NCBI Bookshelf. A service of the National Library of Medicine, National Institutes of Health.
Madame Curie Bioscience Database [Internet]. Austin (TX): Landes Bioscience; 2000-2013.
Sphingolipids serve as potential reservoirs for bioactive lipids and are now included with the well-established mediators of signal transduction such as diacylglycerol, phosphatidylinositides, and eicosanoids, all derived from glycerolipids.16 Furthermore, sphingolipid metabolism has clearly been shown to be involved in the regulation of cell growth, differentiation, and programmed cell death.1–6 Indeed, there have been over 4000 studies conducted on sphingolipids during the past five years, and a majority have been directed towards understanding the emerging role of ceramide as a cellular bioregulator. Thus, with the demonstration that ceramide mediates various signaling cascades, there is now a necessity for defining direct targets of ceramide and specific mechanisms regulated by ceramide. The search for potential direct targets for ceramide action led to the identification of several candidate ceramide-regulated enzymes such as ceramide-activated protein kinases (CAPK), cathepsin D, and a key set of targets, serine/threonine protein phosphatases.7–12
Two serine/threonine phosphatases have been shown to be ceramide responsive in vitro and in vivo, protein phosphatase-1 (PP1) and protein phosphatase 2A (PP2A).10,11,13–16 These protein phosphatases are now collectively termed ceramide-activated protein phosphatases (CAPPs).This Chapter will discuss the relevant mechanisms by which CAPP is activated by ceramide in vitro and its emerging role in regulating various cascades of signal transduction through modulation of specific phospho-targets.
Identification of a Ceramide-Activated Protein Phosphatase (CAPP)
Based on biochemical parameters, Ser/Thr protein phosphatases were initially divided into two classes, type 1 protein phosphatases (PP1) and type 2 protein phosphatases (PP2A).17 The PP1 class is inhibited by two heat-stable proteins termed inhibitor 1 (I-1) and inhibitor 2 (I-2) whereas type 2 or PP2 class phosphatases are insensitive to these proteins, but inhibited by the small t-antigen of SV40.17–19 Type 2 phosphatases can be further subdivided into spontaneously active (PP2A), Ca+2-dependent (PP2B) and Mg+2-dependent (PP2C) classes.17,19 In the past few years, many novel serine/threonine phosphatases such as protein phosphatase-V and -X have been identified that do not fit into any of these classes.20
Both PP1 and PP2A are composed of a catalytic subunit bound by one or more regulatory subunits.17–19 These subunits regulate subcellular localization, substrate specificity, enzyme activity, and in some cases cofactor binding.17–19 The catalytic and regulatory subunits are also regulated by covalent modifications such as phosphorylation and methylation.18,19 Thus, regulation of these protein phosphatases is very complex requiring coordination of phosphorylation, methylation, subunit expression, and as discussed in this Chapter, lipid second messengers.
Originally, ceramide was shown to increase the activity of a serine/threonine protein phosphatase(s) in crude cytosolic extracts.10,11 This in vitro activation was achieved by D-erythro-ceramide, but not by the biologically inactive dihydro-ceramide.10,11 From this initial observation, a predominant ceramide-activated protein phosphatase was shown to exist in rat brain,21 and this phosphatase was identified as a member of the 2A (PP2A) family of serine/threonine phosphatases.10,11 This conclusion was based on studies demonstrating that pre-treatment with okadaic acid inhibited the ability of ceramide to activate a serine/threonine phosphatase in T9 glioma cell extracts.10,11 To conclusively demonstrate that PP2A is activated by ceramide, a novel chromatographic approach was utilized to purify the major CAPP activity from rat brain to near homogeneity.21 This approach used hydrophobic interaction chromatography on phenyl Sepharose followed by anion-exchange on MonoQ.21 CAPP purified in this manner was found to be composed of three major bands on SDS-PAGE which co-migrated with the three subunits of heterotrimeric PP2A.21 Immunological studies then identified CAPP as heterotrimeric (AB'C and ABαC) and heterodimeric (AC) PP2A species.21 Further demonstrating that PP2A was a form of CAPP, heterotrimeric PP2A (AB'C) and heterodimeric PP2A (AC) purified from rabbit skeletal muscle were shown to be activated by ceramide directly and in a specific manner.21 These results were further supported by the co-elution of CAPP with PP2A on size-exclusion chromatography and by their immunologic identity.21 These studies clearly demonstrated that PP2A was indeed a form of CAPP and provided a convenient and efficient method for isolating the PP2A species of CAPP to allow for in-depth biochemical analysis.21
Another eluting species of ceramide-activated phosphatases was also noted, and further studies showed that this was PP1, a closely-related serine/threonine phosphatase.13,14 In vitro studies showed that purified PP1 responds to ceramide in vitro with similar specificities as PP2A,13,14 thus establishing PP1 as a second CAPP.
In Vitro Regulation of CAPP by Ceramide
Several studies have now shown that short chain (cell permeable) ceramides activate PP2A and the catalytic subunit of PP1 (PP1c) in vitro.10,14,21,22 D-e-C2-ceramide activated heterotrimeric PP2A (AB'C) up to 3.5-fold with an EC50 of approximately 5 μM and to a lesser extent (up to 2.5-fold) the heterodimeric form (AC) of PP2A.10,21 Chain length studies on heterotrimeric PP2A demonstrated that ceramides possessing hexanoyl, decanoyl, and myristoyl, but not stearoyl acyl chains also activated the phosphatase.10,21
The longer chain ceramides failed to activate due to solubility problems in delivering those hydrophobic ceramides (see below). Activation of heterotrimeric PP2A was ceramide-specific, as closely related lipids had no effect on PP2A activity.10,21 Moreover, dihydro-C2-ceramide (which lacks the trans 4–5 double bond found in ceramide) did not activate PP2A in vitro, but actually inhibited PP2A activity.10,21 These findings were particularly important for several reasons. First, they demonstrated chain-length selectivity in the activation of PP2A. Second, dihydroceramide is a metabolic precursor of ceramide, thus establishing metabolic specificity of ceramide in activation of CAPP. Finally, dihydroceramides had been shown not to mimic the cellular activities of ceramide in inducing apoptosis, cell cycle arrest, or differentiation.23 Thus, these corresponding specificities in cellular action and in vitro activation of CAPP suggested CAPP as a relevant cellular target for ceramide.
