Introduction1
Like other ciliated protozoa, hypotrichous ciliates, to which for example the genera Euplotes, Oxytricha or Stylonychia belong, contain two morphologically and functionally different types of nuclei in one cell, the diploid micronucleus which seems to be transcriptionally almost inert and the transcriptionally very active DNA-rich macronucleus (Fig. 1). During vegetative growth micronuclei divide by conventional mitosis but the macronuclei undergo a process called amitosis, during which the nucleus divides and each daughter nucleus obtains roughly but not necessarily the same amount of DNA. When two cells of different mating types are mixed, sexual reproduction, called conjugation, takes place. A schematic diagram of the nuclear processes occuring during conjugation is shown in Figure 2. Micronuclei undergo a meiotic division, but with the exception of one all of the resulting haploid nuclei disintegrate. The remaining nucleus divides mitotically, and one of its daughter nuclei, the stationary nucleus, stays in the cell whereas the other migrates into the cytoplasm of the conjugation partner where it fuses with the stationary nucleus to form a diploid syncaryon. This syncaryon then divides mitotically and one of the daughter nuclei becomes a micronucleus while the other differentiates into a new macronucleus. During this process of macronuclear differentiation the old macronucleus degenerates.1 In the course of macronuclear differentiation in hypotrichous ciliates most extraordinary DNA-reorganization and DNA-elimination processes take place resulting in a macronucleus with a highly reduced kinetic complexity of its genome compared to the micronuclear genome. Furthermore, during this process the macronuclear genome becomes fragmented into small gene-sized DNA-molecules. Each of these molecules occurs in a defined copy number in the macronucleus, ranging from a few hundred up to 106 copies per nucleus. These molecules carry in general one open reading frame, sequences required for expression and replication, and are all terminated by telomeric sequences. Due to this very high concentration of telomeres in the macronucleus, hypotrichous ciliates became one of the model systems to study telomere sequence, structure and behaviour. In fact, the first telomere sequence from which both the 5'- and 3'-strand were sequenced came from hypotrichous ciliates,2 telomere-telomere interactions were first described in Stylonychia,3 telomere-binding proteins are well characterized in these cells4 and the catalytic telomerase protein subunit was first isolated from Euplotes.5
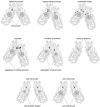
Figure 2
Schematic diagram of conjugation. For explanation see text.
In this Chapter we will first give a short overview on the molecular processes occuring during macronuclear differentiation in hypotrichous ciliates followed by a discussion of the telomere sequences of the different nuclei occuring in the cell, telomere structure, telomere-binding proteins, telomerase and of telomere behaviour during the cell cycle.
The Genome of Hypotrichous Ciliates
The DNA content of the macronucleus is between 25- and 200-fold higher than in the micronucleus. Reassociation kinetics after thermal denaturation show striking differences between macronuclear and micronuclear DNA. Micronuclear DNA reassociates as multiple DNA components, indicating the presence of repeated and nonrepeated DNA sequences and its kinetic complexity has been estimated to be in the order of 0.2–1.5 × 1012 Da. In contrast, the reassociation of macronuclear DNA follows a simple second-order reaction kinetic. Different from almost all other eukaryotic nuclei, no repetitive DNA sequences could be found in the macronucleus. The kinetic complexity of macronuclear DNA is in the order of 3 × 1010 Da, only about 10 to 14 times the complexity of the E. coli DNA. Summarizing these data, it can be concluded that the macronucleus contains only 2–5% of the DNA sequences present in the micronucleus, but each of these sequences occurs highly amplified in the macronucleus. The degree of amplification varies between different hypotrich species, being on average between about 1000 to 15,000 copies per macronucleus.6,7
Micronuclear DNA of hypotrichs is organized in about 100 to 150 small chromosomes and exists as extremely long DNA molecules probably having chromosomal size. The size of macronuclear DNA contrasts sharply with the size of micronuclear DNA. It can be resolved on agarose gels resulting in a size distribution between about 0.4 to over 20 kbp (Fig. 3) with the bulk of DNA in the 1 to 8 kbp range. Each DNA molecule occurs in a specific copy number and the DNA bands that are visible above a background smear on an agarose gel represent molecules which are more amplified than others. For example, a strong band is visible at around 7.2 kbp which represents the DNA-molecule encoding the 25S, 19S and 5.8S ribosomal RNAs occuring in a copy number of almost 106 per Stylonychia macronucleus, compared with an average of 10,000 to 15,000 for other molecules.6,7 A large number of macronuclear DNA-molecules from Oxytricha, Stylonychia and Euplotes have been sequenced,7 they all contain one (in rare cases more than one) open reading frame, leader and trailer sequences of various lengths and all are terminally flanked by short telomeric sequences (Fig. 4). These small linear DNA molecules represent a sort of naturally occurring minichromosomes, containing all DNA sequences required for expression and replication. They are associated with histones and organized as in typical eukaryotic chromatin.8,9 Upon lysis of macronuclei under low ionic conditions, small gene-sized chromatin molecules are released, each of these chromatin fragments corresponding to one gene-sized DNA-molecule.10,11 There is, however, increasing evidence that the gene-sized DNA molecules are organized in vivo in a higher chromatin structure.7,12,16 Replication of these gene-sized DNA molecules occurs in a morphological distinct region, the replication band, that forms on the inner surface of the nuclear envelope, expands across the macronucleus and migrates through it (Fig. 7).
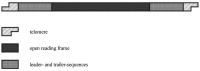
Figure 4
Schematic diagram of a macronuclear DNA molecule.
In micronuclear DNA, macronuclear precursor sequences occur in clusters separated from each other by long non-coding regions. They often contain short internal sequences (IES, internal eliminated sequences) not found in the macronucleus. In general, the exons of these precursor sequences are arranged in a linear order, however, in a significant number of genes the exons are scrambled.7,17 In no case, telomeric repeats were associated with the precursor sequences. A large percentage of the micronuclear genome consists of repetitous sequences, several of such highly repetitous sequence families have been characterized in different hypotrichs.18 Part of the micronuclear repetitous DNA sequences are families of elements resembling transposons and different classes of these transposon-like elements have been described and characterized in the micronucleus of different species.19
Conclusions: The comparison of micronuclear and macronuclear genome organization revealed that three global genomic changes take place during the conversion of a micronucleus to a macronucleus, which are DNA elimination, DNA fragmentation and amplification of DNA sequences.
