All rights reserved. Publications of the World Health Organization can be obtained from WHO Press, World Health Organization, 20 Avenue Appia, 1211 Geneva 27, Switzerland (tel.: +41 22 791 3264; fax: +41 22 791 4857; e-mail: tni.ohw@sredrokoob). Requests for permission to reproduce or translate WHO publications – whether for sale or for noncommercial distribution – should be addressed to WHO Press, at the above address (fax: +41 22 791 4806; e-mail: tni.ohw@snoissimrep).
NCBI Bookshelf. A service of the National Library of Medicine, National Institutes of Health.
Anthrax in Humans and Animals. 4th edition. Geneva: World Health Organization; 2008.
8.1. Introduction
This section should be read in conjunction with Annexes 3, 5 & 6.
Control measures are aimed at breaking the cycle of infection depicted in Fig. 9. Each of the following actions must be rigorously implemented:
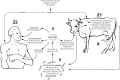
Fig. 9
Breaking the cycle of infection.
- Cut off infection source.
- Dispose of anthrax carcasses correctly (point X).
- Correctly disinfect, decontaminate and dispose of contaminated materials (point Y).
- Vaccinate exposed susceptible animals (point Z1) and, where possible, humans in at-risk occupations (point Z2).
In addition, in some outbreak circumstances in relevant countries, it may be appropriate to consider fly-control measures (point A).
8.2. Discontinuation of infection source
In outbreaks having a defined infection source, clearly discontinuing this source is an essential first step to breaking the cycle of infection (see point Y in Fig. 9). If the infection can be traced to feeding, for example, the feed source should be immediately withdrawn from the index farm and from all others that received it and destroyed. Moving other animals away from the affected area is an important early action. If flies are suspected of being important vectors, fly control should be considered. Detailed practical actions are given in Annex 6.
8.3. Disposal of anthrax (animal) carcasses
See point X in Fig. 9. Please refer also to the OIE Code for general guidance for the disposal of dead animals.1
8.3.1. Principles involved
Because sporulation of B. anthracis requires oxygen and therefore does not occur inside a closed carcass, regulations in most countries forbid postmortem examination of animals when anthrax is suspected. Most, if not all the vegetative B. anthracis cells in the carcass are killed in a few days by putrefactive processes. Nevertheless, with the characteristic (though not invariable) terminal serosanguinous exudates from the nose, mouth and anus, contamination of the environment around an anthrax carcass can still be expected. The precise length of time after which no viable B. anthracis remain within a carcass is unpredictable but depends greatly on climatic conditions, particularly temperature.
8.3.2. Alternatives
8.3.2.1. Livestock
In most countries, the preferred method of disposal of an anthrax carcass is incineration. Controlled heat treatment or “rendering” has been proposed in at least one country of the European Union, but no records were found of this having been done, or of relevant legislative documentation. Where neither of these approaches is possible, for example owing to lack of fuel, burial is the remaining less satisfactory alternative. As discussed in section 3.2, history has many examples of new outbreaks following disturbance of old burial sites.
Consideration might be given to treating anthrax carcasses with 10% formalin, leaving them in situ for some days before disposal while natural putrefaction processes within the carcass kill the vegetative anthrax organisms. The formalin would have the action of killing anthrax organisms shed by the dead animal, preserving the skin so that it retains the anaerobic environment within the putrefying carcass. It may also deter scavengers that would otherwise open up the carcass and thereby increase the contamination, and flies that might spread the disease. However, it was the experience in the major outbreak in wildlife in Zimbabwe (Clegg et al., 2006a, 2006b), that formalin treatment of carcasses per se did not deter scavengers or flies (Clegg & Wenham, personal communication, 2005).
The procedure adopted in the large livestock outbreak in Victoria (Australia) in 1997 was to burn carcasses at the death site or as near as possible to that site. External spore contamination was minimized by spraying with 5% formaldehyde. Where incineration could not be done at the death site and transportation to another site for incineration was necessary, this was done by loading the formalin-sprayed carcass onto double-thickness plastic on a low-loading trailer, and wrapping it in the plastic (Turner, personal communication, 2003).
In some developing country situations where burial, incineration or rendering is not feasible, the last resort may be to leave the carcass unmoved in situ and ensure that it is inaccessible to other animals, particularly scavengers, or people. This is achieved by covering with tarpaulins, branches of trees, corrugated iron or other available materials. Hazard signs should be posted around sites in this case. This again allows the putrefactive process to take effect, although residual environmental contamination may still remain, and either the site should be scorched after putrefaction is complete or it should be treated with 10% formalin. Alternatively, it should be made inaccessible to other animals indefinitely by fencing, capping with concrete or other impervious material, covering with brushwood, or growing impenetrable undergrowth.
Hugh-Jones (personal communication, 2005 reports that in Argentina, carcasses are first covered with lime (see section 8.3.3), then a tarpaulin, and left for 9 months before the bones are collected. This is said to stop scavenging and is cheaper than burying.
8.3.2.2. Large outbreaks in wildlife
Suggested practical actions in the event of an anthrax outbreak in wildlife are given in Annex 6, section 5.