To further demonstrate that this activation was specific, the stereospecificity of activation of these phosphatases by ceramides was examined. Thus, the stereoisomers of the naturally occurring D-erytho-C2 ceramide (2S, 3R) were synthesized. These were its enantiomer, L-erytho-C2 ceramide (2R, 3S), and the two diastereoisomers in the threo conformation, D-threo-C2 ceramide and L-threo-C2 ceramide, which differ from erythro in that the C-2 configuration relative to C-3 is in a cis-conformation unlike erythro in which the groups are in a trans-conformation. PP2A demonstrated some stereospecificity with the short chain ceramides in that only the D- and L-erythro forms of C2-ceramide activated PP2A and not the D- and L-threo forms.10,21
PP1 was also activated by short chain ceramides in vitro. In these studies, D-e-C6- and D-e-C2-ceramide activated PP1c in a dose-dependent manner (Kishikawa K, Chalfant CE, Lee JY, Hannun YA. 2001; unpublished findings). PP1 showed similar N-acyl chain length dependencies (as PP2A) since the N-acetyl, hexanoyl, and decanoyl, but not stearoyl ceramides activated PP1 in vitro (Kishikawa K, Chalfant CE, Lee JY, Hannun YA. 2001; unpublished findings). Similar to PP2A, the activation was specific as again closely related lipids as well as the inactive form, dihydro-C6-ceramide, failed to activate PP1 (Kishikawa K, Chalfant CE, Lee JY, Hannun YA. 2001; unpublished findings). PP1 also demonstrated stereospecificity with D-erythro-C6-ceramide activating to the greatest extent compared to its stereoisomers (Kishikawa K, Chalfant CE, Lee JY, Hannun YA. 2001; unpublished findings).
A major problem that hampered further evaluation of the biochemical regulation of CAPP in vitro had been the lack of an effect of long chain (natural) ceramides. Since long chain neutral lipids are insoluble in aqueous solutions, delivering long chain ceramides in vitro and in vivo is difficult and limited. To overcome this problem, long chain ceramides were solubilized with 2% dodecane at a final reaction concentration of 0.02%.13 Dodecane alone exerted an inhibitory effect on phosphatase activity, but solubilized D-e-C18 ceramide clearly activated the PP1α catalytic subunit (PP1αc), PP1γ catalytic subunit (PP1γc), PP2Ac, and PP2A trimer in a dose-dependent manner.13 This activation was examined for specificity by long chain ceramides using stereoisomers of D-e-C18 ceramide.13 Only D-e-C18 ceramide activated each phosphatase. These observations are significant for at least three reasons.13 First, activation of CAPP by natural ceramides was demonstrated. Second, specificity for ceramide was clearly demonstrated for the long chain ceramide, and the stereochemical specificity became better defined with the long chain isomers than with the short chain ones. Third, a specific ceramide-binding/interaction site was now suggested to be present on the catalytic subunit of at least PP1 and PP2A since they respond directly to ceramide. Since these observations, unsaturated natural ceramides dispersed in aqueous buffer by sonication have been shown to activate both PP1c and PP2Ac without the need for dodecane solubilization (Chalfant CE, Hannun YA. 2001; unpublished findings).
Selective CAPP Substrates in Cells
Since initial studies showed that the specificity for CAPP activation in vitro closely resembled the specificity for various cellular activities of ceramide such as apoptosis,5,10,11,13,14,24,25 CAPP emerged as a key candidate for mediating cellular activities of ceramide through dephosphorylation of key substrates. Several criteria for establishing a CAPP substrate are proposed: 1) exogenous ceramides induce the dephosphorylation of the particular phospho-substrate; 2) inhibitors of serine/threonine protein phosphatase block this effect; 3) exogenous dihydro-ceramide should not affect the phosphorylation state of the particular phospho-substrate; and 4) the target phospho-protein should act as an in vitro substrate for CAPP. Using these criteria, several targets for CAPP have been identified by multiple groups (Fig. 1).
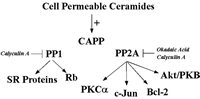
Figure 1
Schematic of known in vivo substrates of ceramide-activated protein phosphatases.
Protein Kinase Cα (PKCα)
The first study demonstrating a CAPP substrate in cells came in 1996 from Obeid and co-workers.16 They first observed that in Molt-4 leukemia cells, ceramide inhibited phorbol 12-myristate 13-acetate (PMA)-induced phosphorylation of many substrates, suggesting a role for ceramide in regulating the protein kinase C (PKC) pathway.16,26 PKCα is a key member of the PKC family that is involved in signal transduction, growth regulation, and metabolic regulation. Importantly, PKCα activity has been specifically implicated as an anti-apoptotic mechanism, and it has even been proposed that inactivation of PKCα is important in inducing apoptosis.26 Therefore, the mechanism by which ceramide regulated PKCα was pursued, and ceramide was found to inhibit basal and PMA-induced phosphorylation of PKCα (i.e., autophosphorylation).16 These results suggested that the effects of ceramide were at the level of regulation of PKC rather than its substrates. Ceramide did not directly inhibit PKCα in vitro nor did ceramide modulate the levels of PKCα protein, or inhibit PMA-induced translocation of PKCα.16 Using an immunoprecipitation assay for PKCα activity, treatment of Molt-4 cells with ceramide resulted in a concentration- and time-dependent inhibition of immunoprecipitated PKCα activity.16 The inactive form of ceramide, dihydro-C6 ceramide had no effect on PKCα activity.16 Ceramide was found to block the phosphorylation of PKCα in cells.16 Together, these results suggested that ceramide inactivated PKC by removing critical phosphates (such as thr 500 in the activation loop). Indeed, using an antibody specific for this site, it was shown that ceramide induces specifically the removal of this phosphate.27 Mechanistically (from the ceramide point of view), okadaic acid at concentrations that inhibit the serine/threonine protein phosphatase PP2A, blocked ceramide-induced dephosphorylation of PKCα in Molt-4 cells.16 These results indicated a direct role for ceramide and ceramide-activated protein phosphatase in mediating the in activation of PKCα in Molt-4 cells.
c-Jun
Also in 1996, Galarreta and co-workers identified c-Jun as a target for CAPP in cells.28 They demonstrated that treatment with exogenous ceramide or TNFα, a ceramide-generating agonist, led to time-dependent dephosphorylation of c-Jun in A431 cells.28 Furthermore and importantly, treatment of these cells with bacterial sphingomyelinase (B-SMase) also induced the dephosphorylation of c-Jun, demonstrating for the first time that endogenously generated ceramide (in this case, ceramide generated at the plasma membrane) induced the dephosphorylation of a CAPP target.28 Pre-treatment of these cells with okadaic acid completely blocked the effects of exogenous ceramide, TNFα, and bacterial SMase on c-Jun, suggesting a PP2A form of CAPP.28 Therefore, c-Jun was shown to be a substrate for CAPP in vitro fulfilling all of the criteria for the designation of a CAPP substrate.
