NCBI Bookshelf. A service of the National Library of Medicine, National Institutes of Health.
Madame Curie Bioscience Database [Internet]. Austin (TX): Landes Bioscience; 2000-2013.
Introduction
Telomerase is a ribonucleoprotein enzyme that maintains telomeres; the ends of eukaryotic chromosomes. Human telomerase synthesizes TTAGGG tandem repeat sequences at the 3' end of the chromosomes using part of its internal RNA moiety as a template.1 The discovery that telomerase expression closely correlates with cell immortalisation and malignant progression has led to considerable interest. Telomerase activity has been found in the vast majority of human tumors (>85%) including the most prevalent cancers that include prostate, colon, lung, breast, ovary and pancreas.2,3 Conversely, telomerase activity is below detection levels in most somatic cells with the exception of cells with high replication potential such as germ cells, epithelial cells, lymphocytes and stem cells.2,3 By maintaining the telomere length of tumor cells, telomerase provides a mechanism for sustaining indefinite replicative capacity4 which is necessary to support tumour growth.
Identifying a universal target for cancer treatment is a significant challenge for modern medicine. Since telomerase appears to be a hallmark of the majority of cancerous cells, it has become a novel and potentially selective target for cancer chemotherapy. The rational for exploring telomerase as a therapeutic target is that disruption of telomerase activity would prevent cells from maintaining their telomeres and thus leads to telomere shortening. Critical telomere shortening would lead to cellular senescence and chromosome instability eventually resulting in cell death and tumor stasis or regression (Fig. 1).5
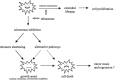
Figure 1
Effects of telomerase inhibition on human tumor cell growth. The possible activation of alternative pathways for telomere maintenance (see Chapter by Reddel) is indicated.
To exploit the potential of telomerase as a therapeutic target for chemotherapy, the development of potent and selective inhibitors is required. In the past decade, a variety of experimental approaches have been used to test and validate telomerase as an anti-cancer target. For example the dominant negative expression of human telomerase reverse transcriptase6,7,8 (hTERT) and the expression of non-functional human telomerase RNA9,10 (hTR) has been successfully exploited in various tumor cell lines. These studies resulted in inhibition of telomerase activity, telomere shortening and loss of cell viability. Various chemical approaches have also been explored. These have focused on three main areas as follows: the design and development of small molecules which inhibit the reverse transcriptase activity of telomerase11,12 (i.e., azidothymidine and other nucleoside triphosphate analogues), small molecules that stabilise G-quadruplex structure of the telomeres,13,14 and “antisense” inhibition of human telomerase by oligonucleotide analogues. It is the latter approach which is the focus of this Chapter.
The “Antisense” Approach to Telomerase Inhibition
The antisense approach normally refers to the inactivation of gene expression at the transcriptional level typically using a short oligonucleotide or mimic to target a complementary sequence of the corresponding messenger RNA (mRNA).15,16 For telomerase, the “antisense” terminology has referred mainly to the targeting of defined sequences within the RNA subunit of human telomerase (hTR) by complementary oligonucleotides. “Normal” antisense targeting of telomerase (i.e., mRNA of hTERT as target) has been reported17,18 but will not be featured in this article.
The RNA subunit of human telomerase is made up of 451 ribonucleotides and contains an 11 nucleotide (nt) single-stranded region known as the template region, of sequence 5'-CUAACCCUAAC-3' located from position 46 to 56.9,19 The numbering of telomerase hTR used has been based on the sequence described by Feng et al.9 In the proposed model for DNA synthesis catalysed by telomerase, the template sequence has two roles (Fig. 2).20,21 The alignment domain (nt 46 to 50) of the template hybridises to the 3' end of the telomere via Watson-Crick base pairing interactions. The telomere is subsequently extended by reverse transcription of the last six nucleotides of the template (nt 51 to 56), sometimes referred to as the elongation domain.22 The template region of telomerase is, therefore, accessible for hybridization with complementary nucleic acids and essential for templating telomeric DNA synthesis. Consequently, it has become the target region of choice for oligonucleotide-based inhibitors of human telomerase.
