Summary
Clinical characteristics.
In Alport syndrome (AS) a spectrum of phenotypes ranging from progressive renal disease with extrarenal abnormalities to isolated hematuria with a non-progressive or very slowly progressive course is observed. Approximately two thirds of AS is X-linked (XLAS); approximately 15% is autosomal recessive (ARAS), and approximately 20% is autosomal dominant (ADAS). In the absence of treatment, renal disease progresses from microscopic hematuria (microhematuria) to proteinuria, progressive renal insufficiency, and end-stage renal disease (ESRD) in all males with XLAS, and in all males and females with ARAS. Progressive sensorineural hearing loss (SNHL) is usually present by late childhood or early adolescence. Ocular findings include anterior lenticonus (which is virtually pathognomonic), maculopathy (whitish or yellowish flecks or granulations in the perimacular region), corneal endothelial vesicles (posterior polymorphous dystrophy), and recurrent corneal erosion. In individuals with ADAS, ESRD is frequently delayed until later adulthood, SNHL is relatively late in onset, and ocular involvement is rare.
Diagnosis/testing.
The diagnosis of Alport syndrome is established in a proband with a pathogenic variant(s) in COL4A3, COL4A4, or COL4A5 identified on molecular genetic testing.
Management.
Treatment of manifestations: Angiotensin-converting enzyme inhibitor or angiotensin receptor blocker to delay onset of ESRD; routine treatment of hypertension; renal transplantation for ESRD. Potential living related donors must be evaluated carefully to avoid nephrectomy in an affected individual. Routine treatment of SNHL and cataracts; surgical intervention for symptomatic leiomyomas.
Agents/circumstances to avoid: Protection of corneas from minor trauma in those with recurrent corneal erosions. Minimize exposure to loud noise.
Surveillance: Follow up of all individuals with Alport syndrome with a nephrologist every six to 12 months; monthly monitoring of at-risk transplant recipients for development of anti-glomerular basement membrane antibody-mediated glomerulonephritis for the first year post transplant; audiologic evaluation of children every one to two years beginning at age six to seven years; monitoring for ocular abnormalities; evaluation for aortic dilatation (for males with XLAS).
Evaluation of relatives at risk: Evaluate at-risk family members either by urinalysis or, if the pathogenic variant(s) in the family are known, by molecular genetic testing.
Genetic counseling.
Three modes of inheritance are recognized for Alport syndrome: X-linked, autosomal recessive, and autosomal dominant.
- In families with X-linked inheritance, mothers heterozygous for a COL4A5 pathogenic variant have a 50% chance of transmitting the pathogenic variant in each pregnancy; sons who inherit the pathogenic variant will be affected with Alport syndrome and will eventually develop ESRD and, in most cases, deafness; daughters who inherit the pathogenic variant will typically have asymptomatic hematuria but may have more severe renal disease. Affected males will pass the pathogenic variant to all of their daughters and none of their sons.
- In families with autosomal recessive inheritance, the parents of an affected child are obligate heterozygotes and carry one pathogenic variant; at conception, each sib of an affected individual has a 25% chance of being affected, a 50% chance of being a carrier who may or may not be symptomatic, and a 25% chance of being unaffected and not a carrier.
- In families with autosomal dominant inheritance, each child of an affected individual has a 50% chance of inheriting the pathogenic variant and being affected.
- In rare Alport families with digenic inheritance (pathogenic variants in two or more of the COL4A3, COL4A4, and COL4A5 genes), transmission patterns may not conform to mendelian expectations.
Molecular genetic testing for at-risk family members, prenatal testing for a pregnancy at increased risk, and preimplantation genetic testing are possible if the pathogenic variant(s) in the family are known.
Diagnosis
Alport syndrome is a familial renal disorder caused by pathogenic variants in COL4A3, COL4A4, and COL4A5 that result in abnormalities of the collagen IV α345 network of basement membranes [Kashtan et al 2018]. Alport syndrome can be transmitted in an X-linked (XLAS), autosomal dominant (ADAS), or autosomal recessive (ARAS) pattern. The spectrum of renal involvement ranges from isolated, non-progressive hematuria to progressive nephropathy characterized by hematuria, proteinuria, and chronic kidney disease (CKD) and end-stage renal disease (ESRD). Affected individuals often have sensorineural hearing loss and characteristic ocular abnormalities. Rare individuals have associated aortic disease or diffuse leiomyomatosis.
This definition differs from previous iterations in that it classifies individuals with isolated hematuria and heterozygous pathogenic variants in COL4A3 and COL4A4 as having ADAS, rather than so-called "thin basement membrane nephropathy." For the argument in favor of this approach see Kashtan et al [2018]; for the counterargument see Savige [2018]. The goals of this approach are:
- To encourage establishment of specific diagnoses in individuals with persistent glomerular hematuria with molecular confirmation whenever possible;
- To facilitate surveillance of individuals with pathogenic variants in COL4A3, COL4A4, and COL4A5 for evidence of renal disease progression;
- To promote early initiation of therapy to delay or prevent ESRD; and
- To improve identification of affected family members.
Suggestive Findings
Alport syndrome should be suspected in an individual with the following clinical and pedigree features [Kashtan 2004].
Renal
- Hematuria. 100% of affected males and more than 90% of affected females with XLAS have microhematuria (blood in the urine detectable only by microscope); 100% of males and females with ARAS have hematuria. Episodic gross hematuria is not unusual. Most individuals with ADAS have hematuria, although it may be intermittent.
- Proteinuria, hypertension, and renal insufficiency develop with advancing age in all males with XLAS and in all males and females with ARAS. Proteinuria is frequent in ADAS, especially with advancing age, and affected individuals may exhibit progressive renal disease. Pathogenic variants in COL4 genes are not unusual in adults with proteinuria and renal biopsy findings of focal segmental glomerulosclerosis.
Cochlear
- Bilateral high-frequency sensorineural hearing loss (SNHL) typically becomes apparent by audiometry in late childhood or early adolescence in males with XLAS and in males and females with ARAS.
- In XLAS, SNHL eventually develops in 80%-90% of affected males as well as in some affected females; the incidence of SNHL in males and females with ARAS is probably similar to that in males with XLAS.
- In some families with XLAS and in ADAS, SNHL may not be detectable until well into adulthood.
Ocular
- Anterior lenticonus is pathognomonic of Alport syndrome. It occurs in 15%-20% of those with XLAS or ARAS and typically becomes apparent in late adolescence or early adulthood.
- Perimacular flecks occur in approximately 30% of individuals with Alport syndrome.
Family history
- Family history positive for hematuria, deafness, and/or ESRD is frequently seen.
- Family history of hematuria or renal failure may be negative because 10%-15% of males with XLAS have the disorder as the result of a de novo pathogenic variant and approximately 15% of affected individuals have ARAS.
Testing
Urinalysis
Males with XLAS; males and females with ARAS. Urinalysis typically shows hematuria (dozens to hundreds of erythrocytes per high-power microscope field). Overlap with normal results is minimal. Proteinuria develops in essentially 100% of affected individuals, although the timing is variable.
Female heterozygotes for XLAS. Approximately 95% of heterozygotes exhibit persistent or intermittent microhematuria. Proteinuria develops in approximately 75% of heterozygous females.
Both males and females with XLAS may have intermittent or constant gross hematuria.
Both males and females with ADAS. Urinalysis frequently shows microhematuria although some individuals are asymptomatic. A substantial proportion of affected individuals eventually develop proteinuria.