Retinoblastoma Gene Product (Rb)
Because of the essential involvement of Rb in the G0/G1 phase of the cell cycle, the effects of ceramide on Rb were investigated. Rb was dephosphorylated, but not proteolytically cleaved, following ceramide treatment in Molt-4 cells overexpressing Bcl-2.11,29–31 Using this model, it was found that D-e-C6 ceramide induced a rapid (within 2-6 hours) dephosphorylation of Rb in a dose-dependent manner.11,29–31 In addition, the inactive form of C6-ceramide, dihydro-C6-ceramide, did not induce dephosphorylation of Rb demonstrating lipid specificity.11,29–31 Moreover, the PP1 and PP2A inhibitor, calyculin A, blocked ceramide-induced Rb dephosphorylation, suggesting a role for CAPP in regulating this mechanism.11,29–31 Of importance, okadaic acid at concentrations that specifically inhibit PP2A did not affect ceramide dephosphorylation of Rb, suggesting for the first time, a PP1-dependent mechanism.11,29–31 These results were further supported by the demonstration that Rb is a substrate for PP1 in vitro and ceramide increased the in vitro activity of PP1 toward Rb (Kishikawa K, Chalfant CE, Lee JY, Hannun YA. 2001; unpublished findings). Taken together, these results defined a mechanism by which ceramide regulates Rb dephosphorylation and suggested a different species of CAPP, PP1, responsible for imparting ceramide effects toward a specific in vivo target (Rb). Furthermore, these studies showed that okadaic acid and calyculin A inhibited distinct effects of ceramide, and implicated the action of different phosphatases.
Bcl-2
Recently, several key apoptotic factors have been shown to be regulated by phosphorylation/dephosphorylation via serine/threonine protein phosphatases. For example, Bcl-2 phosphorylation on serine 70 has been demonstrated to be necessary for its protective/survival function, and dephosphorylation of Bcl-2 serine 70 leads to BAD/Bcl-2 heterodimerization, effectively inhibiting the function of Bcl-2.32,33 Interestingly, serine 70 is a direct target for phosphorylation by PKCα and dephosphorylation by PP2A. Recently, May and co-workers demonstrated increased mitochondrial PP2A activity and specific dephosphorylation of mitochondrial Bcl-2 in response to ceramide.32,33 Again, okadaic acid was able to inhibit this effect, further implicating the PP2A form of CAPP in this ceramide effect.32,33 Thus, PP2A has been demonstrated to dephosphorylate both a Bcl-2 kinase (PKCα) and Bcl-2 in response to ceramide, suggesting a possible signaling complex. Furthermore, this report validated the signal transduction sequence for ceramide since other studies had placed ceramide formation somewhere between the action of upstream and downstream caspases, with Bcl-2 acting downstream of ceramide in that same pathway.34,35 Thus, ceramide regulates the phosphorylation of Bcl-2 and its anti-apoptotic function via CAPP, clearly placing ceramide generation upstream of Bcl-2 (Fig. 2).33–36
Akt/PKB
Multiple recent reports have implicated the protein kinase Akt (also known as PKB) as a target for ceramide action.37–41 Akt/PKB plays key roles in insulin action and in mitogenic and anti-apoptotic signaling.42,43 Akt/PKB is activated by phosphoinositides generated by PI-3 kinase and is also a substrate for PDK, an activating protein kinase, which is also activated by phosphoinositides.9,44,45 In turn, Akt/PKB has been shown to phosphorylate a number of key substrates including Bad, caspase 9, and others.9,43,44 Inactivation of Akt, a known PP2A substrate, has been shown to be necessary for activation of caspase 9 and Bad,9,44 and has been proposed as a necessary event in turning off a key anti-apopotic mechanism.
Importantly, several studies have demonstrated the ability of ceramide to induce dephosphorylation of Akt/PKB with concomitant loss of function/activity.9,37–41,45 These studies can be summarized as follows: First, treatment of mouse and rat skeletal muscle cells with exogenous ceramide has been demonstrated to inhibit Akt/PKB activity, and this effect was blocked by pre-treatment with okadaic acid.38,40–42 This implicated PP2A in regulating the phospho-state of Akt/PKB and, thus, its activity. Second, impairment of Akt/PKB function and insulin sensitivity in adipocytes corresponds with increased levels of ceramide.46,47 Third, ceramide has been shown to block the translocation of Akt/PKB to the membrane in response to such agonists as insulin and PDGF.39 Lastly, Akt/PKB has been shown to rescue adipocytes from apoptosis in response to insulin, and ceramide blocked this effect.46 These data all suggest that ceramide, in most cases through a ceramide-activated protein phosphatase, inactivates Akt/PKB.
SR Proteins
Recently, exogenous ceramide was demonstrated to induce the dephosphorylation of SR proteins, a family of serine/arginine (RS) domain containing proteins that regulate constitutive and alternative pre-mRNA processing.15,48–56 It was demonstrated that treatment of Jurkat cells with D-e-C6 ceramide produced a time- and dose-dependent decrease in the phosphorylation of all detectable SR protein species, SRp70, SRp55, SRp40, and SRp30.15 Treatment of Jurkat cells with the biologically inactive D-e-dihydroC6 ceramide had no effect on the phosphorylation state of SR proteins.15 Caspase inhibitors had also no effect on the dephosphorylation of SR proteins in response to exogenous ceramide, demonstrating that this effect was independent or upstream of caspase activation.15 As with Rb dephosphorylation, calyculin A, but not okadaic acid blocked ceramide-induced dephosphorylation of SR proteins, demonstrating a role for protein phosphatase-1.15 These results, therefore, demonstrated a specific effect of D-e-C6 ceramide on the dephosphorylation of SR proteins via activation of CAPP.
Taken together, all of these studies thus far are beginning to demonstrate the important effects of ceramide on the dephosphorylation of several important substrates linked to signaling cascades.