Macronuclear Differentiation in Hypotrichs
The morphological events taking place during macronuclear differentiation are schematically illustrated in Figure 5.6,7,20 A few hours after the separation of the exconjugant cells, chromosomes become visible in the developing macronucleus. A rapid DNA synthesis converts these chromosomes into polytene chromosomes during the next 40 hours. Depending on the species, not all micronuclear chromosomes become polytenized but a significant percentage, up to 70 % of them become pycnotic and eventually are degraded. Thus, already at this stage some elimination of the micronuclear genome can take place. The polytene chromosomes of hypotrichs have a diversity of banding patterns very much like those of Diptera, but in addition they contain heterochromatic blocks. The total number of bands has been estimated to be between 10,000 to 15,000.20 Typical puffs have never been observed, although experimental evidence exists that low level transcription occurs in this stage.21 Bands of different sizes connected by interband fibers are also visible in electron microscopic thin sections. In spread preparations it became obvious that during the formation of the polytene chromosomes dramatic reorganization processes of the chromatin occur. In the early macronuclear anlage the chromatin appears as coiled 30-nm fibers interconnected by 10-nm fibers. In later stages the 30-nm fibers become organized into loop-like structures. These loops contain between 10 and over 200 kbp of DNA, very similar to polytene chromosomal bands of Diptera. The interband fibers contain between about 1 and 10 kbp. In these preparations the organization of the chromatid of hypotrich polytene chromosomes resembles the organization of lampbrush chromosomes observed e.g., in the meiotic prophase of amphibian oocytes or the spermatocytes of Chironomus. The degradation of polytene chromosomes becomes obvious in electron microscopic thin sections by the appearance of membraneous material surrounding the bands. Later, the bands become completely enclosed into vesicles in which DNA degradation occurs. The number of vesicles roughly corresponds to the number of bands.22 In spread preparations it could be shown that the 30-nm loops are released from the axis as chromatin rings and eventually are degraded while the 10-nm fiber is retained.12,23 The percentage of DNA eliminated during this stage correlates with the DNA content of the micronucleus in the different species. In Stylonychia over 95% of the DNA becomes eliminated, leading to a DNA-poor stage with a DNA content of the diploid micronucleus. In Euplotes, only about 80% of the DNA is eliminated leading to a DNA-poor stage with a DNA content of about 10 times of that of the micronucleus. The DNA-poor stage lasts for about 40 hours, then nucleoli and replication bands become visible in the macronuclear anlage24 and after several rounds of replication, the new macronucleus reaches its final DNA content while the old macronucleus becomes degraded during the formation of the new macronucleus.
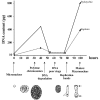
Figure 5
Schematic diagram of macronuclear differentiation in hypotrichous ciliates. Modified after Kraut et al.
Conclusions: At the molecular level, at least the following processes must occur during macronuclear differentiation: Elimination of IES and transposon-like elements, elimination of repetitive as well as unique sequences during degradation of the polytene chromosomes, specific fragmentation of the genome and in some cases reordering of exons, de novo addition of telomeric sequences and specific amplification of the gene-sized DNA molecules.
Events at the DNA Level
Excision of transposon-like elements and IES has been extensively investigated in Euplotescrassus19 but the mechanisms found in this species are obviously also found in other hypotrichs. In Euplotes elimination of transposon-like elements occurs during a discrete time period in the course of polytene chromosome formation and their excision precedes the removal of IES. Both elements show similar excision mechanisms: transposon-like elements and at least the larger IES appear as free circles following excision. The junction of these circles consists of both copies of the target site direct repeat with a few additional nucleotides between them. This suggests that excision involves staggered cuts in the chromosome, the two single-stranded overhangs are filled in and the two ends are ligated.25 The timing of IES removal has been analyzed in one precursor gene cluster in Stylonychia lemnae. The results showed that IES elimination is a highly ordered process, starting very early during polytene chromosome formation and having finished only immediately before DNA fragmentation takes place. The IES are eliminated in a specific order, in which a defined IES is only removed after complete elimination of other IES. Evidence was provided that the structure of the IES itself is not sufficient for its correct excision but that other cis-acting sequences or additional structural requirements are needed for the correct timing of IES excision. It was suggested that this ordered elimination of IES may be an essential requirement for the reordering of exons in scrambled genes.26
Considerable efforts have been made within the past years to identify cis-acting DNA sequences involved in specific DNA fragmentation and telomere addition. By sequence comparison, a 5-bp sequence was identified in Euplotes crassus, that either resides in the subtelomeric region of the macronuclear precursor sequence at position 18, or is located 12 bp from the macronuclear precursor in the flanking micronuclear-specific DNA.27,28 On the basis of the positions of the chromosome breakage sequences (E-Cbs) in Euplotes and the finding of overlapping sequences, a model for chromosome fragmentation was proposed that involves a 6-bp staggered cut on both sides of the macronuclear precursor.28 In Stylonychia lemnae no consensus sequence could be found at defined positions neither in the macronuclear precursor sequence nor in the flanking micronuclear-specific sequences.29 However, using a biological approach, i.e., injecting modified macronuclear precursor sequences and appropriate deletion constructs into the macronuclear anlagen of Stylonychia, it could be shown that the subtelomeric regions of the macronuclear precursor sequences are sufficient to direct DNA fragmentation and telomere addition. An inverted repeat with a sequence almost identical to the E-Cbs could be found in these regions, although it is not at the same position in both subtelomeric regions. An interaction between these two sequences seems to occur and to be required for processing of macronuclear precursors. In addition, a preceding transcription seems to be necessary for the subsequent fragmentation and telomere addition process.21,30 However, it is very likely, that chromatin structure and possible nuclear localization also play a crucial role in these processes.
Very little is known about the regulation of copy number control in the developing macronucleus, although some preliminary data suggest that also cis-acting sequences involved in this process are located in the subtelomeric regions of the macronuclear precursor sequences. The molecular analysis of the control of macronuclear differentiation has only just started but it can be expected that this extraordinary biological system will provide insight how a differentiating cell deals with its DNA sequences and results obtained here will not only be relevant for ciliated protozoa but very likely also for differentiating eukaryotic cells in general.