Establishing the Diagnosis
The diagnosis of Alport syndrome is established in a proband with a pathogenic (or likely pathogenic) variant(s) in COL4A3, COL4A4, or COL4A5 identified on molecular genetic testing. See Table 1.
Note: (1) Per ACMG/AMP variant interpretation guidelines, the terms "pathogenic variants" and "likely pathogenic variants" are synonymous in a clinical setting, meaning that both are considered diagnostic and both can be used for clinical decision making [Richards et al 2015]. Reference to "pathogenic variants" in this section is understood to include any likely pathogenic variants. (2) Identification of variant(s) of uncertain significance cannot be used to confirm or rule out the diagnosis.
Molecular genetic testing approaches can include a combination of gene-targeted testing (multigene panel) and comprehensive genomic testing (exome sequencing, exome array, genome sequencing) depending on the phenotype.
Gene-targeted testing requires that the clinician determine which gene(s) are likely involved, whereas genomic testing does not. Because the phenotype of Alport syndrome is broad, individuals with the distinctive findings described in Suggestive Findings are likely to be diagnosed using gene-targeted testing (see Option 1), whereas those with atypical features in whom the diagnosis of Alport syndrome has not been considered are more likely to be diagnosed using genomic testing (see Option 2).
Option 1
When the phenotypic and laboratory findings suggest the diagnosis of Alport syndrome, the recommended molecular genetic testing approach is the use of a multigene panel.
A multigene panel that includes COL4A3, COL4A4, COL4A5, and other genes of interest (see Differential Diagnosis) is most likely to identify the genetic cause of the condition while limiting identification of variants of uncertain significance and pathogenic variants in genes that do not explain the underlying phenotype. Note: (1) The genes included in the panel and the diagnostic sensitivity of the testing used for each gene vary by laboratory and are likely to change over time. (2) Some multigene panels may include genes not associated with the condition discussed in this GeneReview. (3) In some laboratories, panel options may include a custom laboratory-designed panel and/or custom phenotype-focused exome analysis that includes genes specified by the clinician. (4) Methods used in a panel may include sequence analysis, deletion/duplication analysis, and/or other non-sequencing-based tests.
For this disorder a multigene panel that also includes deletion/duplication analysis is recommended (see Table 1).
For an introduction to multigene panels click here. More detailed information for clinicians ordering genetic tests can be found here.
Note: Some laboratories may offer targeted analysis for pathogenic variants, which may vary by laboratory. The pathogenic variants particularly common in the United States include the following:
Option 2
When the diagnosis of Alport syndrome is not considered because an individual has atypical phenotypic features, comprehensive genomic testing (which does not require the clinician to determine which gene[s] are likely involved) is the best option. Exome sequencing is most commonly used; genome sequencing is also possible. Exome array (when clinically available) may be considered if exome sequencing is not diagnostic. For an introduction to comprehensive genomic testing click here. More detailed information for clinicians ordering genomic testing can be found here.
Note: Pathogenic variants in COL4A3 and COL4A4 can be heterozygous (autosomal dominant) or biallelic (autosomal recessive). Pathogenic variants in COL4A5 are hemizygous or heterozygous (X-linked).
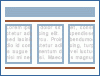
Table 1.
Molecular Genetic Testing Used in Alport syndrome (AS)
Digenic inheritance. Mencarelli et al [2015] have described digenic inheritance of Alport syndrome in several families. The causative allelic variants may be in cis or in trans, and result in variable clinical features and transmission patterns.
Clinical Characteristics
Clinical Description
In Alport syndrome a spectrum of phenotypes ranging from progressive renal disease with extrarenal abnormalities to isolated hematuria with a nonprogressive or very slowly progressive course is observed.
Alport syndrome has renal and, frequently, cochlear and ocular manifestations. Approximately two thirds of Alport syndrome is X-linked (XLAS); approximately 15% is autosomal recessive (ARAS), and approximately 20% is autosomal dominant (ADAS). In the X-linked form of Alport syndrome, disease manifestations are typically more severe in affected males. However, affected females with either XLAS or ARAS may have severe involvement as well. ADAS is typically a slowly progressive disorder; renal insufficiency and sensorineural hearing loss (SNHL) may not develop until relatively late in life.
Renal Manifestations
The hallmark of Alport syndrome is microscopic hematuria (microhematuria). Males with XLAS have persistent microhematuria from early in life. Episodic gross hematuria can occur, especially during childhood. More than 90% of females with XLAS have microhematuria, although it may be intermittent. Individuals with ARAS have persistent microhematuria; males and females are equally affected. Individuals with a heterozygous COL4A3 or COL4A4 pathogenic variant associated with ARAS have an estimated 50% incidence of persistent or intermittent microhematuria.
All males with XLAS develop proteinuria and, eventually, progressive renal insufficiency, which leads to end-stage renal disease (ESRD). Overall, an estimated 60% reach ESRD by age 30 years, and 90% by age 40 years [Jais et al 2000]. The rate of progression to ESRD is influenced by the nature of the COL4A5 pathogenic variant (see Genotype-Phenotype Correlations).
Approximately 12% of females with XLAS develop ESRD before age 40 years, increasing to 30% by age 60 years and 40% by age 80 years [Jais et al 2003].
Most individuals with ARAS develop significant proteinuria in late childhood or early adolescence and ESRD before age 30 years. Progression to ESRD occurs at a slower pace in individuals with ADAS (frequently delayed until later adulthood) than in those with XLAS or ARAS.
Renal Biopsy
Immunohistochemical analysis
- Males with XLAS typically show complete absence of immunostaining for the collagen α3(IV) chain, α4(IV) chain, and α5(IV) chain on renal biopsy.Approximately 20% of males with XLAS show normal staining of renal basement membranes for the collagen α3(IV) chain, α4(IV) chain, and α5(IV) chain.
- Females heterozygous for XLAS typically exhibit patchy loss of staining for the collagen α3, α4, and α5(IV) chains in GBMs and tubular basement membranes [Kashtan et al 1996]. Some heterozygous females exhibit normal staining for the collagen α3, α4, and α5(IV) chains in renal basement membranes.
- Individuals with ARAS show abnormalities of renal type IV collagen expression that differ from those of individuals with X-linked disease. Individuals with ARAS typically exhibit complete absence of staining for the collagen α3(IV) chain and α4(IV) chain. However, whereas their GBMs show no staining for the collagen α5(IV) chain, staining of Bowman's capsules and tubular basement membranes for the collagen α5(IV) chain is positive [Gubler et al 1995]. Some individuals with ARAS exhibit normal renal basement membrane staining for the collagen α3(IV) chain, α4(IV) chain, and α5(IV) chain.
- Individuals with ADAS exhibit normal GBM staining for the collagen α3(IV) chain, α4(IV) chain, and α5(IV) chain.
Electron microscopy
- Normal. The normal glomerular capillary wall has a trilaminar appearance consisting of a homogeneous electron-dense layer (lamina densa) sandwiched between two electron-lucent layers (the laminæ rara interna and externa).The outer (subepithelial) aspect of the glomerular capillary wall, where it abuts the foot processes of the glomerular visceral epithelial cells, is smooth and regular.A variety of techniques have been used to measure GBM width. The cutoff value in adults ranges from 250 nm to 330 nm, depending on technique. The cutoff value in children ranges from 200 nm to 250 nm, depending on technique (250 nm is within 2 SD of the mean at age 11 years).