Role of Endogenous Ceramide in Regulating CAPP Activity
One of the main difficulties in validating CAPP in cells had been the absence of studies demonstrating a dependence on the generation of endogenous ceramide for the dephosphorylation of a particular phospho-protein. As discussed previously in the Chapter, B-SMase treatment was shown to induce activation of PP2A leading to the dephosphorylation of c-JUN, thus demonstrating the ability of endogenous ceramide to exert specific functions.28 Although this study showed the ability of endogenous ceramide to activate CAPP in cells, a physiological role for endogenous ceramide in regulating CAPP in cells was not established. In order to establish a role for CAPP in signal transduction, studies are needed that utilize relevant agonists (e.g., TNFα) and demonstrate a necessary role for the generation of ceramide to activate CAPP. Indeed, two such models of CAPP activation have been defined recently. First, fumonisin B1, and inhibitor of Co-A dependent ceramide synthase and the generation of de novo ceramide, was shown to block dephosphorylation of PKCα by protein phosphatase 2A in response to TNFα in L929 cells (Fig. 3).27 Second, the same inhibitor of de novo ceramide generation also blocked the dephosphorylation of SR proteins in response to Fas activation (Fig. 3).15 These two reports were milestones validating the existence of ceramide-activated protein phosphatase in cells and demonstrating the necessity of generating endogenous ceramide in order to activate CAPP. Furthermore, these studies demonstrate that de novo ceramide can activate both PP1 and PP2A forms of CAPP.
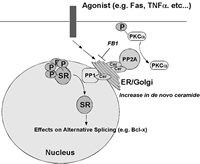
Figure 3
Schematic of serine/threonine protein phosphatases activated by de novo ceramide.
Now the question can be raised whether ceramide generated by other pathways can activate CAPP, only specific forms of CAPP, or even CAPPs localized in specific cellular compartments. Further studies are needed to answer these questions, but the identification of these ceramide-dependent mechanisms now also allows for the investigation of mechanisms downstream of CAPP.
Thus, the recent availability of specific inhibitors of pathways for generating ceramide in response to exogenous agonists and molecular tools (such as the overexpression of enzymes that clear ceramide, or antisense constructs for enzymes that generate ceramide), should now allow the modulation of ceramide levels, thereby, determining their effects on signaling events. Based on this, the criteria for defining a particular phospho-target as a substrate for CAPP should be expanded, and the designation as a CAPP substrate should now require the additional criterion of demonstrating that specific inhibition of ceramide generation leads to subsequent inhibition of the dephosphorylation of the phospho-substrate in question.
General Mechanisms Regulated by Ceramide-Activated Protein Phosphatases
Studies using specific inhibitors of serine/threonine protein phosphatases have demonstrated a clear role for CAPPs in regulating key biological responses. These studies and observations are briefly discussed below along with their implications.
Apoptosis
A role for serine-threonine protein phosphatases in regulating apoptosis has been established by many laboratories. Inhibitors of serine/threonine protein phosphatases have been demonstrated to block apoptosis induced by docosahexanoic acid, cytosine arabinoside, interleukin-3 withdrawal, heat stress, γ-radiation, and etoposide.40,46,57,58 Most of these apoptosis-inducers also cause an increase in ceramide levels, and several studies have specifically characterized a role for CAPPs in the apoptotic response. First, CAPP has been shown to be involved in c-Myc downregulation (a prelude to apoptosis in many cell models) in response to ceramide and TNFα.11 Second, CAPP has been shown to be involved in activation of caspases, and inhibition of CAPP blocks both apoptosis and caspase activation.59 Third, activation of CAPP has been shown to be involved in PC12 apoptosis and to block cell survival pathways mediated by nerve growth factor.40 Thus, many reports have shown both a correlation and a direct link between serine/threonine protein phosphatases, ceramide generation, and apoptosis in many cell models.
Differentiation
Two studies demonstrate a role for serine/threonine protein phosphatases in regulating neural cell differentiation. The first study demonstrated that both okadaic acid and calyculin A blocked the differentiation of Neuro 2a cells induced by lactacystin.60 In another study, Tettamanti and co-workers implicated CAPP in regulating the differentiation of Neura 2a cells.61,62 They showed that okadaic acid blocked Neura 2a cell differentiation under conditions that increased ceramide levels.61,62 This study clearly implicated the PP2A subspecies of CAPP and demonstrated a role for CAPP in mediating neural cell differentiation.
Cell Growth and Proliferation
Probably the most impressive body of work implicating CAPP in regulating specific cellular mechanisms is in the field of cell growth and proliferation. Many laboratories have established a role for CAPP genetically, biochemically, and at the level of pharmacology. Genetically, Nickels and Broach demonstrated a necessity for yeast CAPP in the induction of ceramide-mediated cell cycle arrest.25 Briefly, they demonstrated the PP2A yeast homologue was necessary for arresting yeast cells in the G1 phase and mutation of any of the heterotrimeric subunits which composed yeast PP2A rendered the cells resistant to ceramide-induced cell cycle arrest.25
In mammalian cells, we have already discussed the CAPP target, Rb, and dephosphorylation of Rb by CAPP has been shown to induce cell cycle arrest.29 Recently, the hypo-phosphorylated form of Rb has been shown to be an efficient substrate for caspase 3 unlike the phosphorylated form.63 Furthermore, cyclin-dependent kinase II (CDK2) has been recently shown as a substrate for CAPP.64 This study demonstrated that inhibitors of PP1 and PP2A block the inactivation of CDK2 in response to ceramide.64 Since inactivation of CDK2 has been demonstrated to induce the dephosphorylation of Rb, this illustrates another example of a signaling complex where CAPP both inactivates a kinase and its substrate through dephosphorylation.64
Insulin Secretion and Action
The role of CAPP in insulin signaling is becoming an emergent theme. First, ceramide and CAPP have been implicated in blocking insulin secretion and induction of apoptosis in isolated beta cells.65 This finding taken together with the knowledge that increased cytokines, specifically those that increase ceramide levels in cells (e.g., TNFα), were upregulated in diabetic patients led to a recent flood of reports on inhibition of insulin action in response to ceramide.66,67 In these reports, exogenous ceramide treatment was shown to block both insulin-stimulated glucose transport (ISGT) and insulin-stimulated glycogen synthesis (ISGS).38,39,41,42,45,46,68,69 Okadaic acid in both instances inhibited the effect of ceramide on ISGT and ISGS implicating the PP2A form of CAPP. Since okadaic acid has also been established as an inducer of ISGT, a role for PP2A inhibition (CAPP inhibition) has been hypothesized as a necessary mechanism in insulin responses to permit kinase cascades to proceed.70 As previously discussed, one role of CAPP, mechanistically, is the inhibition of Akt/PKB in response to ceramide.38,39,41,42,45,47,65,69
Thus, CAPP has been shown to play an integral role in regulating both insulin secretion and insulin responses, making it a possible target for the design of therapies to alleviate the diabetic condition.