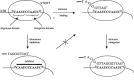
Figure 2
The mechanism of telomeric DNA synthesis catalysed by human telomerase.
Oligonucleotides with sequence complementarity to the template region of human telomerase can hybridise and thus compete with the 3' end of telomeric DNA for telomerase. By blocking access to the active site of the enzyme such molecules are capable of preventing telomere elongation (Fig. 2). An advantage of this approach is the potential affinity and selectivity that oligonucleotide mimics have for targeting nucleic acid. In the absence of significant structural information for telomerase, the primary structure of hTR from the work of Villeponteau and co-workers provides sufficient information to design oligonucleotide-based telomerase inhibitors.9
To be useful therapeutic agents, oligonucleotides must fulfill a number of criteria that include: high sequence specificity, in vivo stability, uptake and retention by target cells and a viable chemical synthesis on the required scale. Furthermore, anti-telomerase oligonucleotides that target the template region must bind with a very high affinity per base pair in order to compensate for the limited number of continuous bases accessible on the RNA template.23,24 Corey and co-workers have evaluated the relative potency of a series of PNA oligomers overlapping both the template region of hTR and its 3' or 5' vicinity (spanning nt 36 to 67). These studies have shown that it is principally the template region and 4 nucleotides downstream and upstream of the template (nt 42 to 45 and 57 to 60 respectively) which are the most potent target sites for inhibition.23,24 In particular, the four cytosines C50-C52, C56 of the template sequence and to a lesser extent bases U53, U57, A54 play an important role in the recognition and binding of complementary sequences.24 In the same study, the authors also demonstrated that the potency of PNA oligomers complementary to the template region and its 3' vicinity increases with the length of the PNA inhibitors. Optimal inhibitory effects on telomerase activity were observed with PNA 11 to 13 bases in length of sequence 5'-TAGGGTTAGAC(AA)-3'.24 These PNAs inhibited telomerase activity with a comparable IC50 value of 10 nM. In contrast, shorter PNAs (6 to 10-mer) were typically 1,000 to 10,000-fold less potent than longer PNAs. Furthermore, the 15-mer of sequence 5'-GTTAGGGTTAGACAA-3', did not exert significantly stronger inhibitory effects.24
Oligonucleotide Analogues as Human Telomerase Inhibitors
Natural oligonucleotides (DNA, RNA) have limited potential as therapeutic agents, the main reason being their poor in vivo stability as a result of the phosphodiester backbone's susceptibility to enzymatic cleavage by cellular nucleases.25 To overcome this general problem in the antisense field, there have been significant advances in the discovery and development of analogues bearing structural features which improve the pharmacological properties of natural oligonucleotides. Several of these oligonucleotide analogues have been tested as telomerase inhibitors. The most potent include phosphorothioate DNA (PS-DNA), 2'-O-alkyl RNA and peptide nucleic acids (PNA) (Fig. 3).
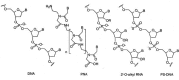
Figure 3
Chemical backbone structure of DNA analogues that are the most potent inhibitors of telomerase. (Alkyl is methyl for 2'-OMe RNA and 2-methoxyethyl (C2H4OCH3) for 2'-OMOE RNA). B= nucleobase (A, T, G, C).