In national parks from which livestock are excluded and which have “hands-off” management policies for all but emergency situations, control actions may be regarded as interference with natural processes. This is discussed in sections 8.9 and 8.10. Frequently, carcasses of animals that have died of anthrax on a sporadic basis will only be seen some time after death, if they are seen at all, and they will have been opened up by scavengers. It is likely in enzootic areas that for every one seen by park officials, many are not seen. Consequently, it is impractical to attempt to rigidly enforce “burn or bury” action plans. It can also be counterproductive (i) environmentally, through depletion of scarce wood for burning or from pollution if fuel such as diesel fuel is used, and (ii) by taking underresourced human time from more valuable activities.
Where action is seen to be necessary, all carcasses are again best burnt but, if this is impractical, they should be buried. In the case of burial (and possibly burning also) consideration may be given to spraying the carcasses and surrounding ground with 10% formalin to minimize the number of spores which may survive and resurface, causing cases again at some point in the future (section 3.2).
8.3.3. Burial
Periodic reports of viable anthrax spores at burial sites of animals that died many years previously, and incidents and outbreaks in animals associated with such sites, have testified to the unreliability of burial procedures for long-term control of the disease (section 3.2). Disturbance of such sites, for example by ploughing or laying drainage, presumably brings the spores to the surface. Even without site disturbance, spores can work their way up to the soil surface. In either case, this may result in new livestock cases as shown by Turnbull et al. (1996). Further disadvantages of burial sites are that scavengers may dig down to reach the carcass, and in dry dusty areas, the digging process can spread the contaminated soil extensively. In Wood Buffalo National Park, Canada, where burials of bison that had died of anthrax were carried out in the 1960s, the raised burial mounds became excavation sites for foxes and wolves building their dens and nesting sites for ants (Dragon, personal communication, 1997). In Africa as an example, it is hard to stop dogs from digging up buried anthrax (cattle) carcasses.
The origins of the common recommendation to bury with lime appear to be lost to history. In theory heat would be generated on contact with body fluids, and that would thereby be expected to hasten decomposition of the carcass. It may have been applied originally to deny corpses (human or animal) to relatives/owners after death where that was officially needed (Hugh-Jones, personal communication, 2004). It is now uncertain just what lime does to buried carcasses, whether it accelerates their disintegration or actually preserves them. Lindner & Böhm (1985) believed that at least as far as lime treatment of tannery effluent is concerned, this probably followed the use of lime as a cheap chemical for disinfecting sewage sludge. However, they showed that at 20 kg/m3, lime failed to inactivate anthrax spores in sewage sludge. In raising the pH of the soil, addition of lime when burying anthrax carcasses may actually be counterproductive to minimizing long-term spore contamination (section 2.1.2.4).
In summary, burial should be discouraged in favour of incineration.
8.3.4. Incineration
Guidelines on incineration procedures are given in Annex 3, section 7. Ideally, the soil surrounding and under the carcass, particularly around the nasal and anal regions, should be decontaminated and then incinerated with the carcass.
Incineration must be carried out with appropriate care to ensure complete burning from beneath. Usually this involves raising the carcass off the ground before the process is started. Mobile commercial incinerators designed to ensure this are available (see Figs 10A–D). It must be appreciated that spores that have soaked into the soil may survive the incineration process, although isolation of B. anthracis from incineration sites is rare. The down-directed blow-torch shown in Figs 10E& F illustrates an alternative incineration procedure that ensures severe scorching of the soil to several centimetres of depth (see also Annex 3, section 7).
Comments are occasionally encountered opposing incineration on the basis that anthrax spores may survive the fire and become aerosolized in the updraft. In general, circumstantial evidence does not support the contention that incineration of anthrax carcasses results in the transmission of anthrax in this manner, and the rapid dilution effect on any spores that may become airborne in viable state reduce the chances of these causing an infection to next to nil. The generally high infectious doses for anthrax by routes other than through a lesion even in the more susceptible species supports this contention (see sections 3.1, 3.3.4, 4.2.1). Nevertheless, suspicion that airborne transmission from carcass incineration occurs has arisen. Henton & Briers (1998) described an outbreak of anthrax in the summer of 1995–1996 in the Kimberley district of South Africa affecting goats, cattle, sheep, horses, roan antelope, gemsbok, laldu and springbok. The outbreak developed by spreading from the initial focus in a south-westerly direction over a distance of 270 km (40–50 km wide). They considered the distances involved too great for the spread to be accounted for in terms of insect transmission, and believed it to result from windborne spores emanating from incineration sites. In support of this possibility, they demonstrated that B. anthracis could be isolated in low numbers from cotton wool held in the smoke above three carcasses and from the face mask of one of the veterinary officers assisting in the incinerations.
Blenkharn & Oakland (1989) were able to isolate Gram-positive bacteria, predominantly Bacillus species, from the base of the exhaust stack of a hospital waste incinerator with design-specified operating temperatures of 800 °C and 1000 °C in the primary and secondary chambers respectively, thereby demonstrating that there is no room for complacency. However, numbers were very low, averaging 56 cfu per cubic metre (range 0–400 cfu per cubic metre, i.e. <1 cfu per litre) and would be subject to rapid further dilution on leaving the chimney. A badly constructed pyre producing smoke with little or no flame might result in a higher survival rate of organisms collected by the updraft.