Regulation of mRNA Processing as a Specific Pathway Regulated by CAPP
Through examination of possible downstream mechanisms regulated by ceramide-responsive enzymes, a common theme of substrates for both CAPP and PKCζ was recognized in the alternative processing of pre-mRNA. Both PP1 and PKCζ have been demonstrated to regulate protein factors involved in the mechanism of alternative splicing.15 Specifically, in response to endogenous ceramide, PP1 dephosphorylated SR proteins, a family of factors that regulate constitutive and alternative processing of pre-mRNA.15,49,51–54,71–73 Dephosphorylation of SR proteins effectively inhibits many of their functions and has been reported to regulate alternative processing of pre-mRNA.49,51–54,71–73 On the other hand, ceramide has been shown to induce PKCζ to phosphorylate hnRNPA1, a known regulator of the alternative processing of pre-mRNA and antagonist of SR proteins.7,74 Phosphorylation of hnRNP A1 induces its nuclear translocation and increases its RNA binding ability, thereby enhancing its function as an antagonist of SR proteins.7,74,75 Therefore, ceramide, via the “cross-talk” of two direct targets, effectively diminishes the ability of SR proteins to regulate pre-mRNA processing by inhibiting activity directly (dephosphorylation) and indirectly (activation of an SR protein antagonist). Thus, based on these observations, we propose that ceramide may regulate the alternative splicing of genes that play an important role in cellular stress responses and apoptosis.
Indeed, examination of the literature reveals that the both Bcl-x and caspase 9 genes produced splicing variants with opposing functions.76,77 The Bcl-x splice variant, Bcl-x(L) and the caspase 9 splice variant, caspase 9b, inhibit apoptosis in contrast to the pro-apoptotic splice variants, Bcl-x(s) and caspase 9.76,77 Ceramide was found to regulate the alternative splicing of both Bcl-x and caspase 9, reducing the expression of the cell survival factors, caspase 9b and Bcl-x(L) while increasing the expression of the pro-apoptotic factors, Bcl-x(s) and caspase 9.78 Ceramide-induced alternative splicing was blocked by inhibitors of serine-threonine protein phosphatases and of the de novo ceramide pathway, demonstrating a role for CAPP and endogenous ceramide in regulating this mechanism.78 These observations were important in that a novel, direct, and specific mechanism mediated by a ceramide-activated protein phosphatase and endogenous ceramide has now been defined.
It is now obvious that regulation of sphingolipid pathways plays an important and complex role in regulating many cellular responses. Through careful examination of ceramide signaling, the various cascades of ceramide action are now being delineated (Fig. 4). This conclusion increases the need for intense investigation of sphingolipid-mediated mechanisms and with the emergence of new molecular and biochemical tools for sphingolipid metabolic enzymes, new mechanisms and targets mediated by ceramide-activated protein phosphatases and other ceramide-responsive enzymes will soon be discovered.
Acknowledgments
This work was supported by a grant (to Y.A.H.) from the National Institutes of Health (CA87584 & GM43825), a National Research Service Award (to C.E.C.) from the National Institutes of Health (GM19953-02), and a VA Merit Review (to C.E.C.) from the Veteran's Administration.
References
- 1.
- Hannun YA, Linardic CM. Sphingolipid breakdown products: Anti-proliferative and tumor-suppressor lipids. Biochim Biophys Acta. 1993;1154:223–236. [PubMed: 8280742]
- 2.
- Merrill A H Jr, Wang E, Gilchrist DG, Riley RT. Fumonisins and other inhibitors of de novo sphingolipid biosynthesis. Adv Lipid Res. 1993;26:215–234. [PubMed: 8379451]
- 3.
- Spiegel S, Merrill A H Jr. Sphingolipid metabolism and cell growth regulation. FASEB J. 1996;10:1388–1397. [PubMed: 8903509]
- 4.
- Pinto WJ, Srinivasan B, Shepherd S, Schmidt A, Dickson RC, Lester RL. Sphingolipid long-chain-base auxotrophs of Saccharomyces cerevisiae: genetics, physiology, and a method for their selection. J Bacteriol. 1992;174:2565–2574. [PMC free article: PMC205895] [PubMed: 1556075]
- 5.
- Hannun YA. Functions of ceramide in coordinating cellular responses to stress. Science. 1996;274:1855–1859. [PubMed: 8943189]
- 6.
- Hanada K, Nishijima M, Kiso M. et al. Sphingolipids are essential for the growth of Chinese hamster ovary cells. Restoration of the growth of a mutant defective in sphingoid base biosynthesis by exogenous sphingolipids. J Biol Chem. 1992;267:23527–23533. [PubMed: 1429697]
- 7.
- Lozano J, Berra E, Municio MM. et al. Protein kinase C zeta isoform is critical for kappa B-dependent promoter activation by sphingomyelinase. J Biol Chem. 1994;269:19200–19202. [PubMed: 8034680]
- 8.
- Mathias S, Dressler KA, Kolesnick RN. Characterization of a ceramide-activated protein kinase: stimulation by tumor necrosis factor alpha. Proc Natl Acad Sci USA. 1991;88:10009–10013. [PMC free article: PMC52856] [PubMed: 1946418]
- 9.
- Basu S, Bayoumy S, Zhang Y, Lozano J, Kolesnick R. BAD enables ceramide to signal apoptosis via Ras and Raf-1. J Biol Chem. 1998;273:30419–30426. [PubMed: 9804808]
- 10.
- Dobrowsky RT, Kamibayashi C, Mumby MC, Hannun YA. Ceramide activates heterotrimeric protein phosphatase 2A. J Biol Chem. 1993;268:15523–15530. [PubMed: 8393446]
- 11.
- Wolff RA, Dobrowsky RT, Bielawska A, Obeid LM, Hannun YA. Role of ceramide-activated protein phosphatase in ceramide-mediated signal transduction. J Biol Chem. 1994;269:19605–19609. [PubMed: 8034729]
- 12.
- Heinrich M, Wickel M, Winoto-Morbach S. et al. Ceramide as an activator lipid of cathepsin D. Adv Exp Med Biol. 2000;477:305–315. [PubMed: 10849758]
- 13.