- Alport syndrome. When diffusely present, the following three alterations are pathognomonic of Alport syndrome:
- The lamina densa appears to be split into multiple interlacing strands of electron-dense material, resembling basket weaving.
- The lacunæ between these strands are frequently occupied by round, electron-dense bodies (possibly entrapped cytoplasm).
- The glomerular capillary wall is diffusely thickened and its epithelial aspect is scalloped.
However, the earliest change in Alport syndrome is diffuse thinning of the GBM. Children with XLAS and ARAS frequently exhibit only GBM thinning on renal biopsy. Women with XLAS and individuals with ADAS also may exhibit only GBM thinning. Marked variability in GBM width within a glomerulus in an individual with persistent microhematuria should raise suspicion of Alport syndrome.
Skin Biopsy
When renal biopsy is contraindicated (and genetic testing is not possible), a skin biopsy could be performed in place of the renal biopsy with the following findings:
- Males with XLAS. In about 80% of males, incubation of a skin biopsy specimen with a monoclonal antibody directed against the collagen α5(IV) chain shows complete absence of staining of epidermal basement membranes. Approximately 20% of males show normal staining.
- Females heterozygous for XLAS. Approximately 60%-70% of heterozygous females exhibit discontinuous staining of the collagen α5(IV) chain [van der Loop et al 1999]. This is attributed to X-chromosome inactivation, by which it would be expected that one half of the basilar keratinocytes would express a normal collagen α5(IV) chain.
- Individuals with ARAS. All individuals have normal skin reactivity for the collagen α5(IV) chain.
- Individuals with ADAS. All individuals have normal skin reactivity for the collagen α5(IV) chain.
Cochlear Manifestations
Hearing loss in Alport syndrome is never congenital. Diminished hearing is frequently detectable by late childhood or early adolescence in boys with XLAS. In its early stages, the hearing deficit is detectable only by audiometry, with bilateral reduction in sensitivity to tones in the 2,000- to 8,000-Hz range. In affected males, the hearing loss is progressive and eventually extends to other frequencies, including those of conversational speech. Hearing loss is frequently identifiable by formal assessment of hearing in late childhood, but in some families is not detectable until relatively late in life.
SNHL develops in 80%-90% of males with XLAS by age 40 years [Jais et al 2000]. The course of the hearing loss depends on the pathogenic variant (see Genotype-Phenotype Correlations). Hearing impairment in members of families with Alport syndrome is always accompanied by evidence of renal involvement; no convincing evidence that deaf males lacking renal disease can transmit Alport syndrome to their offspring has been reported. In females with XLAS, hearing loss is less frequent and tends to occur later in life.
There do not appear to be differences between males and females in the incidence or course of hearing loss in ARAS. Individuals with ARAS typically exhibit juvenile onset of hearing loss. Hearing loss may be a very late development in individuals with ADAS.
A histologic study of cochleas in individuals with Alport syndrome suggests that defective adhesion of the organ of Corti to the basilar membrane may underlie the hearing deficit [Merchant et al 2004].
Ocular Manifestations
Ocular lesions are common in Alport syndrome, occurring in 30%-40% of individuals with XLAS. The spectrum of ocular lesions appears to be similar in XLAS and ARAS. Ocular lesions appear to be relatively uncommon in ADAS.
- Anterior lenticonus, in which the central portion of the lens protrudes into the anterior chamber, is virtually pathognomonic in Alport syndrome. When present, anterior lenticonus is bilateral in approximately 75% of individuals. It is absent at birth, usually appearing during the second to third decade of life. Progressive distortion of the lens may occur, accompanied by increasing myopia. Lens opacities may be seen in conjunction with lenticonus, occasionally resulting from rupture of the anterior lens capsule.All reported individuals with anterior lenticonus who have been adequately examined have exhibited evidence of chronic nephritis and sensorineural hearing loss. It is far more common in affected males but can occur in females. The frequency of lenticonus in males with XLAS was 13% in one large series; its occurrence is related to the pathogenic variant (see Genotype-Phenotype Correlations).
- Maculopathy consisting of whitish or yellowish flecks or granulations in the perimacular region was found in approximately 14% of males with XLAS in a large series. While the maculopathy is usually not associated with any visual abnormalities, some individuals have developed macular holes associated with severe thinning of the retina.
- Corneal endothelial vesicles (posterior polymorphous dystrophy) and recurrent corneal erosion may also be seen in individuals with Alport syndrome.
- Bilateral posterior subcapsular cataracts also occur frequently in individuals with Alport syndrome with diffuse leiomyomatosis (see Other).
Other
Aneurysms of the thoracic and abdominal aorta have been described in a small number of males with Alport syndrome [Lyons et al 2007, Kashtan et al 2010]. These aneurysms are notable for the relatively early age of diagnosis (age <40 years) and have required surgical intervention.
Diffuse leiomyomatosis. The association of Alport syndrome with diffuse leiomyomatosis of the esophagus and tracheobronchial tree has been reported in several dozen families [Antignac & Heidet 1996, Mothes et al 2002]. This results from large deletions that span the adjacent 5' ends of COL4A5 and COL4A6 [Zhou et al 1993] (see Genotype-Phenotype Correlations). Symptoms usually appear in late childhood and include dysphagia, postprandial vomiting, retrosternal or epigastric pain, recurrent bronchitis, dyspnea, cough, and stridor. Affected females in these kindreds typically exhibit genital leiomyomas as well, causing clitoral hypertrophy with variable involvement of the labia majora and uterus.
Genotype-Phenotype Correlations – XLAS
Risk for end-stage renal disease (in untreated individuals)
- Large rearrangements and pathogenic nonsense and frameshift variants confer a 90% probability of end-stage renal disease (ESRD) before age 30 years, with 50% reaching ESRD by age 20 years [Jais et al 2000].
- In affected individuals with splice site variants, the probability of ESRD before age 30 years is 70%, with 50% reaching ESRD by age 25 years.
- Missense variants are associated with only a 50% probability of ESRD before age 30 years and a renal half-life (the time it takes for 50% of the group to reach end stage; i.e., the need for dialysis or transplantation) of 32 years.
- In one study, COL4A5 missense variants that alter glycine and non-glycine residues in the 3' portion of COL4A5 were associated with earlier development of ESRD, compared with missense variants that alter glycine residues in the 5' portion of the gene [Gross et al 2002]. In another study, pathogenic variants at the 5' end of COL4A5 were associated with earlier ESRD, hearing loss, and ocular changes [Bekheirnia et al 2010].
Risk for deafness
- In individuals with large rearrangements of COL4A5 or nonsense, frameshift, or splice site variants, the risk for deafness is 50% at age ten years.
- In individuals with missense variants, the risk for deafness does not reach 50% until age 20 years.
Risk for anterior lenticonus. Anterior lenticonus occurs in approximately 15% of males with XLAS. Anterior lenticonus is almost entirely restricted to families with Alport syndrome with hearing loss and progression to ESRD before age 30 years. This observation is explained by the finding that lenticonus is significantly more common in individuals with a COL4A5 deletion or a small pathogenic variant resulting in a premature stop codon than in those with pathogenic missense or splice site variants [Jais et al 2000].
Risk for diffuse leiomyomatosis
- All families in which XLAS cosegregates with diffuse leiomyomatosis exhibit large deletions that span the adjacent 5' ends of COL4A5 and COL4A6. These deletions involve varying lengths of COL4A5, but the COL4A6 breakpoint is always located in the second intron of the gene [Antignac & Heidet 1996].