An additional consideration is that anthrax organisms in an unopened carcass are in the vegetative form and are readily susceptible to heat and other adverse conditions. The spores will be confined to where the blood has been shed through the body orifices and will mostly be in the soil beneath these points. Relatively few spore forms, therefore, will enter the fire and updraft; vegetative forms will almost certainly not survive. If concern persists, consideration might be given to pretreating the carcass and associated contaminated soil with 10% formalin a few hours before incineration to minimize the number of viable spores present (section 8.3.2.1; Annex 6, section 1).
8.3.5. Rendering
Rendering is essentially a cooking process that results in sterilization of raw materials of animal origin such that parts of carcasses may be utilized safely for subsequent commercial purposes.
There are a number of variations of the rendering process, broadly divided into batch processes and continuous processes. In general, the raw materials are finely chopped and then passed into a steam– heated chamber and subjected to temperatures ranging from 100 °C to 150 °C for 10–60 minutes (this does not include the time taken to bring the material to the peak temperature or the subsequent cooling period time).
The rendering procedure involves correct performance at each of three stages: collection, transport and treatment of the carcass (Riedinger et al., 1975; Riedinger, 1980; Strauch, 1991). These should be supervised by veterinary authorities. The carcass should be bagged and the bag, collection machinery, materials and tools, and the carcass site itself appropriately decontaminated and disinfected. The rendering plant must be properly divided into “dirty” and “clean” areas, which must not be connected via a common drain to avoid possible cross-contamination by back-flow. The dirty side must be suitably equipped for disinfection of the transport vehicles and other equipment involved. Wastewater from the dirty side must be collected and treated by heat or chemicals (preferably heat) to destroy the spores. Before the heat treatment, carcasses should be broken down into pieces not larger than 10 cm3. In the case of anthrax carcasses, this should be done with very careful attention to hygiene during the process, with the necessary disinfection and decontamination of the rendering premises, tools, clothing, waste run-off, etc.
Controlled heat treatment is then carried out with temperature, pressure and time of sterilization recorded.
As well as careful hygiene control on the dirty side of the rendering plant, the level of hygiene being maintained on the clean side should also be monitored at least twice yearly by the veterinary authorities.
8.4. Human cases: infection control in management
The risk of human–to–human transmission is not a serious one given that sensible precautions are taken (section 4.3.2). For example, cutaneous anthrax lesions should be dressed for the first 24–48 hours after treatment; disposable gloves should be worn, or gloves that can be sterilized, while applying the dressing and during subsequent disposal of specimens or sterilization of materials and equipment (see Annex 3, sections 3 & 4).
Prophylactic antibiotics and vaccination are not necessary for health workers or family contacts, although these individuals should know that their medical practitioner should be consulted if suspicious sores or illness develop that might have arisen from their contact.
In fatal cases, postmortem examinations should be discouraged; cremation is preferable to burial where local custom permits. It is advisable for the body to be placed in an impervious body bag for transport from the place of death, and preferably the body should not be extracted from the bag. Where only burial is permitted, the bagged body should be placed in a hermetically sealed coffin and buried without reopening. Useful guidelines are available elsewhere (Healing et al., 1995; Young & Healing, 1995). Bedding and contaminated materials should be bagged and incinerated, autoclaved or fumigated as appropriate. Whether room fumigation is necessary will depend on the perceived level of contamination in the room where the patient died (see Annex 3, sections 3.1–3.3).
8.5. Fumigation, disinfection, decontamination
See point Y in Fig. 9.
8.5.1. Principles
In addition to helping break the cycle of anthrax infection locally, disinfection, decontamination and correct disposal of infected/contaminated material are of considerable importance in preventing long-distance and international transmission of anthrax. In non-endemic countries, risks arise largely from animal products – wool, hair, hides, bone, etc. – imported from endemic regions. Regulations regarding importation of untreated animal products vary from country to country, but several importing countries take the view that the financial costs that would be incurred from legislating for the sterilization of such imports would be disproportionate to the benefits.
In many countries, some or all of the following requirements are in place to limit the risks of importation or dissemination of products contaminated with anthrax spores:
- Products must be accompanied by a certificate signed by a veterinary official in the country of export certifying that they derive from anthrax-free sources (see Annex 4).
- Products regarded as having a high chance of containing anthrax spores are subject to some form of monitoring or control.
- Finished or raw materials from certain countries may be subject to import restrictions, such as treatment before export.
- Finished or raw materials from certain countries may have to undergo a general sporicidal treatment after arrival and before processing or distribution.
In the case of hides and skins (where a sterilization procedure that does not damage the materials has never been devised), the exporting countries nowadays often require the initial processing stages to be carried out before export for financial benefit. This has contributed to a reduction in anthrax-contaminated hides and skins now reaching non-endemic countries as compared with a few years ago, although on the other hand, exporting countries have experienced increased incidence of the disease downstream from the preprocessing tanneries through lack of adequate control programmes (Hugh-Jones, personal communication, 2004).
Practical approaches to disinfection and decontamination of animal products are given in Annex 3, section 6. However, it is stressed that long-term global control depends almost entirely on the application of appropriate measures to prevent the disease among livestock in enzootic exporting countries. Ideally, national policies should ensure that materials known to be contaminated with anthrax spores are appropriately disposed of and are not included in any industrial process.