- Chalfant CE, Kishikawa K, Mumby MC, Kamibayashi C, Bielawska A, Hannun YA. Long chain ceramides activate protein phosphatase-1 and protein phosphatase-2A. Activation is stereospecific and regulated by phosphatidic acid. J Biol Chem. 1999;274:20313–20317. [PubMed: 10400653]
- 14.
- Kishikawa K, Chalfant CE, Perry DK, Bielawska A, Hannun YA. Phosphatidic acid is a potent and selective inhibitor of protein phosphatase 1 and an inhibitor of ceramide-mediated responses. J Biol Chem. 1999;274:21335–21341. [PubMed: 10409693]
- 15.
- Chalfant CE, Ogretmen B, Galadari SH, Kroesen B j B, Pettus BJ, Hannun YA. FAS activation induces dephosphorylation of SR proteins. Dependence on the de novo generation of ceramide and activation of protein phosphatase-1. J Biol Chem. 2001;276:44848–44855. [PubMed: 11502750]
- 16.
- Lee JY, Hannun YA, Obeid LM. Ceramide inactivates cellular protein kinase Calpha. J Biol Chem. 1996;271:13169–13174. [PubMed: 8662781]
- 17.
- Cohen P. Classification of protein-serine/threonine phosphatases: identification and quantitation in cell extracts. Methods Enzymol. 1991;201:389–398. [PubMed: 1658556]
- 18.
- Cohen P. The structure and regulation of protein phosphatases. Adv Second Messenger Phosphoprotein Res. 1990;24:230–235. [PubMed: 2169802]
- 19.
- Wera S, Hemmings BA. Serine/threonine protein phosphatases. Biochem J. 1995;311:17–29. [PMC free article: PMC1136113] [PubMed: 7575450]
- 20.
- Cohen PT, Brewis ND, Hughes V, Mann DJ. Protein serine/threonine phosphatases; An expanding family. FEBS Lett. 1990;268:355–359. [PubMed: 2166691]
- 21.
- Galadari S, Kishikawa K, Kamibayashi C, Mumby MC, Hannun YA. Purification and characterization of ceramide-activated protein phosphatases. Biochemistry. 1998;37:11232–11238. [PubMed: 9698369]
- 22.
- Law B, Rossie S. The dimeric and catalytic subunit forms of protein phosphatase 2A from rat brain are stimulated by C2-ceramide. J Biol Chem. 1995;270:12808–12813. [PubMed: 7759536]
- 23.
- Bielawska A, Crane HM, Liotta D, Obeid LM, Hannun YA. Selectivity of ceramide-mediated biology. Lack of activity of erythro-dihydroceramide. J Biol Chem. 1993;268:26226–26232. [PubMed: 8253743]
- 24.
- Fishbein JD, Dobrowsky RT, Bielawska A, Garrett S, Hannun YA. Ceramide-mediated growth inhibition and CAPP are conserved in Saccharomyces cerevisiae. J Biol Chem. 1993;268:9255–9261. [PubMed: 8387486]
- 25.
- Nickels JT, Broach JR. A ceramide-activated protein phosphatase mediates ceramide-induced G1 arrest of Saccharomyces cerevisiae. Genes Dev. 1996;10:382–394. [PubMed: 8600023]
- 26.
- Gamard CJ, Dbaibo GS, Liu B, Obeid LM, Hannun YA. Selective involvement of ceramide in cytokine-induced apoptosis. Ceramide inhibits phorbol ester activation of nuclear factor kappaB. J Biol Chem. 1997;272:16474–16481. [PubMed: 9195956]
- 27.
- Lee JY, Hannun YA, Obeid LM. Functional dichotomy of protein kinase C (PKC) in tumor necrosis factor-alpha (TNF-alpha ) signal transduction in L929 cells. Translocation and inactivation of PKC by TNF-alpha. J Biol Chem. 2000;275:29290–29298. [PubMed: 10887171]
- 28.
- Reyes JG, Robayna IG, Delgado PS. et al. c-Jun is a downstream target for ceramide-activated protein phosphatase in A431 cells. J Biol Chem. 1996;271:21375–21380. [PubMed: 8702918]
- 29.
- Dbaibo GS, Pushkareva MY, Jayadev S. et al. Retinoblastoma gene product as a downstream target for a ceramide- dependent pathway of growth arrest. Proc Natl Acad Sci USA. 1995;92:1347–1351. [PMC free article: PMC42516] [PubMed: 7877980]
- 30.
- Obeid LM, Linardic CM, Karolak LA, Hannun YA. Programmed cell death induced by ceramide. Science. 1993;259:1769–1771. [PubMed: 8456305]
- 31.
- Chao R, Khan W, Hannun YA. Retinoblastoma protein dephosphorylation induced by D-erythro-sphingosine. J Biol Chem. 1992;267:23459–23462. [PubMed: 1385423]
- 32.
- Ruvolo PP, Deng X, Ito T, Carr BK, May WS. Ceramide induces Bcl2 dephosphorylation via a mechanism involving mitochondrial PP2A. J Biol Chem. 1999;274:20296–20300. [PubMed: 10400650]
- 33.
- Ruvolo PP, Deng X, Carr BK, May WS. A functional role for mitochondrial protein kinase Calpha in Bcl2 phosphorylation and suppression of apoptosis. J Biol Chem. 1998;273:25436–25442. [PubMed: 9738012]
- 34.
- Smyth MJ, Perry DK, Zhang J, Poirier GG, Hannun YA, Obeid LM. prIce: A downstream target for ceramide-induced apoptosis and for the inhibitory action of Bcl-2. Biochem J. 1996;316:25–28. [PMC free article: PMC1217330] [PubMed: 8645213]
- 35.
- Zhang J, Alter N, Reed JC, Borner C, Obeid LM, Hannun YA. Bcl-2 interrupts the ceramide-mediated pathway of cell death. Proc Natl Acad Sci USA. 1996;93:5325–5328. [PMC free article: PMC39244] [PubMed: 8643573]
- 36.
- Ruvolo PP, Deng X, Ito T, Carr BK, May WS. Ceramide induces Bcl2 dephosphorylation via a mechanism involving mitochondrial PP2A. J Biol Chem. 1999;274:20296–20300. [PubMed: 10400650]
- 37.