- Leiomyomatosis does not occur in individuals with deletions of COL4A5 and COL4A6 that extend beyond intron 2 of COL4A6.
- Pathogenic variants of COL4A6 alone do not appear to cause Alport syndrome, a finding consistent with the absence of the α6(IV) chain from normal GBM.
Penetrance
Penetrance may be decreased in ADAS; at present, data are insufficient to draw a definitive conclusion.
Nomenclature
The term "benign familial hematuria," previously applied to Alport syndrome, is a misnomer that should no longer be used.
Prevalence
The prevalence of Alport syndrome has been estimated at 1:50,000 live births [Levy & Feingold 2000]. Data from several series suggest that approximately one fifth of children evaluated by pediatric nephrologists for isolated microhematuria receive a diagnosis of Alport syndrome. According to the United States Renal Data System (USRDS), approximately 0.2% of adults and 3% of children in the United States with ESRD carry a diagnosis of Alport syndrome.
Genetically Related (Allelic) Disorders
AMME complex (Alport syndrome, intellectual disability [mental retardation], midface hypoplasia, and elliptocytosis; OMIM 300194) was described in two brothers shown to have a microdeletion of Xq22.3 involving all of COL4A5 and extending beyond its 3' end.
Focal segmental glomerulosclerosis. Pathogenic variants in COL4A3, COL4A4, or COLA5 are not unusual in adults with proteinuria and renal biopsy findings of focal segmental glomerulosclerosis [Gast et al 2016].
Differential Diagnosis
Conditions with Hematuria
Alport syndrome most often presents in childhood, and thus must be differentiated from other causes of persistent (>6 months in duration) microhematuria in children.
- The first step in the evaluation of hematuria in children is to attempt to establish the source of the hematuria (renal/glomerular, renal/post-glomerular, post-renal).
- This often involves evaluation of urinary erythrocyte morphology by phase contrast microscopy, urinary calcium measurement, and/or renal ultrasound examination.
- Family history is an important component of the initial evaluation of the child with hematuria. When possible, obtaining urinalyses on first-degree relatives can also be informative.
- Once microhematuria is provisionally localized to the glomeruli, possible etiologies include a number of chronic glomerulopathies.In the child with no known family history of hematuria, the most likely diagnoses are IgA nephropathy, Alport syndrome, and C3 glomerulopathy).
Combined Nephritis and Deafness
MYH9-related disorders. MYH9-related disorders (MYH9RD) are characterized by large platelets and thrombocytopenia, both of which are present from birth. MYH9RD is variably associated with young-adult onset of progressive sensorineural hearing loss, presenile cataract, elevation of liver enzymes, and renal disease manifesting initially as glomerular nephropathy. Before identification of pathogenic variants in MYH9, individuals with MYH9RD were diagnosed as having Epstein syndrome, Fechtner syndrome, May-Hegglin anomaly, or Sebastian syndrome based on the combination of different clinical findings at the time of diagnosis. Some individuals with MYH9RD exhibit ultrastructural changes of the glomerular capillary wall reminiscent of those seen in individuals with Alport syndrome. MYH9RD is inherited in an autosomal dominant manner.
Management
Evaluations Following Initial Diagnosis
To establish the extent of disease and needs in an individual diagnosed with Alport syndrome, the evaluations summarized in Table 2 (if not performed as part of the evaluation that led to the diagnosis) are recommended.
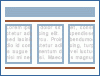
Table 2.
Recommended Evaluations Following Initial Diagnosis in Individuals with Alport Syndrome
Treatment of Manifestations
Clinical practice recommendations for the treatment of individuals with Alport syndrome have been published [Kashtan et al 2013]. These recommendations encourage early detection of microalbuminuria and proteinuria through regular surveillance and early intervention aimed at suppressing proteinuria using angiotensin antagonists (click here for full text).
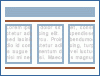
Table 3.
Treatment of Manifestations in Individuals with Alport Syndrome
Note on selection of kidney donors. The following discussion considers potential donors on the basis of their sex and the presence or absence of hematuria:
- Male relative without hematuria. The optimal living related donor is a male who has a normal urinalysis and is therefore unaffected.
- Male relative with hematuria. A related male with hematuria probably has Alport syndrome and cannot be a kidney donor.
- Female relative without hematuria. Female relatives who have normal urinalyses are probably unaffected, although approximately 5% of females heterozygous for a COL4A5 pathogenic variant are asymptomatic. It is rare for asymptomatic females whose children are also asymptomatic to be heterozygous. Asymptomatic females can probably donate a kidney safely but should be informed of their risk of having affected male children to whom they will not be able to donate a kidney. Genetic status can be confirmed or excluded by molecular genetic testing if the pathogenic variant in the family is known.
- Female relative with hematuria (i.e., heterozygous female). Females heterozygous for a COL4A5 pathogenic variant should only be considered as potential kidney donors if no asymptomatic living donors are available.
- The presence of proteinuria or sensorineural deafness is an absolute contraindication to kidney donation as these are risk factors for progression to ESRD.
- Heterozygous females younger than age 40 years should not be used as donors, even in the absence of proteinuria or deafness.
- Heterozygous females age 40 years or older who have normal renal function, blood pressure, and hearing and no proteinuria can be considered as donors because the risk of late progression to ESRD appears to be low in such individuals. However, the donor should be informed of the risk (albeit low) of late progression to ESRD.
Surveillance
Clinical practice recommendations for the treatment and health surveillance of individuals with Alport syndrome have been published [Kashtan et al 2013].
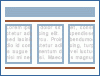
Table 4.
Recommended Surveillance for Individuals with Alport Syndrome
Agents/Circumstances to Avoid
Individuals who suffer recurrent corneal erosions may need to take measures (e.g., wearing goggles when riding a bicycle) to protect their corneas from minor trauma.
Exposure to loud noise should be minimized.
Evaluation of Relatives at Risk
It is appropriate to evaluate family members at risk for Alport syndrome in order to identify as early as possible those who would benefit from initiation of treatment and preventive measures. Evaluations can include:
- Molecular genetic testing if the pathogenic variant(s) in the family are known. Molecular genetic testing is necessary to exclude heterozygosity in an at-risk individual who does not have hematuria.
- Urinalysis and blood pressure determination:
- In males, a single urinalysis is sufficient.
- In females, several urinalyses should be performed before concluding that hematuria is absent.
Relatives found to have proteinuria, hypertension, and/or a pathogenic variant should be referred to a nephrologist for further evaluation. In the absence of proteinuria or hypertension, relatives at risk should, at a minimum, have an annual urinalysis and measurement of blood pressure if molecular genetic testing is not available.
See Genetic Counseling for issues related to testing of at-risk relatives for genetic counseling purposes.
Pregnancy Management
Women with Alport syndrome are at risk for pregnancy complications including increased proteinuria, renal insufficiency, worsened hypertension, and preeclampsia. The risks of these complications are higher in women with preexisting renal insufficiency, proteinuria, or hypertension. Optimal maternal and fetal outcomes may require the involvement of a nephrologist as well as high-risk obstetrics.
Therapies Under Investigation
Search ClinicalTrials.gov in the US and EU Clinical Trials Register in Europe for access to information on clinical studies for a wide range of diseases and conditions. Note: There may not be clinical trials for this disorder.