8.6. Prophylaxis
8.6.1. The nature of protection in anthrax
Four decades of research have resulted in the perception that protection against anthrax in the susceptible host depends almost entirely on the host’s immune response to a single antigen – the protective antigen (PA) component of the anthrax toxin (Ivins & Welkos, 1988; Turnbull et al., 1988; Turnbull, 1991; Friedlander et al., 2002; see section 5.5.3), and the design of putative novel vaccines has been based on this. Immune responses to the other two toxin components, the lethal and oedema factors, may contribute to or enhance protection. One group has proposed that at least the lethal factor component of the anthrax toxin has an important role to play (Price et al., 2001). In contrast, another group (Cohen et al., 2000) obtained protection without the development of measurable anti-PA antibodies and suggested that other, spore-associated antigens may contribute in a significant manner to protective immunity. As yet, no other such antigens have been identified, although alternative possible candidate vaccine antigens continue to be sought, such as S-layer proteins (Fouet et al., 1999; Mesnage et al., 1999; see section 5.5.5) and the collagen-like surface glycoprotein BclA, a major antigen of the exosporium (Sylvestre et al., 2003; Steichen et al., 2003). The possibility that the capsule may be capable of enhancing protective efficacy has also been mooted (section 5.5.1).
Although it has long been believed that the effectiveness of both animal and human vaccines depends on the induction of anti-PA antibodies, it has also been recognized for some years that measurable anti-PA antibodies in the blood of an individual are not, in themselves, a guarantee of protected status although, the theory continues, they must be there for the individual to be protected (Ivins & Welkos, 1988; Turnbull et al., 1988; Ivins et al., 1990a, 1990b; Turnbull et al., 1990b). Certainly the immune response is complex; the recently-acquired knowledge that the macrophage is central to lethality in anthrax (see section 5.4.1) and evidence that Th1 and Th2 responses may be involved in protective efficacy (McBride et al., 1998) – possibly to different extents in different species – indicate that cellular immunity has a role in protection, although this is undefined at present. The enhanced efficacy of future vaccines over existing ones may depend on improved stimulation of the cellular immune response in parallel with the humoral response to PA (see section 8.6.4).
The mechanism whereby an immune process based on antitoxin immunity protects against a disease characterized by rapid in vivo growth of the bacilli is not known yet. Neutralization of the effect of the toxin on macrophages may be a major factor. It is also possible that some action of the toxin that results in the release of nutrients needed for multiplication is inhibited. PA appears to be expressed very early in the germination of spores and is rapidly released. Antibodies to PA may have a role in retarding germination or enhancing spore clearance by phagocytic cells (Welkos et al., 2002; Cote et al., 2005).
8.6.2. Animal vaccines
Gochenour et al. (1935) summarize the situation at the end of the first third of the 19th century:
“Undoubtedly, Pasteur’s ability to protect animals against anthrax by vaccination was heralded at the time as a sure means of preventing that dreaded disease of livestock. The vaccines subsequently prepared by Pasteur did much to control the disease. Experience has shown, however, that the Pasteur vaccine had definite limitations. … Accordingly, numerous investigators undertook the task of developing anthrax biologics … as a result of [which] a number of products have been developed for the control of anthrax. For the immunization of animals against anthrax, the veterinarian has, therefore, a number of biologics at his command, namely, anti-anthrax serum, anti-anthrax serum and anthrax-spore vaccine used simultaneously, anthrax-spore vaccine (single injection), anthrax-spore vaccine (intradermic), anthrax-spore vaccines (2, 3, or 4 injection), anthrax-spore vaccine in saponin solution, anthrax aggressin, and two kinds of killed-organism anthrax bacterins, one being a whole-culture anthrax bacterin, and the other a washed-culture bacterin.”
Most anthrax vaccines for animals in use around the world today utilize the toxigenic, non-capsulating (pXO1+/pXO2–) B. anthracis strain 34F2 derived from a virulent bovine isolate in the 1930s (Sterne, 1937a, 1937b; Alper, 1996). The Pasteur-type vaccines (pXO1-/2+), which carried a ≥ 3% mortality risk, have now been abandoned in almost all countries. The animal vaccines that use strain 34F2 are essentially as originally formulated (Sterne, 1939) with approximately 107 spores per ml suspended in 0.5% saponin in 50% glycerine-saline (Annex 5, Table 18).
Animal vaccines against anthrax should be prepared in accordance with the Requirements for anthrax spore vaccine (live – for veterinary use), Requirements for biological substances No. 13 (WHO, 1967), the Manual for the production of anthrax and blackleg vaccines (FAO, 1991) and the Manual of diagnostic tests and vaccines for terrestrial animals (OIE, 2008).
The WHO Requirements for anthrax spore vaccine (live – for veterinary use) points out the considerable differences in quality that may exist between anthrax vaccines. Glycerine and saponin are important to vaccine performance. Vaccine strains should be maintained carefully since unencapsulated B. anthracis variants may lose their immunogenic powers on subculture (Sterne et al., 1939; Sterne, 1959). Since, in theory, the vaccine is easy to prepare, many countries undertake their own manufacture (Annex 5, section 2.13). However, it is important that careful quality control be exercised to avoid resurgence of anthrax because inadequate vaccines are being used.
