susceptibility tests carried out on 12 species of wild Utah rodents injected intracutaneously with spores of B. anthracis strain M 36 (Marchette et al., 1957).
Sudden death in apparently healthy animals with a very short period of disorientation before falling is the normal clinical picture up to death. Death may be accompanied by bloody discharges from natural orifices (Fig. 2) but it is not a constant. Rapid bloating of the carcass, incomplete rigor mortis and the absence of clotting of the blood are other common characteristics. The bloody discharges were not seen in Texas deer dying in various epidemics and only rarely in Canadian bison dying of anthrax (Gates et al., 1995; Dragon & Elkin, 2001).
Clinical information for carnivores is supplied by Lyon (1973) and Tubbesing (1997). Anorexia, lethargy, dyspnoea, serous nasal discharge, unilateral facial and/or submaxillary swelling with convulsions and death are variously noted by Lyon in the 13 carnivorous animals with anthrax in the Chester Zoo, United Kingdom, in 1971. Tubbesing records the death from anthrax of a leopard showing no clinical signs prior to death, a second leopard which became lethargic and anorexic, a caracal noted to be listless, anorexic and having signs of facial oedema, a lion showing signs of lethargy and partial anorexia and a lioness with lethargy, anorexia and facial swelling. All the animals that developed clinical signs died within 24 hours of the onset of symptoms and all were found at death to have facial oedema, most pronounced in the submandibular area.
The recent report (Leendertz et al., 2004) of an outbreak of anthrax in chimpanzees in the Taï National Park, Côte d’Ivoire, is apparently the first record of naturally acquired anthrax in non-human primates. In experimentally induced inhalational anthrax in rhesus monkeys, irritability, weakness and anorexia 1–4 days before death were observed, the animals dying 3–8 days after exposure to the infecting spores (Friedlander et al., 1993).
It seems uncertain whether cold-blooded animals can contract anthrax. Greenfield (1880) stated that “frogs have been found capable of infection with the anthrax poison”. Snails, especially Arion fuscus, were claimed to be carriers by Karlinski (cited by Hutyra et al., 1946). This may represent passive carriage resulting from environmental contamination rather than true infection. As noted in section 1.1, Sterne (1959) stated that amphibians and reptiles are naturally resistant but warming of cold-blooded animals allows them to be experimentally infected; unfortunately he did not cite the relevant publication(s).
The large epizootic of anthrax in hippopotami in Zambia in 1988–1989 (Turnbull et al., 1991) was accompanied by a higher-than-usual mortality in crocodiles. However, these were not examined bacteriologically and the excess deaths were attributed by crocodile experts to gout from overeating (Turnbull, personal discussions, 1989). In the Chester Zoo outbreak in the United Kingdom (Lyon, 1973) affecting 13 carnivorous mammals, a western diamond-backed rattlesnake also died, but again was not examined to establish whether anthrax was the cause of death.
It is worth noting that in a number of endemic areas, such as in western Texas, various susceptible exotic species are now raised in semidomesticated environments for hunting. For example, in 2001 a large enzootic of anthrax occurred among domesticated white-tailed deer and exotic ungulate species in central Texas. Presumably further examples exist in Africa and probably other regions of the world where anthrax is endemic. These animals cannot be mass-vaccinated, and thus represent susceptible populations and foci of persisting disease in these regions (Yu et al., 2002).
3.5. Diagnosis
3.5.1. Blood smear rationale
In most species, at death from anthrax (the pig being a notable exception) the blood is usually teeming with the capsulated anthrax bacilli, provided the animal has not been treated. Numbers may also be lower in immunized animals that succumb to the disease (Turnbull, 1990b). Published figures for terminal B. anthracis blood counts are (approximate cfu/ml) mice 107, guinea-pigs 108–109, rats 104–107, sheep and goats 108, rhesus monkeys 104–109, chimpanzees 109, zebras 106–108, elephants 106–108, springbok 108, blue wildebeest 108, cheetah 108 (Lincoln et al., 1967; Turnbull, 1990b; Friedlander et al., 1993; Lindeque & Turnbull, 1994; Fritz et al., 1995; Lindeque et al., 1996a). In the occasional case, however, the terminal bacteraemia is unexpectedly low (Friedlander et al., 1993; Lindeque & Turnbull, 1994); this was actually recognized almost a century ago (Stockman, 1911).
Numbers of B. anthracis in the blood at death in pigs are invariably very low. This was recognized from very early on in the history of bacteriology (Stockman, 1911) and has been stated or confirmed frequently (Hudson, 1953; Sterne, 1959; Shaw et al., 1963; Edginton, 1990; Redmond et al., 1996a, 1997).