Genetic Counseling
Genetic counseling is the process of providing individuals and families with information on the nature, mode(s) of inheritance, and implications of genetic disorders to help them make informed medical and personal decisions. The following section deals with genetic risk assessment and the use of family history and genetic testing to clarify genetic status for family members; it is not meant to address all personal, cultural, or ethical issues that may arise or to substitute for consultation with a genetics professional. —ED.
Mode of Inheritance
Alport syndrome caused by pathogenic variants in COL4A5 is inherited in an X-linked manner (XLAS).
Alport syndrome caused by pathogenic variants in COL4A3 or COL4A4 can be inherited in an autosomal recessive (ARAS) or autosomal dominant manner (ADAS).
X-Linked Inheritance – Risk to Family Members
Parents of a male proband
- The father of a male with XLAS will not have the disease nor will he be hemizygous for the COL4A5 pathogenic variant; therefore, he does not require further evaluation/testing.
- In a family with more than one affected individual, the mother of an affected male is an obligate heterozygote. Note: If a woman has more than one affected child and no other affected relatives and if the COL4A5 pathogenic variant cannot be detected in her leukocyte DNA, she most likely has germline mosaicism.
- If a male is the only affected family member (i.e., a simplex case), the likelihood that that individual's mother is heterozygous is 85%-90%. Alternatively, the affected male may have a de novo COL4A5 pathogenic variant, in which case the mother is not heterozygous; approximately 10%-15% of male probands have XLAS as the result of a de novo pathogenic variant.
- Recommended evaluations for the mother of a male known to have XLAS include the following:
- Urinalysis. The presence of microhematuria indicates that she is likely to be heterozygous for a COL4A5 pathogenic variant. If proteinuria or hypertension is present, she should be referred to a nephrologist for further evaluation.
- Molecular genetic testing is necessary to exclude the heterozygous state in an at-risk female who does not have hematuria or hypertension. (Note: Molecular genetic testing requires prior identification of the pathogenic variant in the proband.)
- If the mother of an affected male is not found to have proteinuria or hypertension and if molecular genetic testing is not possible, she should have, at a minimum, an annual urinalysis and measurement of blood pressure.
Parents of a female proband
- The father or the mother of a female proband may have the COL4A5 pathogenic variant, or the proband may have a de novo pathogenic variant.
- Evaluation of parents of a female proband with XLAS begins with urinalysis and proceeds (for both parents) as described for the mother of a male proband.
Sibs of a male proband. The risk to the sibs of a male proband depends on the genetic status of the mother:
- If the mother of the proband has a COL4A5 pathogenic variant, the chance of transmitting it in each pregnancy is 50%. Males who inherit the pathogenic variant will be affected; females who inherit the pathogenic variant may or may not be symptomatic.
- If the mother is not heterozygous, the recurrence risk to sibs is slightly greater than that of the general population (though still <1%) because of the possibility of maternal germline mosaicism [Beicht et al 2013, Okamoto et al 2019].
Sibs of a female proband. The risk to the sibs of a female proband depends on the genetic status of the parents:
- If the mother of the proband has a COL4A5 pathogenic variant, the chance of transmitting it in each pregnancy is 50%. Males who inherit the pathogenic variant will be affected; females who inherit the pathogenic variant may or may not be symptomatic.
- If the father of the proband has a COL4A5 pathogenic variant, he will transmit it to all of his daughters and none of his sons.
- If the proband represents a simplex case (i.e., a single occurrence in a family) and if the COL4A5 pathogenic variant cannot be detected in the leukocyte DNA of either parent, the risk to sibs is slightly greater than that of the general population (though still <1%) because of the possibility of parental germline mosaicism.
Offspring of a proband
- Affected males transmit the COL4A5 pathogenic variant to all of their daughters (who may or may not be symptomatic) and none of their sons.
- Heterozygous females have a 50% chance of transmitting the pathogenic variant to each child: sons who inherit the COL4A5 pathogenic variant will be affected; daughters will have a range of possible phenotypic expression.
Other family members. The male proband's maternal aunts may be at risk of being heterozygous and the aunts' offspring, depending on their sex, may be at risk of being heterozygous for the pathogenic variant or being affected.
Heterozygote detection. Molecular genetic testing for at-risk females who lack microhematuria requires prior identification of the COL4A5 pathogenic variant in the family.
Note: (1) Females are heterozygotes for this X-linked disorder and may develop clinical findings related to the disorder. (2) Identification of female heterozygotes requires either (a) prior identification of the pathogenic variant in the family or, (b) if an affected male is not available for testing, molecular genetic testing first by sequence analysis, and then, if a pathogenic variant is not identified, by methods to detect gross structural abnormalities.
Autosomal Recessive Inheritance – Risk to Family Members
Parents of a proband
- The parents of an affected child are obligate heterozygotes (i.e., carriers of one COL4A3 or COL4A4 pathogenic variant).
- Approximately 50% of heterozygotes exhibit persistent or intermittent microhematuria.
Sibs of a proband
- At conception, each sib of an affected individual has a 25% chance of being affected, a 50% chance of being a heterozygote, and a 25% chance of being unaffected and not a heterozygote.
- Approximately 50% of heterozygotes exhibit persistent or intermittent microhematuria.
Offspring of a proband. The offspring of an individual with ARAS are obligate heterozygotes for a COL4A3 or COL4A4 pathogenic variant.
Other family members. Each sib of the proband's parents is at a 50% risk of being heterozygous for a COL4A3 or COL4A4 pathogenic variant.
Heterozygote detection. Genetic testing of at-risk relatives is possible if the COL4A3 or COL4A4 pathogenic variants have been identified in the family.
Autosomal Dominant Inheritance – Risk to Family Members
Parents of a proband
- Most individuals diagnosed with ADAS have an affected parent.
- A proband with ADAS may have the disorder as the result of a de novo COL4A3 or COL4A4 pathogenic variant. The proportion of cases caused by de novo pathogenic variants is unknown.
- Urinalysis is recommended for the evaluation of parents of a proband with an apparent de novo pathogenic variant and/or molecular genetic testing if the pathogenic variant has been identified in the proband.
- If the pathogenic variant found in the proband cannot be detected in the leukocyte DNA of either parent, possible explanations include a de novo pathogenic variant in the proband or germline mosaicism in a parent. Though theoretically possible, no instances of germline mosaicism have been reported.
- Note: Although most individuals diagnosed with ADAS have an affected parent, the family history may appear to be negative because of failure to recognize the disorder in family members, early death of the parent before the onset of symptoms, or late onset of the disease in the affected parent. Therefore, an apparently negative family history cannot be confirmed unless appropriate clinical evaluation and/or molecular genetic testing has been performed on the parents of the proband.
Sibs of a proband. The risk to the sibs of a proband depends on the clinical/genetic status of the proband's parents:
- If a parent of the proband is affected and/or is known to have the pathogenic variant identified in the proband, the risk to sibs is 50%.
- If the proband has a known COL4A3 or COL4A4 pathogenic variant that cannot be detected in the leukocyte DNA of either parent, the recurrence risk to sibs is estimated to be 1% because of the theoretic possibility of parental germline mosaicism [Rahbari et al 2016].
- If the parents have not been tested for the pathogenic variant identified in the proband but are clinically unaffected, the risk to the sibs of a proband appears to be low. However, sibs of a proband with clinically unaffected parents are still presumed to be at increased risk for ADAS because of the possibility of reduced penetrance in a parent or the theoretic possibility of parental germline mosaicism.