The Telomeres of Hypotrichous Ciliates
Macronuclear and Micronuclear Telomeres
Already in 1975 it became obvious that all macronuclear DNA molecules carry inverted terminal repeats: the denatured DNA molecules form single-stranded intramolecular circles when quickly renatured.31 In 1980, the 5'-strands of macronuclear DNA molecule ends were sequenced and it was shown that this terminal inverted repeat is identical in all macronuclear DNA molecules.32 Finally, Klobutcher et al2 sequenced the ends of both strands of macronuclear DNA molecules of four species of hypotrichs and showed not only that the inverted repeat is identical in all molecules but also demonstrated for the first time the presence of a 3'-overhang, a structure which is now generally believed to occur in all telomeres. However, in contrast to all other eukaryotic cells, no telomere length variation is observed in hypotrichs, suggesting a very tight telomere length control. So far, this control is not understood. The telomere sequence of Stylonychia and Oxytricha species is
5'-dC4A4C4A4 C4
3'-dG4T4G4T 4G4T4G4T 4G4
and in Euplotes aediculatus it is
5'-dC4A4C4A4 C4 A4 C4
3'-dG2T4G4T 4G4T4G4T 4G4 T 4G4
During replication, new telomeres must be synthesized by telomerase, an enzyme which is well charcterized in hypotrichs (see below). In order to explain length homogeneity of macronuclear telomeric sequences a model for replication of gene-sized DNA molecules has been proposed.33,34 In this model the 16 bases 3'-overhang serves as a template for the synthesis of a RNA primer with the sequence (5'-rC4A4)n by DNA primase. With this RNA primer DNA replication could proceed by conventional leading-strand lagging-strand mechanism. Finally, replication would be completed by the addition of 16 bases of 5'-dT4G4T4G4 by telomerase. This model is based on the assumptions that telomeres are actually origins of replication and that telomerase can add a defined number of nucleotides to the ends. Both assumptions certainly need to be verified experimentally. In any case, restoration of the correct telomere structure after replication has to be very precise and, furthermore it has to occur very fast. In an attempt to characterize telomere intermediates during replication, Stylonychia vegetative cells were synchronized and replicating molecules were labeled with BrdU using short pulse labeling. The BrdU-labeled DNA was isolated and the ends sequenced using the Maxam-Gilbert technique. In no case, a sequence different from that of unreplicating DNA molecules could be identified.35
Micronuclear telomeres of Oxytricha fallax are also composed of 3'-dG4T4-repeats. Digestion of micronuclear DNA with several restriction enzymes and subsequent probing with a telomeric sequence reveals a smear between 3 to 6 kbp, suggesting that micronuclear telomeres are heterogenous in length, similar to those of other eukaryotes. The presence of a 3'-overhang has not yet been demonstrated.36 90% of telomeric repeats are Bal31 sensitive in undigested micronuclear DNA. While some of these internal telomeric repeats are associated with transposon-like elements, this is not true for other internal telomeric squences.37–39 Replication of micronuclear telomeres follows most probably the mechanisms described for other eukaryotes.
De novo Addition of Telomeres During Macronuclear Differentiation
Macronuclear precursor sequences are not associated with telomeric sequences in the micronuclear genome, they rather have to be added de novo to the ends of newly excised macronuclear DNA molecules in the course of macronuclear differentiation. Although never experimentally demonstrated, this addition of new telomeres is probably catalyzed by telomerase as it has been described in Tetrahymena.40 Telomere addition has been most extensively analyzed in Euplotes crassus. The process of DNA fragmentation and telomere addition is most probably closely linked and must occur very fast since already in the early vesicle stage no free ends without telomeres can be detected. Initially the telomeres are several times longer than macronuclear telomeres and variable in length.41,42 Telomere shortening occurs at a defined time period during anlagen development and seems not to be linked to DNA synthesis.43 According to the present view,42,44 the G-rich strand becomes synthesized by telomerase onto the ends of the excised DNA molecules. A specific single-stranded binding protein becomes bound at the beginning of the 5' end of this strand. This protein should both protect the single-stranded DNA and set the length of the G-rich strand. After synthesis of a 14b ribonucleotide RNA primer on the G-rich strand by RNA primase, this primer becomes extended with deoxynucleotides to create the 28-bp C-rich strand. Removal of the RNA primer then will yield the 14b G-rich overhang. While the telomerase of vegetative cells can only add telomeric repeats to telomeric ends, a telomerase complex has been shown to be present in exconjugant cells that adds telomeric DNA sequences de novo to nontelomeric ends in vitro.45
Telomere DNA Structure in Hypotrichs
Like all other telomeric sequences, the 3'-strand of macronuclear telomeres is G-rich and it extends beyond the 5'-strand. Therefore it has the potential of adopting a number of alternative DNA structures and, in fact, hypotrich telomeres were used for most of the stuctural studies on telomeric sequences. The first hint that telomeres may adopt an unusual structure came from work on Stylonychia macronuclear DNA. When macronuclear DNA is incubated under increased ionic strength, only high molecular weight aggregates are visible on agarose gels. This DNA aggregation is not observed after gentle digestion with Bal31, demonstrating that terminal sequences are involved in these interactions. In the electron microscope, end-to-end aggregated DNA molecules as well as intramolecular double-stranded DNA circles can be visualized.3 Further work showed that the 3'-overhang is required for these interactions.46,47 Experimental evidence was provided that the observed intramolecular circles behave as being topologically constrained.47 The exact structure of these interactions still has to be determined.