Apart from the cheetah (section 3.4.7), little relevant information relating to carnivores was found. Tubbesing (1997) was unable to visualize the capsulated bacilli in any of the blood smears from the two leopards or the two lions dead from anthrax on his farm (see section 3.4.7).
These figures are relevant to understanding the value of the blood smear for diagnosis. Lincoln et al. (1967) found that it became very difficult to observe B. anthracis in blood when numbers were below about 5 x 104 per ml.
3.5.2. Smear and culture
The blood characteristically clots poorly or not at all upon death from anthrax and is dark (sometimes described as “tarry”) and partially haemolysed. In a carcass that remains intact and unscavenged, it is usually easy to obtain the necessary drops of blood for smear and/or culture by means of a syringe from an appropriately accessible vein for about 24 hours after death. Alternatively, a dry swab inserted into a small incision in a region well supplied with blood vessels (the ear is traditionally recommended) can be used to make a smear and for culture (Annex 1, section 7.5). After fixing and staining as described in Annex 1, section.9.3, the capsulated bacilli may be looked for in the smears under a microscope. The haemorrhagic oronasal or anal exudate will also yield B. anthracis on culture, but selective isolation from other environmental contaminating bacteria may be necessary.
B. anthracis does not compete well with putrefactive bacteria and, with increasing age of the carcass, the capsulated bacilli become more difficult to visualize. Smears, as a diagnostic procedure, become unreliable about 24 hours after death, although capsular material may still be observed some time after the bacilli themselves can no longer be seen. Blood in transit at ambient temperatures for over 72 hours may not reveal organisms on examination or culture.
Where blood from a fresh carcass fails to reveal the presence of B. anthracis, but anthrax is still a possible or likely diagnosis, the capsulated bacilli will probably be visible in other body-fluid smears made from fine needle aspirates of affected areas, such as submandibular swellings, regional lymph nodes, mesenteric fluid, etc., or cultured from such specimens. This would be appropriate in pigs, for example, in which terminal bacteraemia is invariably low compared with most other species (see section 3.4.4).
Milk is not generally regarded as being useful for diagnostic purposes. There appear to be very few reports of isolation of B. anthracis from the milk of affected animals. Rare exceptions are instanced in Annex 6, section 3.
Regulations in most countries prohibit the opening and postmortem examination of animals where anthrax is the suspected cause of death. Contamination of the environment by spilled body fluids with subsequent spore formation is thereby avoided. In suspect cases, if negative results are obtained with the procedures described above, deeper examination of the cause of death may be necessary, “paying due regard to the possibility of anthrax existing and taking all reasonable precautions against infective material being disseminated” (Stockman, 1911). This could start with scalpel incision to obtain a smear or culture from a lymph node near the surface, progressing to more internal organs such as mesenteric lymph nodes or spleen.
If anthrax was not suspected and the carcass has been opened inadvertently, the dark unclotted blood and markedly enlarged haemorrhagic spleen will probably be immediately apparent. The mesentery may be thickened and oedematous and peritoneal fluid may be excessive. Petechial haemorrhages may be visible on many of the organs and the intestinal mucosa may be dark red and oedematous with areas of necrosis. The subcutaneous and intramuscular tissues may be oedematous. As indicated above, smears and cultures should be made from the mesenteric fluid and lymph nodes. Where anthrax has been diagnosed after a carcass has been opened, special attention should be paid to decontamination of the site at which the postmortem examination was carried out and of the tools and materials that were used (see Annex 3, sections 6 & 7).
When a carcass is old or putrefied, B. anthracis can often be cultured from residual skin or bloodstained material for some days after death, but this becomes progressively less easy the longer the time between death and examination. If the carcass has been opened and residual lymph node or spleen samples are available, these should be taken for culture. Diagnosis becomes increasingly dependent with time on isolation of spores from surface swabs of the remaining skeleton, particularly in the nostrils and eye sockets, or from soil or other environmental samples around or under the carcass that had been contaminated by the oronasal or anal exudates or spillages of body fluids.
3.5.3. Diagnosis in animals that have been treated before death
It may not be possible to find the bacilli in smears or to isolate B. anthracis from animals that were treated before death (see Annex 1, section 8.2); treatment can sterilize the blood and tissues but, if sufficient toxin has been formed, the animal may still die. Tests based on antigens, if available, may be the only practical approach (section 3.5.4). If it is sufficiently important to confirm the cause of death as anthrax, injection of spleen or lymph node extracts into mice or guinea-pigs may result in infection of the test animals by any residual viable B. anthracis (see Annex 1, section 12). Otherwise diagnosis may have to be made on the basis of the history and associated circumstances and findings (such as a positive feed sample that had been fed to the animal that died).