- Chen D, Fucini RV, Olson AL, Hemmings BA, Pessin JE. Osmotic shock inhibits insulin signaling by maintaining Akt/protein kinase B in an inactive dephosphorylated state. Mol Cell Biol. 1999;19:4684–4694. [PMC free article: PMC84266] [PubMed: 10373517]
- 38.
- Cazzolli R, Carpenter L, Biden TJ, Schmitz-Peiffer C. A role for protein phosphatase 2a-like activity, but not atypical protein kinase c zeta, in the inhibition of protein kinase b/akt and glycogen synthesis by palmitate. Diabetes. 2001;50:2210–2218. [PubMed: 11574400]
- 39.
- Stratford S, DeWald DB, Summers SA. Ceramide dissociates 3'-phosphoinositide production from pleckstrin homology domain translocation. Biochem J. 2001;354:359–368. [PMC free article: PMC1221664] [PubMed: 11171115]
- 40.
- Salinas M, Lopez-Valdaliso R, Martin D, Alvarez A, Cuadrado A. Inhibition of PKB/Akt1 by C2-ceramide involves activation of ceramide-activated protein phosphatase in PC12 cells. Mol Cell Neurosci. 2000;15:156–169. [PubMed: 10673324]
- 41.
- Schmitz-Peiffer C, Craig DL, Biden TJ. Ceramide generation is sufficient to account for the inhibition of the insulin-stimulated PKB pathway in C2C12 skeletal muscle cells pretreated with palmitate. J Biol Chem. 1999;274:24202–24210. [PubMed: 10446195]
- 42.
- Hajduch E, Litherland GJ, Hundal HS. Protein kinase B (PKB/Akt)A key regulator of glucose transport? FEBS Lett. 2001;492:199–203. [PubMed: 11257494]
- 43.
- Kim DS, Kim SY, Moon SJ. et al. Ceramide inhibits cell proliferation through Akt/PKB inactivation and decreases melanin synthesis in Mel-Ab cells. Pigment Cell Res. 2001;14:110–115. [PubMed: 11310790]
- 44.
- Cardone MH, Roy N, Stennicke HR. et al. Regulation of cell death protease caspase-9 by phosphorylation. Science. 1998;282:1318–1321. [PubMed: 9812896]
- 45.
- Wick MJ, Dong LQ, Riojas RA, Ramos FJ, Liu F. Mechanism of phosphorylation of protein kinase B/Akt by a constitutively active 3-phosphoinositide-dependent protein kinase-1. J Biol Chem. 2000;275:40400–40406. [PubMed: 11006271]
- 46.
- Navarro P, Valverde AM, Rohn JL, Benito M, Lorenzo M. Akt mediates insulin rescue from apoptosis in brown adipocytes: effect of ceramide. Growth Horm IGF Res. 2000;10:256–266. [PubMed: 11042022]
- 47.
- Summers SA, Garza LA, Zhou H, Birnbaum MJ. Regulation of insulin-stimulated glucose transporter GLUT4 translocation and Akt kinase activity by ceramide. Mol Cell Biol. 1998;18:5457–5464. [PMC free article: PMC109130] [PubMed: 9710629]
- 48.
- Du K, Peng Y, Greenbaum LE, Haber BA, Taub R. HRS/SRp40-mediated inclusion of the fibronectin EIIIB exon, a possible cause of increased EIIIB expression in proliferating liver. Mol Cell Biol. 1997;17:4096–4104. [PMC free article: PMC232263] [PubMed: 9199345]
- 49.
- Caceres JF, Misteli T, Screaton GR, Spector DL, Krainer AR. Role of the modular domains of SR proteins in subnuclear localization and alternative splicing specificity. J Cell Biol. 1997;138:225–238. [PMC free article: PMC2138183] [PubMed: 9230067]
- 50.
- Zahler AM. Purification of SR protein splicing factors. Methods Mol Biol. 1999;118:419–432. [PubMed: 10549540]
- 51.
- Zahler AM, Neugebauer KM, Lane WS, Roth MB. Distinct functions of SR proteins in alternative pre-mRNA splicing. Science. 1993;260:219–222. [PubMed: 8385799]
- 52.
- Zahler AM, Lane WS, Stolk JA, Roth MB. SR proteins: A conserved family of pre-mRNA splicing factors. Genes Dev. 1992;6:837–847. [PubMed: 1577277]
- 53.
- Utz PJ, Hottelet M, van Venrooij WJ, Anderson P. Association of phosphorylated serine/arginine (SR) splicing factors with the U1-small ribonucleoprotein (snRNP) autoantigen complex accompanies apoptotic cell death. J Exp Med. 1998;187:547–560. [PMC free article: PMC2212144] [PubMed: 9463405]
- 54.
- Manley JL, Tacke R. SR proteins and splicing control. Genes Dev. 1996;10:1569–1579. [PubMed: 8682289]
- 55.
- Lopato S, Mayeda A, Krainer AR, Barta A. Pre-mRNA splicing in plants: Characterization of Ser/Arg splicing factors. Proc Natl Acad Sci USA. 1996;93:3074–3079. [PMC free article: PMC39763] [PubMed: 8610170]
- 56.
- Lin CH, Patton JG. Regulation of alternative 3' splice site selection by constitutive splicing factors. RNA. 1995;1:234–245. [PMC free article: PMC1369077] [PubMed: 7489496]
- 57.
- Marushige K, Marushige Y. Modulation of cell rounding and apoptosis in trigeminal neurinoma cells by protein phosphatase inhibitors. Anticancer Res. 1998;18:295–300. [PubMed: 9568093]
- 58.
- Siddiqui RA, Jenski LJ, Neff K, Harvey K, Kovacs RJ, Stillwell W. Docosahexaenoic acid induces apoptosis in Jurkat cells by a protein phosphatase-mediated process. Biochim Biophys Acta. 2001;1499:265–275. [PubMed: 11341974]
- 59.
- Morana SJ, Wolf CM, Li J, Reynolds JE, Brown MK, Eastman A. The involvement of protein phosphatases in the activation of ICE/CED-3 protease, intracellular acidification, DNA digestion, and apoptosis. J Biol Chem. 1996;271:18263–18271. [PubMed: 8663484]
- 60.
- Tanaka H, Katagiri M, Arima S, Matsuzaki K, Inokoshi J, Omura S. Neuronal differentiation of Neuro 2a cells by lactacystin and its partial inhibition by the protein phosphatase inhibitors calyculin A and okadaic acid. Biochem Biophys Res Commun. 1995;216:291–297. [PubMed: 7488103]
- 61.