It has been shown that the G-rich strand can adopt a variety of unusual DNA structures48 from which, however, only guanine quadruplex DNA (G-quartet) and t-loops are stable in vitro under physiological conditions.49–52 Parallel as well as antiparallel-stranded guanine quadruplex structures (Fig. 6A) have been biophysically and structurally analyzed in detail with synthetic oligonucleotides resembling the G-rich strand of hypotrichs.49,50,53,54 This four-stranded structure consists of four stacked quartets of guanine bases hydrogen bonded by Hoogsten-base pairing. The single stranded 3'-overhang can form a hairpin and two hairpins can interact to produce the G-quartet structure. Until recently, only indirect evidence for the occurrence of the G-quartet structure in vivo existed. For example, the β-subunit of the Oxytricha telomere-binding protein accelerates G-quartet formation,55 in vitro telomerase activity is greatly reduced in the presence of G-quartet56 which recently led to attempts to use this structure as a target for cancer therapy57 and in yeast, a nuclease activity that is specific for G-quartet four-stranded DNA has been identified.58 But only very recently, experimental evidence for the existence of G-quartet structure in vivo was provided.16 Antibodies were selected in vitro by ribosome display and were shown to be specific for Stylonychia telomeric G-quartet structure. Moreover, antibodies were obtained which could discriminate between the parallel and anti-parallel quadruplex structure. These antibodies were used for in situ experiments on Stylonychia nuclei. Results clearly indicate that the anti-parallel quadruplex structure occurs in the macronucleus of Stylonychia, no signal was obtained on micronuclei. The macronuclear replication band, the nuclear region where replication and telomere elongation takes place, was also not stained, suggesting that G-quartet structure becomes resolved during replication (Fig. 7).
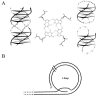
Figure 6
Schematic diagrams of telomeric DNA. (A) G-quartet structure. (B) t-loop structure. Modified after Schaffitzel et al and Murti and Prescott.
The t-loop telomere structure (Fig. 6B) has been observed at the ends of mammalian chromosomes.52 In this structure, the 3' G-rich strand folds back and invades the double-stranded region of the telomeric DNA, displacing between 75–200 nt of the G-rich strand and forming a duplex structure. Electron microscopical analysis of DNA from polytene chromosomes of Oxytricha macronuclear anlagen showed that in these nuclei telomeric DNA folds back and hybridizes with downstream telomeric sequences forming a t-loop that is stable in the absence of protein and cross-linking (Fig. 8).59 Although the structure of micronuclear telomeres has not yet been determined it is very likely that they adopt a similar structure to that observed in polytene chromosomes.

Figure 8
Electron micrograph of t-loop structure occuring in Oxytricha polytene chromosome DNA. Kindly provided by D.M. Prescott.
Conclusions: The demonstration of G-quartet structure in the macronucleus and the t-loop structure in polytene chromosomes, and possibly in the micronuclei, show that different telomeric structures occur within one cell. Furthermore, three different mechanisms of telomere capping are obviously possible and occur in hypotrichous ciliates. In the micronucleus and the polytene chromosomes, telomeres are capped with a t-loop; in the macronucleus, telomeres are capped both by telomere-binding proteins as well as by the formation of guanine quadruplex DNA.
Telomere-Binding Proteins in Hypotrichs
When macronuclei of hypotrichous ciliates are lysed in 2 M NaCl and the DNA-protein complex is purified, a major protein of about 50 KD copurifies with the DNA. It was originally demonstrated by South-Western analysis that this protein specifically binds to telomeric DNA sequences.13,60,61
Meanwhile, the telomere-binding proteins (TP) of Oxytricha and Euplotes were characterized in detail. The Oxytricha TP is a heterodimeric protein with an α-subunit of 56 KD and a β-subunit of 41 KD.60 This heterodimeric protein binds to the single-stranded, G-rich 3'-overhang.
The α-subunit is largely responsible for this DNA binding specificity but dimerization of these subunits depends on binding of both subunits to single-stranded DNA.57,62 The carboxy-terminus of the β-subunit is lysine-rich and shows some similarity to histone H1.63 This subunit greatly accelerates the formation of G-quartet in vitro and therefore could be responsible for the observed inhibition of telomerase activity by TP.56 The amino-terminal two thirds of the α-subunit are necessary and sufficient for sequence-specific DNA-binding, whereas the carboxy-terminal one third is responsible for interactions between the two subunits.65 Each subunit gene occurs in several versions in the macronucleu63with all of the α-subunit gene versions being highly scrambled.66 The heterodimeric TP of Stylonychia is highly homologous to the Oxytricha TP, showing a 79% amino acid homology in the α-subunit and 77% in the β-subunit.62,67 The Euplotes TP shows a high identity to the α-subunit of Oxytricha TP. This TP binds as a monomer of 51 KD to the 3'-single-strand telomeric overhang.68 The genes coding for the Euplotes TP have been cloned. One encodes the 51 KD protein while the other encodes a 53 KD protein.69 Whereas the 51 KD protein is located throughout the macronucleus, the 53KD protein is only present in the replication band of developing and mature macronuclei,70 suggesting that it plays a crucial role during replication. Although the regions of conservations to the 51 KD TP are located within the N-terminal section that comprises the DNA-binding domain of the TP, binding of the 53 KD TP (rTP) is much weaker than that of the 51 KD TP and it can be extracted already with 150 mM NaCl. The rTP does not preferentially bind the single-strand overhang but also recognizes the telomeric double-stranded region.71 Even though the details of protein-DNA interactions seem to be different in Euplotes and Oxytricha,68,72–74 their biological function in capping and protecting telomeres are most likely identical.