Offspring of a proband. Offspring of an individual with ADAS are at a 50% risk of inheriting the COL4A3 or COL4A4 pathogenic variant.
Other family members. The risk to other family members depends on the status of the proband's parents: if a parent is affected or has a COL4A3 or COL4A4 pathogenic variant, the parent's family members are at risk.
Related Genetic Counseling Issues
See Management, Evaluation of Relatives at Risk for information on evaluating at-risk relatives for the purpose of early diagnosis and treatment.
Screening of potential living related kidney donors. Most individuals with Alport syndrome who require kidney transplantation have X-linked disease. Both hemizygous males and heterozygous females with XLAS are at risk for ESRD. Potential living related donors must be evaluated carefully to avoid nephrectomy in an affected individual. Kashtan [2006] discusses in detail the evaluation of potential donors.
Family planning
- The optimal time for determination of genetic risk and discussion of the availability of prenatal/preimplantation genetic testing is before pregnancy.
- X-linked or autosomal recessive inheritance. It is appropriate to offer genetic counseling (including discussion of potential risks to offspring and reproductive options) to young adults who are affected, are heterozygous, or are at risk of being heterozygous.
- Autosomal dominant inheritance. It is appropriate to offer genetic counseling (including discussion of potential risks to offspring and reproductive options) to young adults who are affected or at risk.
DNA banking. Because it is likely that testing methodology and our understanding of genes, pathogenic mechanisms, and diseases will improve in the future, consideration should be given to banking DNA from probands in whom a molecular diagnosis has not been confirmed (i.e., the causative pathogenic mechanism is unknown). For more information, see Huang et al [2022].
Prenatal Testing and Preimplantation Genetic Testing
Once the COL4A3, COL4A4, or COL4A5 pathogenic variant(s) have been identified in an affected family member, prenatal testing for a pregnancy at increased risk and preimplantation genetic testing are possible.
Differences in perspective may exist among medical professionals and in families regarding the use of prenatal testing. While most centers would consider use of prenatal testing to be a personal decision, discussion of these issues may be helpful.
Resources
GeneReviews staff has selected the following disease-specific and/or umbrella support organizations and/or registries for the benefit of individuals with this disorder and their families. GeneReviews is not responsible for the information provided by other organizations. For information on selection criteria, click here.
- Alport Syndrome FoundationPhone: 480-800-3510Email: info@alportsyndrome.org
- MedlinePlus
- NCBI Genes and Disease
- Kidney Foundation of CanadaCanadaPhone: 514-369-4806Email: info@kidney.ca
- National Association of the DeafPhone: 301-587-1788 (Purple/ZVRS); 301-328-1443 (Sorenson); 301-338-6380 (Convo)Fax: 301-587-1791Email: nad.info@nad.org
- National Kidney FoundationPhone: 855-NKF-CARES; 855-653-2273Email: nkfcares@kidney.org
- Alport Syndrome Treatments and Outcomes Registry (ASTOR)University of Minnesota, Department of PediatricsEmail: alport@umn.edu
Molecular Genetics
Information in the Molecular Genetics and OMIM tables may differ from that elsewhere in the GeneReview: tables may contain more recent information. —ED.
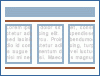
Table A.
Alport Syndrome: Genes and Databases
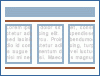
Table B.
OMIM Entries for Alport Syndrome (View All in OMIM)
Molecular Pathogenesis
Basement membranes. Basement membranes, the sheet-like structures that support epithelial and endothelial cells, are composed of several major and minor glycoproteins. Collagen IV is present ubiquitously in basement membranes, where it is the major collagenous component. Collagen IV molecules secreted by endothelial and epithelial cells self-associate into polygonal networks, which interact with laminin networks, as well as with nidogens, proteoglycans, and other glycoproteins, to form basement membranes.
Collagen IV. Collagen IV α chains share basic structural features and show extensive sequence homology. The major structural features of α(IV) chains are the following:
- A (Gly)-X-Y collagenous domain of approximately 1,400 residues
- A carboxy-terminal non-collagenous (NC1) domain of approximately 230 residues and 12 conserved cysteine residues, which participate in intrachain and interchain disulfide bonds
- A non-collagenous amino terminal sequence of 15-20 residues
Approximately 20 interruptions of the collagenous triplet sequence are present in the collagenous domain.
Collagen IV chains form helical heterotrimers through associations between their COO- NC1 domains. The heterotrimers form networks through intermolecular interaction such as:
- End-to-end linkages between the COO- NC1 domains of two heterotrimers;
- Covalent interactions between four heterotrimers at their NH- ends; and
- Lateral associations between heterotrimers via binding of the COO- domains to sites along the collagenous region of another heterotrimer.
Linkages between collagen IV molecules form a scaffolding for the deposition of other matrix glycoproteins and for cell attachment.
In the normal developing kidney:
- Collagen α1(IV) and collagen α2(IV) chains predominate in the primordial glomerular basement membrane (GBM) of immature glomeruli.
- The formation of capillary loops within the maturing glomeruli is associated with the appearance of collagen α3, α4, and α5(IV) chains in the GBM.
- As glomerular maturation progresses, the α3, α4, and α5(IV) chains become the predominant collagen IV chains in GBM.
Mechanism of disease causation. Absence or underexpression of the collagen α3, α4, α5, and possibly α6(IV) chains in the basement membrane such that the networks that they form are absent – or, if present, are defective – cause the clinical features of Alport syndrome.
A pathogenic variant affecting one of the chains involved in the collagen IV α345 network can prevent basement membrane expression not only of that chain but of the other two chains as well. Similarly, a pathogenic variant involving the α5(IV) chain can interfere with basement membrane expression of the α6 chain.
Most missense collagen IV variants occur in glycine-encoding codons. The presence of a bulkier amino acid in a glycine position presumably creates a kink or an unfolding in the triple helix, as is observed in collagen α1(I) (see COL1A1/2-Related Osteogenesis Imperfecta and other genetic disorders of collagen). Abnormally folded collagen triple helices exhibit increased susceptibility to proteolytic degradation. The position of the substituted glycine, or the substituting amino acid itself, may influence protein folding and ultimately the severity of the clinical phenotype.
COL4A3- and COL4A4-specific laboratory technical considerations. Variants in COL4A3 and COL4A4 can be associated with either AR or AD disease. There is no specific association between the type of variant (e.g., missense, nonsense, splice site) and inheritance pattern.
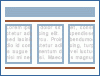
Table 5.
Notable COL4A5 Pathogenic Variants
Chapter Notes
Author Notes
Acknowledgments
The author's work is supported by grants from the National Institutes of Health.