- Prinetti A, Bassi R, Riboni L, Tettamanti G. Involvement of a ceramide activated protein phosphatase in the differentiation of neuroblastoma Neuro2a cells. FEBS Lett. 1997;414:475–479. [PubMed: 9315744]
- 62.
- Riboni L, Prinetti A, Bassi R, Caminiti A, Tettamanti G. A mediator role of ceramide in the regulation of neuroblastoma Neuro2a cell differentiation. J Biol Chem. 1995;270:26868–26875. [PubMed: 7592930]
- 63.
- Wang RH, Liu CW, Avramis VI, Berndt N. Protein phosphatase 1alpha-mediated stimulation of apoptosis is associated with dephosphorylation of the retinoblastoma protein. Oncogene. 2001;20:6111–6122. [PubMed: 11593419]
- 64.
- Lee JY, Bielawska AE, Obeid LM. Regulation of cyclin-dependent kinase 2 activity by ceramide. Exp Cell Res. 2000;261:303–311. [PubMed: 11112337]
- 65.
- Kowluru A, Metz SA. Ceramide-activated protein phosphatase-2A activity in insulin-secreting cells. FEBS Lett. 1997;418:179–182. [PubMed: 9414122]
- 66.
- Mishima Y, Kuyama A, Tada A, Takahashi K, Ishioka T, Kibata M. Relationship between serum tumor necrosis factor-alpha and insulin resistance in obese men with Type 2 diabetes mellitus. Diabetes Res Clin Pract. 2001;52:119–123. [PubMed: 11311966]
- 67.
- Cavallo MG, Pozzilli P, Bird C. et al. Cytokines in sera from insulin-dependent diabetic patients at diagnosis. Clin Exp Immunol. 1991;86:256–259. [PMC free article: PMC1554128] [PubMed: 1934594]
- 68.
- Begum N, Ragolia L. Effect of tumor necrosis factor-alpha on insulin action in cultured rat skeletal muscle cells. Endocrinology. 1996;137:2441–2446. [PubMed: 8641197]
- 69.
- Hajduch E, Balendran A, Batty IH. et al. Ceramide impairs the insulin-dependent membrane recruitment of protein kinase B leading to a loss in downstream signalling in L6 skeletal muscle cells. Diabetologia. 2001;44:173–183. [PubMed: 11270673]
- 70.
- Standaert ML, Bandyopadhyay G, Sajan MP, Cong L, Quon MJ, Farese RV. Okadaic acid activates atypical protein kinase C (zeta/lambda) in rat and 3T3/L1 adipocytes. An apparent requirement for activation of Glut4 translocation and glucose transport. J Biol Chem. 1999;274:14074–14078. [PubMed: 10318822]
- 71.
- Mermoud JE, Cohen PT, Lamond AI. Regulation of mammalian spliceosome assembly by a protein phosphorylation mechanism. Embo J. 1994;13:5679–5688. [PMC free article: PMC395533] [PubMed: 7988565]
- 72.
- Du C, McGuffin ME, Dauwalder B, Rabinow L, Mattox W. Protein phosphorylation plays an essential role in the regulation of alternative splicing and sex determination in Drosophila. Mol Cell. 1998;2:741–750. [PubMed: 9885562]
- 73.
- Misteli T, Spector DL. Serine/threonine phosphatase 1 modulates the subnuclear distribution of pre-mRNA splicing factors. Mol Biol Cell. 1996;7:1559–1572. [PMC free article: PMC276006] [PubMed: 8898362]
- 74.
- Municio MM, Lozano J, Sanchez P, Moscat J, Diaz-Meco MT. Identification of heterogeneous ribonucleoprotein A1 as a novel substrate for protein kinase C zeta. J Biol Chem. 1995;270:15884–15891. [PubMed: 7541049]
- 75.
- van der Houven van Oordt W, Diaz-Meco MT, Lozano J, Krainer AR, Moscat J, Caceres JF. The MKK(3/6)-p38-signaling cascade alters the subcellular distribution of hnRNP A1 and modulates alternative splicing regulation. J Cell Biol. 2000;149:307–316. [PMC free article: PMC2175157] [PubMed: 10769024]
- 76.
- Minn AJ, Boise LH, Thompson CB. Bcl-x(S) anatagonizes the protective effects of Bcl-x(L). J Biol Chem. 1996;271:6306–6312. [PubMed: 8626425]
- 77.
- Srinivasula SM, Ahmad M, Guo Y. et al. Identification of an endogenous dominant-negative short isoform of caspase-9 that can regulate apoptosis. Cancer Res. 1999;59:999–1002. [PubMed: 10070954]
- 78.
- Chalfant CE, Rathman K, Pinkerman RL. et al. De novo ceramide regulates the alternative splicing of caspase 9 and Bcl-x in A549 lung adenocarcinoma cells. Dependence on protein phosphatase-1. J Biol Chem. 2002;277:12587–12595. [PubMed: 11801602]
- Identification of a Ceramide-Activated Protein Phosphatase (CAPP)
- In Vitro Regulation of CAPP by Ceramide
- Selective CAPP Substrates in Cells
- Role of Endogenous Ceramide in Regulating CAPP Activity
- General Mechanisms Regulated by Ceramide-Activated Protein Phosphatases
- Regulation of mRNA Processing as a Specific Pathway Regulated by CAPP
- Acknowledgments
- References
- The Role of Serine/Threonine Protein Phosphatases in Ceramide Signaling - Madame...The Role of Serine/Threonine Protein Phosphatases in Ceramide Signaling - Madame Curie Bioscience Database
- Target Recognition of Guanylate Cyclase by Guanylate Cyclase-Activating Proteins...Target Recognition of Guanylate Cyclase by Guanylate Cyclase-Activating Proteins - Madame Curie Bioscience Database
- Vascular Endothelial Growth Factor - Madame Curie Bioscience DatabaseVascular Endothelial Growth Factor - Madame Curie Bioscience Database
- The Biology of Telomeres in Hypotrichous Ciliates - Madame Curie Bioscience Data...The Biology of Telomeres in Hypotrichous Ciliates - Madame Curie Bioscience Database
- Homo sapiens small EDRK-rich factor 1A (SERF1A), transcript variant 1, mRNAHomo sapiens small EDRK-rich factor 1A (SERF1A), transcript variant 1, mRNAgi|1890270737|ref|NM_021967.4|Nucleotide
Your browsing activity is empty.
Activity recording is turned off.
See more...