Conclusions: Proteins interacting with micronuclear telomeres or telomeres added de novo during macronuclear differentiation still need to be characterized. However, it has been shown that at the time of new telomere addition, the level of TP transcripts rises which could imply that the newly synthesized TP binds to these de novo added oversized telomeres.42,43
The Telomerase of Hypotrichs
Telomerase activity was first demonstrated in Tetrahymena 75 and in fact, most of the work on telomerase was done in this species.76,77 Telomerase is a ribonucleoprotein, the catalytic protein subunit has a reverse transcriptase-like activity, the RNA subunit contains a sequence complementary to the G-rich 3'-telomeric strand which serves as a template to elongate the 3'-telomeric strand.76
The RNA subunit of various hypotrich species has been isolated and analyzed. Most of the primary sequences differ between species but a common secondary structure for all of them has been proposed that resembles the model of Tetrahymena telomerase RNA. The only conserved regions include the template region and a short stretch of 5'-(C)UGUCA-3' which precedes the template region by two bases. The template region in Stylonychia and Oxtricha itself is circularly permuted relative to that of Euplotes. The template sequence for Oxytricha and Stylonychia is 5'-AAAACCCC-3' whereas for Euplotes it is 5'-CAAAACCC-3'.78 The gene encoding telomerase RNA is transcribed by RNA polymerase III in ciliates.40,78,80 The telomerase RNA could be localized in the macronuclear replication band of hypotrichous ciliates, demonstrating that telomere elongation takes place in this nuclear region.64
The catalytic protein subunit of telomerase was first characterized in Euplotes.81 Two protein subunits p123 and p43 were identified. Since the telomerase RNP complex has a size of 230 to 280 KD, telomerase seems to be a 1:1:1 complex of RNA:p123:p43. This complex is sufficient for the addition of telomeric repeats onto telomeric DNA primers.45,77 The p123 contains a reverse transcriptase motif in its carboxy-terminal part and shows homology to the yeast Saccharomyces EST2 gene product. The isolation of the Euplotes catalytic subunit gene encoding p123 led to the identification of the homologous genes in yeast and human. The Euplotes p43 protein subunit is associated with most or all active telomerases and shows similarity to the La autoantigen, an RNA binding protein involved in maturation of RNA polymerase III transcripts. It has been suggested that p43 may function in chaperoning the assembly and/or facilitating nuclear retention of telomerase.82
It is generally assumed that in the course of macronuclear differentiation in ciliates, telomeric sequences are added de novo to nontelomeric 3'ends.83 It has been shown in Euplotes that during macronuclear differentiation, telomerase assembles into larger complexes of 550 KD, 1600 KD and 5 MD. The 1600 KD complex as well as the 5 MD complex can add telomeric repeats de novo onto nontelomeric 3'-ends. It is assumed that these larger complexes contain a chromosome healing factor84,85 and perhaps some more as yet uncharacterized components involved in DNA fragmentation and synthesis of the 3' as well as the 5' telomeric strands.45
In Vivo Telomere Interactions and Contribution to Macronuclear Structure
The macronucleus contains millions of individual DNA molecules and it has been speculated for a long time whether this nucleus is just a sac full of genes or whether they are organized in some sort of higher order structure. Several in vitro as well as in vivo observations support this latter view. For example, in vitro telomere-telomere and telomere-protein interactions resulting in high molecular weight aggregates have been described.13 Electron microscopic studies of macronuclei spread gently on a water surface showed continuous chromatin fibers12 and after gentle treatment of macronuclei with proteinase K, rosette-like DNA structures held together by proteinous material became visible (Fig. 9A).7 Finally, antibodies directed against Z-DNA bind strongly to macronuclei. However, after gentle treatment of macronuclei with DNase I, binding no longer occurs, suggesting that macronuclear DNA molecules are organized in vivo in a topologically constrained form.14 Recently, evidence was provided that the telomere-telomere binding protein complex specifically interacts with components of the nuclear matrix and that this interaction is mediated by the telomere-binding protein. In the course of replication, the interaction of TP with components of the nuclear matrix is resolved and an attachment of the telomeres to the nuclear matrix no longer occurs.15
Taking all data together, the following picture emerges for telomere interactions occuring in the macronucleus (summarized in Fig. 9B): Macronuclear telomeres of individual DNA-molecules can interact with each other by G-quartet formation,16 the formation of this structure is enhanced and stabilized by TP.55 These rosette-like structures are attached to the nuclear matrix by the specific interaction of TP with components of the nuclear matrix. In the course of replication, this interaction is resolved and individual molecules can be replicated in their linear form.15
Conclusions: Hypotrichous ciliates were one of the first model systems for the study of telomere structure and behavior. As outlined above, relevant observations made in these organisms were used to trace telomere behaviour in other species, including human. A number of important questions regarding telomere metabolism still need to be solved and it can be assumed that the study of telomere behaviour in hypotrichous ciliates will provide further insight into these questions.
Acknowledgments
Work in our laboratory was supported by the Deutsche Forschungsgemeinschaft, the National Science Foundation and the Alfried Krupp von Bohlen and Halbach foundation. We thank Jan Postberg for help with the figures and all colleagues working in this field for providing us with material.
References
- 1.
- Grell KG. Protozoology. Berlin: Springer-Verlag. 1973
- 2.
- Klobutcher LA, Swanton MT, Donini P, Prescott DM. All gene-sized DNA molecules in four species of hypotrichs have the same terminal sequence and an unusual 3' terminus. Proc Natl Acad Sci USA. 1981;78:3015–3019. [PMC free article: PMC319490] [PubMed: 6265931]
- 3.
- Lipps HJ. In vitro aggregation of the gene-sized DNA molecules of the ciliate Stylonychia mytilus. Proc Natl Acad Sci USA. 1980;77:4104–4107. [PMC free article: PMC349778] [PubMed: 6776521]
- 4.
- Price CM. Telomeres. Capping of the ends. Nature. 1999;397:213–214. [PubMed: 9930692]
- 5.
- Lingner J, Cech TR. Purification of telomerase from Euplotes aediculatus: requirements of a primer 3' overhang. Proc Natl Acad Sci USA. 1996;93:10712–10717. [PMC free article: PMC38220] [PubMed: 8855245]
- 6.
- Kraut H, Lipps HJ, Prescott DM. The genome of hypotrichous ciliates. Int Rev Cytol. 1986;99:1–28. [PubMed: 3082786]
- 7.
- Prescott DM. The DNA of ciliated protozoa. Microbiol Rev. 1994;58:233–267. [PMC free article: PMC372963] [PubMed: 8078435]
- 8.
- Lipps HJ, Morris NR. Chromatin structure in the nuclei of the ciliate Stylonychia mytilus. Biochem Biophys Res Commun. 1977;74:230–234. [PubMed: 402131]
- 9.
- Lawn R, Heumann JM, Herrick G, Prescott DM. The gene-sized DNA molecules of Oxytricha. Cold Spring Harbor Symp Quant Biol. 1978;42:483–492. [PubMed: 98283]
- 10.
- Lipps HJ, Nock A, Riewe M, Steinbrück G. Chromatin structure in the macronucleus of the ciliate Stylonychia mytilus. Nucleic Acids Res. 1978;5:4699–4709. [PMC free article: PMC342782] [PubMed: 106365]
- 11.