Immunohistochemical studies of fixed tissue or smears of aspirates have been shown to be a sensitive and specific method of diagnosis (Fritz et al., 1995; Guarner et al., 2003).
3.5.4. Diagnosis based on tests for antigens
The thermostable antigen precipitin test devised by Ascoli (1911) (see Annex 1, section 11.1) is still used in several countries of Europe and the Far East for detecting residual antigens in tissue in which it is no longer possible to demonstrate B. anthracis microscopically or by culture. However, it should be borne in mind that it is not a highly specific test; the antigens being detected are shared by other Bacillus species. The test relies on the fact that, if Bacillus antigens are present in the tissues, this probably represents B. anthracis infection since infections with other Bacillus species are rare. Care has to be taken if the tissue being examined has been grossly contaminated with environmental materials (soil, sand, etc.) which frequently harbour large numbers of other Bacillus species.
A simple, rapid and highly sensitive and specific chromatographic device, a more reliable and more sensitive alternative to the Ascoli test and utilizing a monoclonal capture antibody detecting the anthrax–specific protective antigen, has now been designed and shown to be useful for rapid on-site diagnosis in the field (Burans et al., 1996; Tubbesing, 1997; Muller et al., 2004) and not to give false reactions in recently vaccinated animals (Muller et al., 2004). Unfortunately, this has not become commercially available. These types of device are covered in section 6.2.
3.5.5. Molecular diagnosis
Genetically-based confirmation by PCR is becoming increasingly accepted on a stand-alone basis for many types of specimen and is increasingly available worldwide through commercial kits. It is still advisable to attempt to confirm bacteriologically (i) a positive to avoid the chance of a false positive, and (ii) a negative in case the PCR test was insufficiently sensitive.
3.5.6. Retrospective diagnosis (seroconversion)
Historically, there has been little need for serological support for the diagnosis of anthrax in animals. Either the animal had anthrax, recognized from the recent history of the herd or site, and was treated accordingly, or it died. Most of the interest in developing serological testing has been for research on humoral responses in humans, and – to a lesser extent – animals, to vaccines and for epidemiological studies involving naturally acquired seroconversion in humans, livestock and wild mammals.
Currently accepted as the best serological procedure is the ELISA in microtitre plates coated with the Protective Antigen (PA) and Lethal Factor (LF) components of the anthrax toxin. The toxin antigens appear to be truly specific for B. anthracis. PA and LF are available commercially3 but are costly. This tends to mean that anthrax serology is currently confined to a few specialist laboratories. Examples of the successful field application of anthrax serology in animals are given elsewhere (Turnbull et al., 1992a; Redmond et al., 1996a; 1997; Turnbull et al., 2001b, 2004b).
3.5.7. Differential diagnosis
For differential diagnosis, other causes of sudden death should be considered. Among these are African horse sickness, botulism, blackleg (Clostridium chauvoei), peracute babesiosis, chemical poisoning (heavy metals, other poisons), ingestion of toxic plants, snake bite, lightning strike or metabolic disorders such as lactic acidosis, magnesium deficiency, bloat. An outbreak of Rift Valley Fever in Kenya in 1997 was initially thought to be anthrax (WHO, 1997a). The differential diagnosis list will inevitably vary by species and geographical area, and the above list is not exhaustive.
Footnotes
- 1
This section summarizes information from different sources. Past and updated official information on disease occurrence is available through the OIE World Animal Health Information Database (WAHID) Interface: http://www
.oie.int/wahid-prod/public .php?page=home. - 2
Information from Dr P. Baptista, Department of Agriculture, South Rio Grande State, Brazil.
- 3
From www
.listlabs.com.
- Anthrax in animals - Anthrax in Humans and AnimalsAnthrax in animals - Anthrax in Humans and Animals
- PREDICTED: Talpa occidentalis uncharacterized LOC129145665 (LOC129145665), ncRNAPREDICTED: Talpa occidentalis uncharacterized LOC129145665 (LOC129145665), ncRNAgi|2470669352|ref|XR_008551584.1|Nucleotide
- Homo sapiens chromosome 3 open reading frame 34, mRNA (cDNA clone MGC:14126 IMAG...Homo sapiens chromosome 3 open reading frame 34, mRNA (cDNA clone MGC:14126 IMAGE:3533603), complete cdsgi|33871785|gb|BC007827.2|Nucleotide
- LOC127819058 [Homo sapiens]LOC127819058 [Homo sapiens]Gene ID:127819058Gene
Your browsing activity is empty.
Activity recording is turned off.
See more...