The protective effect of a single dose of strain 34F2 vaccine is said to last about one year (Sterne, 1939), and annual boosters are recommended for livestock in endemic areas. The duration of the protection has never been systematically studied in livestock or laboratory animals, and this is an area of research that needs attention. A small study set up to examine the effect of maternal antibody on the response of calves to vaccination, described in Annex 5, section 2.11.3, showed that after two doses 4–5 weeks apart, and with dose 1 administered at 5–9 weeks of age, titres had fallen to just measurable by 5–6 months after dose 2. Some information has also emerged from studies on vaccinated wildlife. In a study on antibody levels to PA in vaccinated zebra in the Etosha National Park in Namibia (Lindeque et al., 1996b) it was evident that two initial doses approximately 8 weeks apart were necessary for the development of dependably measurable antibody titres, and the decline in titre one year after the second booster indicated that the next booster should be administered no later than that. A study in vaccinated cheetah utilizing passive protection in mice (Turnbull et al., 2004b) showed that more than one initial dose was necessary to ensure substantial protective immunity, and that lasting immunity might not set in until after a subsequent booster. Although the manner in which the vaccinations were given in that study did not permit the recommendation of a precise schedule, it was felt that the logical schedule, at least in that species, would be two initial doses two months or more apart, followed by annual boosters.
Strain 34F2 and its analogs in China and the Russian Federation lack the genes for capsule formation but still produce the toxin (i.e. they are pXO1+/2-). They thus possess “reduced” virulence, rather than being totally avirulent (Welkos et al., 1986; section 5.5.7) and occasional losses occur. Overdosing with the live spore vaccines based on these strains is hazardous. Certain species such as goats (Sterne, 1939) appear to be especially susceptible to adverse reactions, and the vaccines need to be used with extra caution in these (see Annex 5, section 2.5).
The extent to which vaccination should be applied to control an outbreak will be determined by the nature of the outbreak. Sporadic confined outbreaks will only require one herd vaccination, whereas annual vaccination may be required for extensive periods following widespread outbreaks (see also section 8.7).
Other limitations of the live spore vaccines in use today are (i) limited duration of effect (animals in enzootic areas should be immunized annually as discussed above); (ii) they should be administered parenterally; and (iii) efficacy and unwanted side-effects may be greatly influenced by small faults in production or administration. Items (i) and (ii) become important in developing countries where appropriate equipment and manpower are limiting factors; item (iii) has increasingly become relevant as “local” production has tended to replace manufacture by a few major companies or centres.
8.6.3. Human vaccines
In China and in the Russian Federation, live spore vaccines are prepared and licensed for human use (Annex 5, section 3). The Russian vaccine, dating from the 1930s and 1940s (Shlyakhov & Rubinstein, 1994), uses strain STI-1, analogous in its derivation to Sterne’s 34F2 (STI was derived from strain “Krasnaya Niva”, isolated from a horse that had died of anthrax (Cherkasskiy, personal communication, 2002). It was licensed for administration by scarification in 1953 and by subcutaneous injection in 1959. Good target populations for clinical trials existed in the southern European and middle Asian Soviet republics, where a 75%–84.2% rate of effectiveness was apparently recorded (Demicheli et al., 1998). The available information is that the Russian vaccine may be procured outside the Russian Federation. China however only produces enough to meet its national needs, and the Chinese vaccine is not available outside the country.
Trials were conducted by Russian workers in the 1950s and 1960s on the possibility of carrying out vaccination by the inhalational route. Large inhaled doses (20–50 million spores or more) of live vaccine strain spores were found to be needed to induce substantial protective immunity in various animal species by the inhalational route (Anon., 1967; Lebendinskii, 1971; Ogarov & Gapochko, 1975). At this level of dose, generalized infection by the vaccine strain occurred. Lebendinskii (1971) reviewed a number of papers published between 1958 and 1967 describing the testing of aerosolized “dust” anthrax vaccines on some 4000 humans using doses of 15–300 million STI strain spores. A further 260 persons were vaccinated with highly dispersed liquid aerosols of STI strain spores. Although the only pathophysiological changes noted were a transient moderate leucocytosis with slight shift to the left, as monitored by the AnthraxinT test (section 4.4.2.2), the percentage of positive skin tests, which peaked at 3 months, depended directly on the dose of vaccine inhaled.
In the United States and the United Kingdom, nonliving human vaccines developed in the 1950s and 1960s, and licensed in 1972 and 1979 respectively, are produced (Turnbull, 2000; Friedlander et al., 2002). However, their availability has recently been largely restricted to their respective national needs, for the most part defence-related. However, they remain nominally available for persons in other at-risk occupations, primarily industrial workers concerned with processing of animal products from endemic regions. For further information, the relevant organization given in Table 19 should be contacted directly. The United Kingdom vaccine is an alum-precipitated cell-free culture filtrate of strain 34F2, while the United States vaccine is an aluminium hydroxide-adsorbed cell-free culture filtrate of a non-capsulating, non-proteolytic derivative of bovine isolate V770. No evidence has been found of anthrax vaccines for humans produced or licensed in other countries.