- Butler AP, Laughlin TJ, Cadilla CL, Henty JM, Olins DE. Physical structure of the gene-sized chromatin from the protozoan Oxytricha. Nucleic Acids Res. 1984;12:3201–3217. [PMC free article: PMC318739] [PubMed: 6718249]
- 12.
- Meyer GF, Lipps HJ. The formation of polytene chromosomes during macronuclear development of the hypotrichous ciliate Stylonychia mytilus. Chromosoma. 1981;82:309–314. [PubMed: 6785046]
- 13.
- Lipps HJ, Gruissem W, Prescott DM. Higher order DNA structure in macronuclear chromatin of the hypotrichous ciliate Oxytricha nova. Proc Natl Acad Sci USA. 1982;79:2495–2499. [PMC free article: PMC346225] [PubMed: 6806811]
- 14.
- Lipps HJ, Nordheim A, Lafer EM, Ammermann D, Stollar BC, Rich A. Antibodies against Z DNA react with the macronucleus but not the micronucleus of the hypotrichous ciliate Stylonychia mytilus. Cell. 1983;32:435–441. [PubMed: 6218888]
- 15.
- Postberg J, Juranek SA, Feiler S, Kortwig H, Jönsson F, Lipps HJ. Association of the telomere-telomere binding protein-complex of hypotrichous ciliates with the nuclear matrix and dissociation during replication. J Cell Sci. 2001;114:1861–1866. [PubMed: 11329372]
- 16.
- Schaffitzel C, Berger I, Postberg J, Hanes J, Lipps HJ, Plückthun A. In vitro generated antibodies specific for telomeric guanine quadruplex DNA react with Stylonychia lemnae macronuclei. Proc Natl Acad Sci USA. 2001;98:8572–8577. [PMC free article: PMC37477] [PubMed: 11438689]
- 17.
- Landweber LF, Kuo TC, Curtis EA. Evolution and assembly of an extremely scrambled gene. Proc Natl Acad Sci USA. 2000;97:3298–3303. [PMC free article: PMC16233] [PubMed: 10725348]
- 18.
- Lipps HJ, Eder C. Macronucleus structure and macronucleus development in hypotrichous ciliates. Int J Dev Biol. 1996;40:141–147. [PubMed: 8735923]
- 19.
- Klobutcher LA, Jahn CL. Develomentally controlled genomic rearrangements in ciliated protozoa. Curr Opin Genet Dev. 1991;1:397–403. [PubMed: 1668650]
- 20.
- Ammermann D. Morphology and development of the macronuclei of the ciliates Stylonychia mytilusand Euplotes aediculatus. Chromosoma. 1971;33:209–238. [PubMed: 4996055]
- 21.
- Jönsson F, Wen JP, Fetzer CP, Lipps HJ. A subtelomeric DNA sequence is required for correct processing of the macronuclear DNA sequences during macronuclear development in the hypotrichous ciliate Stylonychia lemnae. Nucleic Acids Res. 1999;27:2832–2841. [PMC free article: PMC148495] [PubMed: 10390523]
- 22.
- Kloetzel JA. Compartmentalization of the developing macronucleus following comjugation in Stylonychia and Euplotes. J Cell Biol. 1970;47:395–407. [PMC free article: PMC2108083] [PubMed: 19866739]
- 23.
- Meyer GF, Lipps HJ. Chromatin elimination in the hypotrichous ciliate Stylonychia mytilus. Chromosoma. 1980;77:285–297. [PubMed: 6768532]
- 24.
- Maercker C, Harjes P, Neben M, Niemann H, Sianidis G, Lipps HJ. The formation of new nucleoli during macronuclear development of the hypotrichous ciliate Stylonychia lemnae visualized by in situ hybridization. Chromosome Res. 1997;5:333–335. [PubMed: 9292238]
- 25.
- Klobutcher LA, Turner LR, LaPlante ME. Circular forms of developmentally excised DNA in Euplotes crassus have a heteroduplex junction. Genes Dev. 1993;7:84–94. [PubMed: 8422990]
- 26.
- Wen JP, Maercker C, Lipps HJ. Sequential excision of internal eliminated DNA sequences in the differentiating macronucleus of the hypotrichous ciliate Stylonychia lemnae. Nucleic Acids Res. 1996;24:4415–4419. [PMC free article: PMC146267] [PubMed: 8948632]
- 27.
- Baird SE, Klobutcher LA. Characterization of chromosome fragmentation in two protozoans and identification of a candidate fragmentation sequence in Euplotes crassus. Genes Dev. 1989;3:585–597. [PubMed: 2744456]
- 28.
- Klobutcher LA. Characterization of in vivo developmental chromosome fragmentation intermediates in E. crassus. Mol Cell. 1999;4:695–704. [PubMed: 10619017]
- 29.
- Maercker C, Lipps HJ. Analysis of the subtelomeric regions of the suntelomeric regions of the macronuclear gene-sized DNA-molecules from the hypotrichous ciliate Stylonychia lemnae. Implications for the DNA fragmentation process during macronuclear development? Dev Genet. 1993;14:378–384. [PubMed: 8293579]
- 30.
- Jönsson F, Steinbrück G, Lipps HJ. Both subtelomeric regions are required and sufficient for DNA fragmentation during macronuclear development in Stylonychia lemnae. Genome Biol. 2001;2:00051–000511. [PMC free article: PMC25775] [PubMed: 11182888]
- 31.
- Wesley RD. Inverted repetitous sequences in the macronuclear DNA of hypotrichous ciliates. Proc Natl Acad Sci USA. 1975;72:678–682. [PMC free article: PMC432378] [PubMed: 804696]
- 32.
- Oka Y, Susumu S, Nakai S, Nishida Y, Okubo S. Inverted terminal repeat sequence in the macronuclear SNA of Stylonychia pustulata. Gene. 1980;10:301–306. [PubMed: 6776007]
- 33.
- Zahler AM, Prescott DM. Telomere terminal transferase activity in the hypotrichous ciliate Oxytricha nova and a model for replication of the ends of linear DNA molecules. Nucleic Acids Res. 1988;16:6953–6972. [PMC free article: PMC338344] [PubMed: 3136437]
- 34.