Revision History
- 21 February 2019 (ha) Comprehensive update posted live
- 25 November 2015 (me) Comprehensive update posted live
- 28 February 2013 (me) Comprehensive update posted live
- 15 July 2010 (me) Comprehensive update posted live
- 23 January 2008 (me) Comprehensive update posted live
- 8 January 2007 (cd) Revision: deletion/duplication analysis for COL4A5 clinically available
- 26 September 2005 (me) Comprehensive update posted live
- 29 December 2003 (ck) Revision: sequence analysis for COL4A5, COL4A3, COL4A4 clinically available; prenatal diagnosis available
- 28 August 2003 (me) Comprehensive update posted live
- 28 August 2001 (me) Review posted live
- March 2001 (ck) Original submission
References
Literature Cited
- Antignac C, Heidet L. Mutations in Alport syndrome associated with diffuse esophageal leiomyomatosis. Contrib Nephrol. 1996;117:172–82. [PubMed: 8801045]
- Arrondel C, Deschênes G, Le Meur Y, Viau A, Cordonnier C, Fournier A, Amadeo S, Gubler MC, Antignac C, Heidet L. A large tandem duplication within the COL4A5 gene is responsible for the high prevalence of Alport syndrome in French Polynesia. Kidney Int. 2004;65:2030–40. [PubMed: 15149316]
- Beicht S, Strobl-Wildemann G, Rath S, Wachter O, Alberer M, Kaminsky E, Weber LT, Hinrichsen T, Klein HG, Hoefele J. Next generation sequencing as a useful tool in the diagnostics of mosaicism in Alport syndrome. Gene. 2013;526:474–7. [PubMed: 23732293]
- Bekheirnia MR, Reed B, Gregory MC, McFann K, Shamshirsaz AA, Masoumi A, Schrier RW. Genotype-phenotype correlation in X-linked Alport syndrome. J Am Soc Nephrol. 2010;21:876–83. [PMC free article: PMC2865738] [PubMed: 20378821]
- Bullich G, Domingo-Gallego A, Vargas I, Ruiz P, Lorente-Grandoso L, Furlano M, Fraga G, Madrid Á, Ariceta G, Borregán M, Piñero-Fernández JA, Rodríguez-Peña L, Ballesta-Martínez MJ, Llano-Rivas I, Meñica MA, Ballarín J, Torrents D, Torra R, Ars E. A kidney-disease gene panel allows a comprehensive genetic diagnosis of cystic and glomerular inherited kidney diseases. Kidney Int. 2018;94:363–71. [PubMed: 29801666]
- Daga S, Baldassarri M, Lo Rizzo C, Fallerini C, Imperatore V, Longo I, Frullanti E, Landucci E, Massella L, Pecoraro C, Garosi G, Ariani F, Mencarelli MA, Mari F, Renieri A, Pinto AM. Urine-derived podocytes-lineage cells: a promising tool for precision medicine in Alport syndrome. Hum Mutat. 2018;39:302–14. [PubMed: 29098738]
- Fallerini C, Dosa L, Tita R, Del Prete D, Feriozzi S, Gai G, Clementi M, La Manna A, Miglietti N, Mancini R, Mandrile G, Ghiggeri GM, Piaggio G, Brancati F, Diano L, Frate E, Pinciaroli AR, Giani M, Castorina P, Bresin E, Giachino D, De Marchi M, Mari F, Bruttini M, Renieri A, Ariani F. Unbiased next generation sequencing analysis confirms the existence of autosomal dominant Alport syndrome in a relevant fraction of cases. Clin Genet. 2014;86:252–7. [PubMed: 24033287]
- Gast C, Pengelly RJ, Lyon M, Bunyan DJ, Seaby EG, Graham N, Venkat-Raman G, Ennis S. Collagen (COL4A) mutations are the most frequent mutations underlying adult focal segmental glomerulosclerosis. Nephrol Dial Transplant. 2016;31:961–70. [PubMed: 26346198]
- Gross O, Licht C, Anders HJ, Hoppe B, Beck B, Tönshoff B, Höcker B, Wygoda S, Ehrich JH, Pape L, Konrad M, Rascher W, Dötsch J, Müller-Wiefel DE, Hoyer P, Knebelmann B, Pirson Y, Grunfeld JP, Niaudet P, Cochat P, Heidet L, Lebbah S, Torra R, Friede T, Lange K, Müller GA, Weber M, et al. Early angiotensin-converting enzyme inhibition in Alport syndrome delays renal failure and improves life expectancy. Kidney Int. 2012;81:494–501. [PubMed: 22166847]
- Gross O, Netzer KO, Lambrecht R, Seibold S, Weber M. Meta-analysis of genotype-phenotype correlation in X-linked Alport syndrome: impact on clinical counselling. Nephrol Dial Transplant. 2002;17:1218–27. [PubMed: 12105244]
- Gross O, Netzer KO, Lambrecht R, Seibold S, Weber M. Novel COL4A4 splice defect and in-frame deletion in a large consanguine family as a genetic link between benign familial haematuria and autosomal Alport syndrome. Nephrol Dial Transplant. 2003;18:1122–7. [PubMed: 12748344]
- Gubler MC, Knebelmann B, Beziau A, Broyer M, Pirson Y, Haddoum F, Kleppel MM, Antignac C. Autosomal recessive Alport syndrome: immunohistochemical study of type IV collagen chain distribution. Kidney Int. 1995;47:1142–7. [PubMed: 7783412]
- Hanson H, Storey H, Pagan J, Flinter F. The value of clinical criteria in identifying patients with X-linked Alport syndrome. Clin J Am Soc Nephrol. 2011;6:198–203. [PMC free article: PMC3022243] [PubMed: 20884774]
- Huang SJ, Amendola LM, Sternen DL. Variation among DNA banking consent forms: points for clinicians to bank on. J Community Genet. 2022;13:389–97. [PMC free article: PMC9314484] [PubMed: 35834113]
- Jais JP, Knebelmann B, Giatras I, De Marchi M, Rizzoni G, Renieri A, Weber M, Gross O, Netzer KO, Flinter F, Pirson Y, Dahan K, Wieslander J, Persson U, Tryggvason K, Martin P, Hertz JM, Schroder C, Sanak M, Carvalho MF, Saus J, Antignac C, Smeets H, Gubler MC. X-linked Alport syndrome: natural history and genotype-phenotype correlations in girls and women belonging to 195 families: a "European Community Alport Syndrome Concerted Action" study. J Am Soc Nephrol. 2003;14:2603–10. [PubMed: 14514738]
- Jais JP, Knebelmann B, Giatras I, De Marchi M, Rizzoni G, Renieri A, Weber M, Gross O, Netzer KO, Flinter F, Pirson Y, Verellen C, Wieslander J, Persson U, Tryggvason K, Martin P, Hertz JM, Schroder C, Sanak M, Krejcova S, Carvalho MF, Saus J, Antignac C, Smeets H, Gubler MC. X-linked Alport syndrome: natural history in 195 families and genotype-phenotype correlations in males. J Am Soc Nephrol. 2000;11:649–57. [PubMed: 10752524]
- Kashtan CE. Diagnosis of Alport syndrome. Kidney Int. 2004;66:1290–1. [PubMed: 15327435]
- Kashtan CE. Renal transplantation in patients with Alport syndrome. Pediatr Transplant. 2006;10:651–7. [PubMed: 16911486]
- Kashtan CE, Ding J, Garosi G, Heidet L, Massella L, Nakanishi K, Nozu K, Renieri A, Rheault M, Wang F, Gross O. Alport syndrome: a unified classification of genetic disorders of collagen IV α345: a position paper of the Alport Syndrome Classification Working Group. Kidney Int. 2018;93:1045–51. [PubMed: 29551517]
- Kashtan CE, Ding J, Gregory M, Gross O, Heidet L, Knebelmann B, Rheault M, Licht C. Clinical practice recommendations for the treatment of Alport syndrome: a statement of the Alport Syndrome Research Collaborative. Pediatr Nephrol. 2013;28:5–11. [PMC free article: PMC3505543] [PubMed: 22461141]