There are no human efficacy data for the current United States and United Kingdom vaccines and the extent and duration of the protection afforded in humans by vaccination with these is uncertain. In the case of the United Kingdom vaccine, the original administration schedule was 2 doses of 1 ml at days 1 and 10 with an annual booster. This was based on protection tests in monkeys challenged with 10–15 LD50 of spores by the inhalation route 7 days (group 1), 1 year (group 2) and 2 years (group 3) after dose 2. All animals in groups 1 (10 animals) and 2 (10 animals) survived; 1 of 7 in group 3 died. All controls died (Darlow et al., 1956). Three-dose schedules were adopted a few years later and subsequent assessment of the efficacy of the United Kingdom vaccine depended on observations in the 1960s of decreasing incidence in the wool and hide industries where vaccination programmes were in place, while infection rates outside these trades remained steady (Darlow & Pride, 1969), and of a fourfold decline in the number of reported cases (including both cutaneous and inhalational forms of the disease) between the early 1960s and the late 1970s, in parallel with the introduction of the vaccine (CDSC, 1981). There is also no case on record of anthrax in a human vaccinated with the United Kingdom vaccine.
With the United States vaccine, now commonly referred to as AVA (anthrax vaccine adsorbed), a study of mill workers when the vaccine was first being introduced recorded a protection rate of 93% (Brachman et al., 1962). In its early days of use in mill workers processing animal products from anthrax-endemic regions of the world, four cases were recorded in partially-vaccinated persons and one in a fully-vaccinated individual (Brachman et al., 1962). This vaccine has also evolved somewhat since then, and more recent evaluations in rhesus monkeys indicated that it is able to confer a high degree of protection against aerosolized spore challenge (Ivins et al., 1996, 1998).
The United States and United Kingdom vaccines have been associated with complaints of reactogenicity, although this rarely exceeds mild erythema, soreness and swelling at the site of injection lasting 2–3 days (Turnbull, 2000). The topic is being studied in depth for the United States vaccine (Marano et al., 2005). No adverse effect “in more than 30 years” has been claimed for the Russian STI vaccine (Shlyakhov & Rubenstein, 1994).
In certain circumstances, including following known or suspected exposure as a result of a deliberate release event, postexposure vaccination at the same time as administration of antibiotics may be appropriate (see section 7.3.2.1).
Vaccination of humans who are not occupationally exposed to anthrax is inappropriate. For the general public, control of the disease should be done through control in livestock.
8.6.4. Prospective new vaccines
8.6.4.1. Human vaccines
The basic prerequisite for protection against anthrax is an adequate humoral immunity to the protective antigen (PA) combined with an appropriate cellular immune response (section 8.6.1). This should be taken into account in the design of new vaccines. While cellular immune stimulation can be achieved by means of an effective adjuvant, the surest way of ensuring this combined humoral and cellular response is to immunize with live vaccines. The current animal vaccine (section 8.6.2) is a live vaccine, and its greater efficacy over the nonliving human vaccines (section 8.6.3) in animal protection studies is attributed to its greater stimulation of cellular immune responses in the recipients (Ivins & Welkos, 1988; Turnbull et al., 1988; Turnbull, 1991).
When first introduced for administration to workers in at-risk occupations, the United States human anthrax vaccine of the time was shown to be clearly associated with protection against the disease (Brachman et al., 1962). That paper contains the only existing reference to anthrax in a fully-vaccinated person (section 8.6.3); since then, there have been no other such cases on record. However, protection tests in laboratory animals have resulted in a confusing view of the efficacy that can be expected from the currently licensed human nonliving vaccines for a given challenge situation, and of the duration of the protection they afford. Being essentially simple culture filtrates, the vaccines are somewhat undefined, lack consistency in production and involve a cumbersome dosing schedule. They are occasionally associated with complaints of side-effects (Turnbull, 2000). The case for the development of new vaccines rests on these issues.
The aims for prospective new vaccines are that they should be:
- well-defined with well-characterized ingredients and mode of action;
- effective against challenge with any anthrax strain;
- safe, giving no dangerous side-effects in any species;
- long-lasting;
- easy to administer rapidly and by non-skilled persons;
- requiring just one dose, or very few doses;
- orally administrable, especially for livestock and at-risk wildlife species;
- cheap;
- environmentally acceptable.
The next-generation human vaccines are likely to be parenterally administered nonliving vaccines consisting of purified recombinant PA with adjuvant. Two such vaccines are in clinical trials, and progress towards licensure for one or both of these can be tracked on the Web through standard search engines. At present, only certain aluminium salts are approved for use as adjuvants in human vaccines; these induce Th2 responses predominantly. It is thought that this may be advantageous for promoting protective immunity in primates and that it may also account for the apparently better performance of the current licensed Al3+-adjuvanted vaccines in protecting monkeys as compared with guinea-pigs and mice in which, it is theorized, stimulation of Th1 responses may be more advantageous (see also section 8.6.1).
For subsequent-generation human vaccines, pressure is likely to arise for the vaccine to be based on more than just PA, and to include at least one or both of the other toxin components, LF and EF, and possibly other antigens such as S-layer proteins or even the capsule (Fouet et al., 1999; Friedlander et al., 2002). In this case a mutant form of the PA that can no longer combine with LF and EF (Singh et al., 1989) and which is, therefore, non-toxigenic while still being fully immunogenic, is likely to be involved. It may be that this will take the form of a DNA vaccine (Price et al., 2001) rather than a protein vaccine, with the DNA encoding the desired vaccine antigens, whole or mutated as desired. At this stage it is difficult to predict how many doses would be needed for effectiveness, whether a non-parenteral route of administration may be possible, or the likely duration of the conferred protection.