- Zahler AM, Prescott DM. DNA primase and the replication of the telomeres in Oxytricha nova. Nucleic Acids Res. 1989;17:6299–6317. [PMC free article: PMC318279] [PubMed: 2475856]
- 35.
- Juranek S, Jönsson F, Maercker C, Lipps HJ. The telomeres of replicating macronuclear DNA molecules of the ciliate Stylonychia lemnae. Protistology. 2000;1:148–151.
- 36.
- Dawson D, Herrick G. Telomeric properties of C4A4-homologous sequences in micronuclear DNA of Oxytricha fallax. Cell. 1984;36:171–177. [PubMed: 6319006]
- 37.
- Dawson D, Herrick G. Rare internal C4A4 repeats in the micronuclear genome of Oxytricha fallax. Mol. Cell Biol. 1984;4:2661–2667. [PMC free article: PMC369275] [PubMed: 6098818]
- 38.
- Hunter DJ, Williams K, Cartinhour S, Herrick G. Precise excision of telomere-bearing transposons during Oxytricha fallaxmacronuclear development. Genes Dev. 1989;3:2101–2112. [PubMed: 2560753]
- 39.
- Stoll S, Zirlik T, Maercker C, Lipps HJ. The organization of internal telomeric repeats in the polytene chromosomes of the hypotrichous ciliate Stylonychia lemnae. Nucleic Acids Res. 1993;21:1783–1788. [PMC free article: PMC309415] [PubMed: 8493097]
- 40.
- Yu GL, Blackburn EH. Developmentally programmed healing of chromosomes by telomerase in Tetrahymena. Cell. 1991;67:823–832. [PubMed: 1934071]
- 41.
- Roth M, Prescott DM. DNA intermediates and telomere addition during genome reorganization in Euplotes crassus. Cell. 1985;41:411–417. [PubMed: 2985276]
- 42.
- Vermeesch JR, Price C. Telomeric DNA sequence and structure following de novo telomere synthesis in Euplotes crassus. Mol Cell Biol. 1994;14:554–566. [PMC free article: PMC358405] [PubMed: 8264623]
- 43.
- Vermeesch JR, Williams D, Price C. Telomere processing in Euplotes. Nucleic Acids Res. 1993;21:5366–5371. [PMC free article: PMC310572] [PubMed: 8265350]
- 44.
- Fan X, Price CM. Coordinate regulation of G- and C-strand length during new telomere synthesis. Mol Biol Cell. 1997;8:2145–2155. [PMC free article: PMC25698] [PubMed: 9362059]
- 45.
- Greene EC, Shippen DE. Developmentally programmed assembly of higher order telomerase complexes with distinct biochemical and structural properties. Genes Dev. 1998;12:2921–2931. [PMC free article: PMC317169] [PubMed: 9744868]
- 46.
- Oka Y, Thomas C A Jr. The cohering telomeres of Oxytricha. Nucleic Acids Res. 1987;15:8877–8898. [PMC free article: PMC306411] [PubMed: 3120149]
- 47.
- Lipps HJ, Feiler S, Azorin F. Telomeric interactions result in the formation of intramolecular circles behaving as topologically constrained. J. Mol. Biol. 1998;283:1–7. [PubMed: 9761668]
- 48.
- Henderson E. Telomere DNA structureIn: Blackburn,E.H., Greider, C.W., eds.Telomeres Cold Spring Harbor: CSH Laboratory Press199511–34.
- 49.
- Sen D, Gilbert W. Formation of parallel four-stranded complexes by guanine-rich motifs in DNA and its implications for meiosis. Nature. 1988;334:364–366. [PubMed: 3393228]
- 50.
- Sundquist WI, Klug A. Telomeric DNA dimerizes by formation of guanine tetrads between hairpin loops. Nature. 1989;342:825–829. [PubMed: 2601741]
- 51.
- Williamson JR, Raghuraman MK, Cech TR. Monovalent cation-induced structure of telomeric DNA: the G-quartet model. Cell. 1989;59:871–880. [PubMed: 2590943]
- 52.
- Griffith JD, Comeau L, Rosenfield S, Stansel RM, Bianchi A, Moss H, DeLange T. Mammalian telomeres end in a large duplex loop. Cell. 1999;97:503–514. [PubMed: 10338214]
- 53.
- Kang C, Zhang X, Ratliff R, Moyzis R, Rich A. Crystal structure of four-stranded Oxytricha telomeric DNA. Nature. 1982;356:126–131. [PubMed: 1545863]
- 54.
- Smith FW, Feigon J. Quadruplex structure of Oxytricha telomeric DNA oligonucleotides. Nature. 1992;356:164–168. [PubMed: 1545871]
- 55.
- Fang G, Cech TR. The ß subunit of Oxytricha telomere-binding protein promotes G-quartet formation by telomeric DNA. Cell. 1993;74:875–885. [PubMed: 8374954]
- 56.
- Zahler AM, Williamson JR, Cech TR, Prescott DM. Inhibition of telomerase by G-quartet DNA structures. Nature. 1991;350:718–720. [PubMed: 2023635]
- 57.
- Han H, Hurley LH. G-quadruplex DNA: a potential target for anti-cancer drug design. Trends Pharmacol Sci. 2000;21:136–142. [PubMed: 10740289]
- 58.
- Liu Z, Frantz JD, Gilbert W, Tye BK. Identification and characterization of a nuclease activity specific for G4 tetrastranded DNA. Proc Natl Acad Sci USA. 1993;90:3157–3161. [PMC free article: PMC46258] [PubMed: 8475054]
- 59.
- Murti KG, Prescott DM. Telomeres of polytene chromosomes in a ciliated protozoan terminate in duplex DNA loops. Proc Natl Acad Sci USA. 1999;96:14436–14439. [PMC free article: PMC24454] [PubMed: 10588723]
- 60.
- Gottschling DE, Zakian VA. Telomere proteins: specific recognition and protection of the natural termini of Oxytricha macronuclear DNA. Cell. 1986;47:195–205. [PubMed: 3094961]
- 61.
- Steinhilber W, Lipps HJ. A telomere binding protein in the macronuclei of the hypotrichous ciliate Stylonychia lemnae. FEBS Lett. 1987;206:25–28.