- Kashtan CE, Kleppel MM, Gubler MC. Immunohistologic findings in Alport syndrome. Contrib Nephrol. 1996;117:142–53. [PubMed: 8801043]
- Kashtan CE, Segal Y, Flinter F, Makanjuola D, Gan JS, Watnick T. Aortic abnormalities in males with Alport syndrome. Nephrol Dial Transplant. 2010;25:3554–60. [PMC free article: PMC2980995] [PubMed: 20494893]
- King K, Flinter FA, Green PM. A two-tier approach to mutation detection in the COL4A5 gene for Alport syndrome. Hum Mutat. 2006;27:1061. [PubMed: 16941480]
- Levy M, Feingold J. Estimating prevalence in single-gene kidney diseases progressing to renal failure. Kidney Int. 2000;58:925–43. [PubMed: 10972657]
- Lyons OT, St. John ERC, Morales JP, Chan YC, Taylor PR. Ruptured thoracoabdominal aortic aneurysm in a renal transplant patient with Alport's syndrome. Ann Vasc Surg. 2007;21:816–8. [PubMed: 17697765]
- Mencarelli MA, Heidet L, Storey H, van Geel M, Knebelmann B, Fallerini C, Miglietti N, Antonucci MF, Cetta F, Sayer JA, van den Wijngaard A, Yau S, Mari F, Bruttini M, Ariani F, Dahan K, Smeets B, Antignac C, Flinter F, Renieri A. Evidence of digenic inheritance in Alport syndrome. J Med Genet. 2015;52:163–74. [PubMed: 25575550]
- Merchant SN, Burgess BJ, Adams JC, Kashtan CE, Gregory MC, Santi PA, Colvin R, Collins B, Nadol JB Jr. Temporal bone histopathology in Alport syndrome. Laryngoscope. 2004;114:1609–18. [PubMed: 15475791]
- Morinière V, Dahan K, Hilbert P, Lison M, Lebbah S, Topa A, Bole-Feysot C, Pruvost S, Nitschke P, Plaisier E, Knebelmann B, Macher MA, Noel LH, Gubler MC, Antignac C, Heidet L. Improving mutation screening in familial hematuric nephropathies through next generation sequencing. J Am Soc Nephrol. 2014;25:2740–51. [PMC free article: PMC4243343] [PubMed: 24854265]
- Mothes H, Heidet L, Arrondel C, Richter KK, Thiele M, Patzer L, Sado Y, Gubler MC, Antignac C, Scheele J. Alport syndrome associated with diffuse leiomyomatosis: COL4A5-COL4A6 deletion associated with a mild form of Alport nephropathy. Nephrol Dial Transplant. 2002;17:70–4. [PubMed: 11773466]
- Nozu K, Minamikawa S, Yamada S, Oka M, Yanagita M, Morisada N, Fujinaga S, Nagano C, Gotoh Y, Takahashi E, Morishita T, Yamamura T, Ninchoji T, Kaito H, Morioka I, Nakanishi K, Vorechovsky I, Iijima K. Characterization of contiguous gene deletions in COL4A6 and COL4A5 in Alport syndrome-diffuse leiomyomatosis. J Hum Genet. 2017;62:733–5. [PubMed: 28275241]
- Oka M, Nozu K, Kaito H, Fu XJ, Nakanishi K, Hashimura Y, Morisada N, Yan K, Matsuo M, Yoshikawa N, Vorechovsky I, Iijima K. Natural history of genetically proven autosomal recessive Alport syndrome. Pediatr Nephrol. 2014;29:1535–44. [PubMed: 24633401]
- Okamoto T, Nozu K, Iijima K, Ariga T. Germline mosaicism is a pitfall in the diagnosis of "sporadic" X-linked Alport syndrome. J Nephrol. 2019;32:155–9. [PubMed: 30062677]
- Plant KE, Green PM, Vetrie D, Flinter FA. Detection of mutations in COL4A5 in patients with Alport syndrome. Hum Mutat. 1999;13:124–32. [PubMed: 10094548]
- Rahbari R, Wuster A, Lindsay SJ, Hardwick RJ, Alexandrov LB, Turki SA, Dominiczak A, Morris A, Porteous D, Smith B, Stratton MR, Hurles ME, et al. Timing, rates and spectra of human germline mutation. Nat Genet. 2016;48:126–33. [PMC free article: PMC4731925] [PubMed: 26656846]
- Renieri A, Galli L, Grillo A, Bruttini M, Neri T, Zanelli P, Rizzoni G, Massella L, Sessa A, Meroni M, Peratoner L, Riegler P, Scolari F, Mileti M, Giani M, Cossu M, Savi M, Ballabio A, De Marchi M. Major COL4A5 gene rearrangements in patients with juvenile type Alport syndrome. Am J Med Genet. 1995;59:380–5. [PubMed: 8599366]
- Richards S, Aziz N, Bale S, Bick D, Das S, Gastier-Foster J, Grody WW, Hegde M, Lyon E, Spector E, Voelkerding K, Rehm HL, et al. Standards and guidelines for the interpretation of sequence variants: a joint consensus recommendation of the American College of Medical Genetics and Genomics and the Association for Molecular Pathology. Genet Med. 2015;17:405–24. [PMC free article: PMC4544753] [PubMed: 25741868]
- Savige J. Should we diagnose autosomal dominant Alport syndrome when there is a pathogenic heterozygous COL4A3 or COL4A4 variant? Kidney Int Rep. 2018;3:1239–41. [PMC free article: PMC6224634] [PubMed: 30450445]
- Storey H, Savige J, Sivakumar V, Abbs S, Flinter FA. COL4A3/COL4A4 mutations and features in individuals with autosomal recessive Alport syndrome. J Am Soc Nephrol. 2013;24:1945–54. [PMC free article: PMC3839543] [PubMed: 24052634]
- van der Loop FT, Monnens LA, Schroder CH, Lemmink HH, Breuning MH, Timmer ED, Smeets HJ. Identification of COL4A5 defects in Alport's syndrome by immunohistochemistry of skin. Kidney Int. 1999;55:1217–24. [PubMed: 10200983]
- Zhou J, Mochizuki T, Smeets H, Antignac C, Laurila P, de Paepe A, Tryggvason K, Reeders ST. Deletion of the paired alpha 5(IV) and alpha 6(IV) collagen genes in inherited smooth muscle tumors. Science. 1993;261:1167–9. [PubMed: 8356449]
Publication Details
Author Information and Affiliations
Publication History
Initial Posting: August 28, 2001; Last Update: February 21, 2019.
Copyright
GeneReviews® chapters are owned by the University of Washington. Permission is hereby granted to reproduce, distribute, and translate copies of content materials for noncommercial research purposes only, provided that (i) credit for source (http://www.genereviews.org/) and copyright (© 1993-2024 University of Washington) are included with each copy; (ii) a link to the original material is provided whenever the material is published elsewhere on the Web; and (iii) reproducers, distributors, and/or translators comply with the GeneReviews® Copyright Notice and Usage Disclaimer. No further modifications are allowed. For clarity, excerpts of GeneReviews chapters for use in lab reports and clinic notes are a permitted use.
For more information, see the GeneReviews® Copyright Notice and Usage Disclaimer.
For questions regarding permissions or whether a specified use is allowed, contact: ude.wu@tssamda.
Publisher
University of Washington, Seattle, Seattle (WA)
NLM Citation
Kashtan CE. Alport Syndrome. 2001 Aug 28 [Updated 2019 Feb 21]. In: Adam MP, Feldman J, Mirzaa GM, et al., editors. GeneReviews® [Internet]. Seattle (WA): University of Washington, Seattle; 1993-2024.