8.6.4.2. Veterinary vaccines
It is probably due in part to the known effectiveness of the Sterne strain and analogous vaccines, and in part to the relative unimportance in veterinary spheres of anthrax as a day-to-day disease, that there has been little interest shown in developing new livestock vaccines. The capability to do so certainly exists. Vaccines producing stronger initial immunity, and immunity of longer duration with lower potential risks of casualties, would certainly be desirable. Particularly useful would be a vaccine that is unaffected by antibiotics, permitting simultaneous treatment and vaccination during outbreaks.
8.6.5. Studies on orally administered live spore vaccines
As indicated in section 8.6.4.1, the ideal vaccine would be an oral formulation that:
- meets the requirements of manufacturers for a readily-standardized production with batch-to-batch reproducibility;
- meets the requirements of regulatory bodies for readily-demonstrated pharmacological and safety data;
- meets the needs of recipients for a single-dose administration resulting in rapid development of immunity and lasting efficacy with no undesirable effects;
- could also be a combination vaccine covering multiple-disease entities;
- could be administered with equal effectiveness to humans, domestic animals or wildlife as needed;
- could be administered in an easy and non-invasive manner;
- would be cheap.
Current anthrax vaccines are administered by parenteral injection. Claims of immunization with a level of success by oral delivery of anthrax vaccines appear to be confined to the reference by Ebedes (1976) to an oral vaccine that protected guinea-pigs from repeated challenge with virulent anthrax spores, the reports of Rengel (1993) and Rengel & Boehnel (1994, 1995) on the results of feeding Sterne vaccine strain spores to guinea-pigs, unpublished claims in Texas of reduced disease in white-tailed deer given Sterne-strain spores mixed in with their feed (Hugh-Jones, personal communication, 2004), and partial protection in challenged mice that had been immunized with a Salmonella typhimurium construct expressing the B. anthracis protective antigen (PA) gene (Coulson et al., 1994).
The evidence for Bacillus species being capable of germinating, colonizing and/or multiplying in the intestinal tract is tenuous. Granum et al. (1993) express the opinion that the diarrhoeal syndrome of B. cereus may result from in situ production of the enterotoxin within the intestine rather than from ingestion of preformed toxin, basing this on the ability of the bacterium to grow anaerobically. The same group (Andersson et al., 1998) considered their demonstration of hydrophobic attachment of the spores of certain strains of B. cereus to monolayers of Caco-2 human epithelial cells to indicate that such strains might be capable of epithelial adhesion and colonization within the intestine. Similarities between B. cereus diarrhoeal-type food poisoning and food poisoning by Clostridium perfringens, which is known to colonize the intestine, is another argument, by analogy, for Bacillus species being able to colonize, germinate and multiply within the intestine. It is well documented that LD100s and LD50s for fully virulent B. anthracis by the oral route are enormously high, even in species regarded as highly susceptible to infection (see section 3.1). Most reports indicate figures of 108 or higher, although de Vos (1990) gives an oral LD50 of about 1.5 x 106 spores for impala. This compares with parenteral route LD50s in the order of tens or hundreds for the same species where documented. Illness and recovery following oral challenge clearly can occur with non-lethal doses (Schlingman et al., 1956; Jackson et al., 1957; Redmond et al., 1996a, 1997) followed by seroconversion (Jackson et al., 1957; Redmond et al., 1996a, 1997). To what extent these oral-dose data reflect some, if limited, colonization or toxin production within the gut, as opposed to simply reflecting the points at which a proportion of the very large numbers present overcome the natural intestinal barriers (Walker & Owen, 1990), is impossible to say.
The bottom line is that it is not known whether vaccine strains such as the Sterne strain or recombinants, such as B. subtilis WB600 (pPA101), are capable of producing PA/rPA in situ and, if so, to an extent where immunity can result. In a study to address this question (Turnbull et al., 2001a), guinea-pigs were fed by stomach gavage on days 1, 21 and 42 with 1 ml volumes of spore suspensions of B. subtilis WB600 pPA 101-1, B. anthracis Sterne animal vaccine and B. globigii (control group), all pre-adjusted to 108 cfu per ml. Faecal samples were collected from each individual animal before each feeding session for IgA anti-spore or anti-PA antibody analysis and, after each feeding session, all faeces were collected to day 6 for determination of numbers of excreted spores. Following test bleeding, the guinea-pigs were challenged intramuscularly with 103 cfu of B. anthracis Vollum strain spores on day 64. In all three groups, the administered organisms were recovered exclusively in the spore form in the faeces and became undetectable by day 6. There was no evidence of multiplication within the gastrointestinal tracts of the animals. Increasingly rapid declines in faecal counts, reflecting accumulating immunity, were not readily apparent with successive feeding sessions, and IgG and IgA anti-spore or anti-PA antibodies were not detected in faecal and/or serum samples. All the guinea-pigs succumbed to the challenge with no significant differences in time to death between the livestock vaccine (B. anthracis) group, the recombinant B. subtilis producing PA group and B. globigii controls. The indications, therefore, were that the vaccine strains failed to colonize, produce PA and thereby induce immunity.
Similarly, Hugh-Jones and colleagues (personal communication, 2005) carried out a field trial in 2004 with 15 goats, feeding them 60 times the prescribed parenteral dose of Sterne vaccine together with crushed pecan nuts. Just one of the animals developed a low anti-PA titre.