- 62.
- Fang G, Cech TR. (1993) Oxytricha telomere-binding protein: DNA-dependent dimerization of the a and ß subunits. Proc Natl Acad Sci USA. 1987;90:6056–6060. [PMC free article: PMC46866] [PubMed: 8327484]
- 63.
- Hicke BJ, Celander DW, MacDonald GH, Price CM, Cech TR. Two versions of the gene encoding the 41 kilodalton subunit of the telomere binding protein of Oxytricha nova. Proc Natl Acad Sci USA. 1990;87:1481–1485. [PMC free article: PMC53499] [PubMed: 1689486]
- 64.
- Fang G, Cech TR. Telomerase RNA localized in the replication band and spherical subnuclear organelles in hypotrichous ciliates. J Cell Biol. 1995;130:243–253. [PMC free article: PMC2199938] [PubMed: 7615628]
- 65.
- Fang G, Gray JT, Cech TR. Oxytricha telomere-binding protein: Separable DNA-binding and dimerization domains of the a subunit. Genes Dev. 1993;7:870–882. [PubMed: 8491383]
- 66.
- Mitcham JL, Lynn AJ, Prescott DM. Analysis of a scrambled gene: the gene encoding a-telomere-binding protein in Oxytricha nova. Genes Dev. 1992;6:788–800. [PubMed: 1577273]
- 67.
- Fang G, Cech TR. Molecular cloning of telomere-binding protein genes from Stylonychia mytilis. Nucleic Acids Res. 1991;19:5515–5518. [PMC free article: PMC328950] [PubMed: 1945829]
- 68.
- Price CM, Skopp R, Krueger J, Williams D. DNA recognition and binding by the Euplotes telomere protein. Biochemistry. 1992;31:10835–10843. [PubMed: 1420196]
- 69.
- Wang W, Skopp R, Scofield M, Price CM. Euplotes crassus has multiple genes encoding telomere-binding proteins and telomere-binding protein homologs. Nucleic Acids Res. 1992;20:6621–6629. [PMC free article: PMC334579] [PubMed: 1480483]
- 70.
- Skopp R, Wang W, Price CM. rTP: a candidate telomere protein that is associated with DNA replication. Chromosoma. 1996;105:82–91. [PubMed: 8753697]
- 71.
- Carlson DL, Skopp R, Price CM. DNA-binding properties of the replication telomere protein. Biochemistry. 1997;36:15900–15908. [PubMed: 9398323]
- 72.
- Price CM. Telomere structure in Euplotes crassus: Characterization of DNA-protein interactions and isolation of a telomere-binding protein. Mol Cell Biol. 1990;10:3421–3431. [PMC free article: PMC360778] [PubMed: 2355912]
- 73.
- Price CM, Cech TR. Telomeric DNA-protein interactions of Oxytricha macronuclear DNA. Genes Dev. 1987;1:783–793. [PubMed: 3123321]
- 74.
- Price CM, Cech TR. Properties of the telomeric DNA-binding protein from Oxytricha nova. Biochemistry. 1989;28:769–774. [PubMed: 2713343]
- 75.
- Greider CW, Blackburn EH. Identification of a specific telomere terminal transferase activity in Tetrahymena extracts. Cell. 1985;43:405–413. [PubMed: 3907856]
- 76.
- Greider CW. Telomerase biochemistry, regulation In: Blackburn, E.H., Greider, C.W., eds.Telomeres Cold Spring Harbor: CSH Laboratory Press199535–68.
- 77.
- Lingner J, Cech TR. Telomerase and chromosome end maintenance. Curr Opin Genet Dev. 1998;8:226–232. [PubMed: 9610414]
- 78.
- Lingner J, Hendrick LL, Cech TR. Telomerase RNAs of different ciliates have a common secondary structure and permuted template. Genes Dev. 1994;8:1984–1998. [PubMed: 7958872]
- 79.
- Greider CW, Blackburn EH. A telomeric sequence in the RNA of Tetrahymena telomerase required for telomere repeat synthesis. Nature. 1989;337:331–337. [PubMed: 2463488]
- 80.
- Shippen-Lentz D, Blackburn EH. Functional evidence for an RNA template in telomerase. Science. 1990;247:546–552. [PubMed: 1689074]
- 81.
- Lingner J, Hughes TR, Shevchenko A, Mann A, Lundblad V, Cech TR. Reverse transcriptase motifs in the catalytic subunit of telomerase. Science. 1997;276:561–567. [PubMed: 9110970]
- 82.
- Aigner S, Lingner J, Goodrich KJ, Grosshans CA, Shevechenko A, Mann M, Cech TR. Euplotes telomerase contains an La motif protein produced by apparent translational frameshifting. EMBO J. 2000;19:6230–6239. [PMC free article: PMC305813] [PubMed: 11080168]
- 83.
- Melek M, Shippen DE. Chromosome healing: spontaneous and programmed de novo telomere formation by telomerase. BioEssays. 1996;18:301–308. [PubMed: 8967898]
- 84.
- Bednenko J, Melek M, Greene EC, Shippen DE. Developmentally regulated initiation of DNA synthesis by telomerase: evidence for factor-assisted de novo telomere formation. EMBO J. 1997;16:2507–2518. [PMC free article: PMC1169850] [PubMed: 9171363]
- 85.
- Bednenko J, Melek M, Shippen DE. Reiterative dG addition by Euplotes crassus telomerase during extension of non-telomeric DNA. Nucleic Acids Res. 1998;26:3998–4004. [PMC free article: PMC147809] [PubMed: 9705511]
Footnotes
- *
Recently, the hypotrichous ciliates were reclassified into hypotrichs (e.g., Euplotes) and stichotrichs (e.g., Oxytricha and Stylonchia).
Publication Details
Author Information and Affiliations
Authors
Franziska Jonsson and Hans J. Lipps.Copyright
Publisher
Landes Bioscience, Austin (TX)
NLM Citation
Jonsson F, Lipps HJ. The Biology of Telomeres in Hypotrichous Ciliates. In: Madame Curie Bioscience Database [Internet]. Austin (TX): Landes Bioscience; 2000-2013.