8.7. Decisions on treatment and/or vaccination of livestock
While vaccination of livestock is the fundamental control measure in enzootic areas with seasonal recurrence of the disease, when an incident occurs unexpectedly in a non-endemic area, antibiotic treatment of exposed animals may be preferable to vaccination – or at least more immediately practical – as the primary control measure. Vaccination may be added as an adjunct if prolonged incubation periods are of concern and there is reason to fear that an incident is going to develop into an outbreak. In fact, in temperate climates such as the United States, where years may elapse between anthrax outbreaks in a given locality, anecdotal evidence suggests that significant decreased livestock losses may be associated with a control programme of first administering a long-acting antibiotic followed by vaccination after 7 to 10 days.
The decision on whether to vaccinate should be made soon after infection with anthrax has been confirmed. With livestock, when infection has been confirmed in the first case, immediate vaccination of all animals thought to have an equal chance of exposure can be expected to result in no further cases. On the other hand, where multiple cases have already occurred, further cases may occur over the next week or two despite vaccination, presumably in animals already incubating the disease. In this case, antibiotic treatment followed by vaccination after 7–10 days is the best control option (Annex 5, section 2.2).
Only animals thought to have an equal chance of exposure need be vaccinated if separation from other animals can be assured. If separation cannot be assured and other animals will have access to the infected group and/or infected site(s), or if multiple cases have occurred, neighbouring herds and flocks should be vaccinated. In an outbreak situation ring vaccination may need to be applied to a distance of 1 km beyond an infected property. If biting flies are considered responsible for the spread of the outbreak, the ring should cover an area exceeding the distance normally travelled by the flies.
All animal vaccines are live vaccines, and their use requires a withholding period prior to slaughter for human consumption. This period may be stipulated on the label of the vaccine or in the accompanying leaflet, and varies from 3 to 6 weeks. Withholding periods from slaughter of up to 6 weeks are also required by some countries for the purposes of trade in meat products. There is no withholding period for milk destined for human consumption following vaccination of milking animals with Sterne 34F2 strain vaccine. There are no known reports of illness in humans following the consumption of animal products from animals immunized with that vaccine.
Anthrax vaccines, being living organisms, are restricted to veterinary use in some countries, and in some jurisdictions they can only be used with the approval of the official veterinary service. It is important that local requirements be met.
8.8. Duration of veterinary vaccination programmes
Despite the well–known longevity of anthrax spores, decline in spore numbers, as evidenced by reduced outbreaks, does occur through decay and/or dispersal at contaminated sites (see also sections 2.1.2.4, 2.1.2.6). It was the experience of Max Sterne, and others since, that pastures associated with anthrax continued to give rise to cases for up to three years after the index cases. Therefore, if there has been an outbreak on a farm, the stock should be revaccinated annually for at least three years to prevent further cases.
8.9. Intersectoral issues: the question of eradication
As with any zoonosis, intersectoral cooperation is essential for effective control of anthrax. Public health officials should be notified by the veterinary authorities in the event of outbreaks in livestock so that they become alert to the possibility of associated human cases. Likewise, medical authorities should notify veterinary health officials when a human case is encountered (see section 9.1.2).
Cooperation between livestock officials and wildlife managers in enzootic zones is more complex. While the target of the former is eradication from the region, the disease is regarded in large game-management areas where it is not rate-limiting in any of the species as an integral part of natural population-control mechanisms. In these, action, usually vaccination, is often seen as being necessary only when endangered species are at risk, and is usually targeted specifically at that species (section 8.3.2.2). Beyond this, control actions are regarded as constituting unwarranted interference with natural processes. In this instance, cooperation between livestock and wildlife management needs to take the form of joint efforts to minimize mingling of susceptible livestock and wildlife species. Fencing is one approach, although this interferes with the normal migrations of several of the susceptible wildlife species, and therefore should only be done advisedly. Annual vaccination of livestock that are likely to be in regular direct or indirect contact with susceptible wildlife species is another approach.
Eradication from livestock areas not in contact with enzootic wildlife is feasible (section 8.8), given sufficient time together with unremitting efficient application of the control procedures discussed in this chapter.
8.10. Control in wildlife
While large national wildlife parks may adopt the “hands-off” management policies outlined in sections 8.3.2.2 and 8.9, this may be inappropriate for commercial or smaller parks or sustainable resource development management areas that cannot sustain the financial losses resulting from the disease. Suggested approaches to anthrax control for these are given in Annex 6, section 5.
Footnotes
- 1
Terrestrial Animal Health Code, Appendix 3.6.6. Paris: World Organisation for Animal Health (OIE); 2007. General guidance for the disposal of dead animals. (http://www
.oie.int/eng /normes/mcode/en_chapitre_3.6.6.htm)
- Introduction
- Discontinuation of infection source
- Disposal of anthrax (animal) carcasses
- Human cases: infection control in management
- Fumigation, disinfection, decontamination
- Prophylaxis
- Decisions on treatment and/or vaccination of livestock
- Duration of veterinary vaccination programmes
- Intersectoral issues: the question of eradication
- Control in wildlife
- Control - Anthrax in Humans and AnimalsControl - Anthrax in Humans and Animals
- Bacteriology - Anthrax in Humans and AnimalsBacteriology - Anthrax in Humans and Animals
Your browsing activity is empty.
Activity recording is turned off.
See more...