PS-DNA is prepared by the modification of the phosphodiester backbone linkage of DNA by replacement of one non-bridging phosphate oxygen atom by sulphur.26 Relative to DNA, PS-DNA offers several potential advantages including increased affinity and improved resistance to nuclease degradation.27,28 However, PS-DNA can also interact with proteins in a non-specific manner with affinities typically in the micromolar to millimolar range,29,30 which represents a possible limitation. One PS-DNA oligomer is currently used as an antisense drug to treat CMV retinitis31 and several other examples have entered clinical trials for other disease areas.30,32 2'-O-alkyl RNA belongs to a class of oligonucleotide mimics with structural modification at the 2' position of the ribose sugar of RNA.33 2'-O-methyl RNA (2'-OMe RNA) and 2'-O-(2-methoxyethyl) RNA (2'-OMOE RNA) are examples of such a modification (Fig. 3). 2'-O-alkyl RNA binds complementary nucleic acid sequences with higher affinity than analogous DNA or RNA,33,34 and have been shown to display greater oral bioavailability in vivo.35 2'-O-alkyl RNA oligomers are also currently being evaluated in clinical trials.36 PNA is a peptide-nucleic acid hybrid in which the phosphate backbone has been replaced by an achiral and neutral backbone made from N-(2-aminoethyl)-glycine units (Fig. 3). The nucleobases A, G, T, C are attached to the amino nitrogen on the backbone via methylenecarbonyl linkages.37 The use of PNA as antisense agents provides several benefits.37,38 PNA oligomers bind complementary sequence with high affinity relative to analogous DNA or RNA.38 PNA/DNA or PNA/RNA hybrids also exhibit higher thermal stability compared to the corresponding DNA/DNA or DNA/RNA duplex.38 This is generally ascribed to the lack of electrostatic repulsion between the neutral PNA backbone and the negatively charged phosphodiester backbone of nucleic acids. Furthermore, PNA displays high sequence specificity.38 PNA also possesses high chemical and biological stability, due to the unnatural amide backbone that is not recognized by nucleases or proteases.39
Inhibition of Telomerase Activity by Antisense Oligonucleotides Directed Against the RNA Template
Several studies have shown that 2'-O-alkyl RNA, PS-DNA and PNA complementary to the template region of telomerase can disrupt human telomerase activity in vitro with IC50 values in the low nanomolar range for the best inhibitors. These data have been summarized in Table 1.
Table 1
Reported oligonucleotide-based inhibition of human telomerase activity.
Natural oligonucleotides (DNA) have been shown to inhibit telomerase activity in ciliate,20 mice40 and human cells.41 However, high concentrations (typically micromolar) and long sequences (typically >18-mer) were required. Shorter oligonucleotides (13-mer) were found to be ineffective.42 In comparison, PS-DNA is a more potent inhibitor of human telomerase. However, independent studies by Corey et al and Lehmann et al have demonstrated that PS-DNA are 40 to 200 fold less potent inhibitors of telomerase activity than the analogous PNA (Table 1).23,43 For instance the 13-mer PS-DNA 1 of sequence 5'-TAGGGTTAGACAA-3' overlapping the 11 nt template and 2 nt downstream possess an IC50 value of 200 nM whereas the PNA analogue (PNA 2, Table 2) inhibits telomerase activity with a substantially lower IC50 of 1 nM.44 Stronger inhibitory effects were achieved with PS-DNA 15 nucleotides or longer.43 For example, PS-DNA 6 (Table 2) has an IC50 value of 0.5 nM. Inhibition of human telomerase by PS-DNA oligomers is also subject to lower sequence selectivity than PNA.23,43 For instance the non-complementary 13-mer PS-DNA 3 and 15-mer PS-DNA 7 of scrambled sequence inhibited telomerase activity with IC50 values only 2 to 3 fold higher (450 nM and 1.3 nM respectively, Table 2) than complementary PS-DNA 1 and PS-DNA 6 (200 nM and 0.5 nM, respectively).23,43 The mode of action of PS-DNA still remains to be elucidated but their propensity to bind to proteins suggest hTERT or telomerase-associated proteins as putative targets. In contrast, PNA displays high sequence specificity. The 13-mer PNA 5 (Table 2) which contained 4 mismatches relative to the template is 1000-fold less active than complementary PNA 2 whereas PNA 4 of scrambled sequence showed no detectable inhibition.44 These results demonstrate that the use of PNA provides important benefits over PS-DNA.
Table 2
Specificity and potency of human telomerase inhibition in vitro by template-targeting peptide nucleic acid (PNA) and phosphorothioate DNA (PS-DNA).
Only one class of oligonucleotide-based inhibitors of human telomerase with potencies superior to PNA has been published.42,45 Corey and co-workers have made a direct comparison between the relative potency of 2'-O-alkyl RNA and PNA homologues to inhibit telomerase activity (Table 3).42 13 base 2'-O-alkyl RNAs complementary to the template of telomerase and 2 nucleotides upstream (nt 46 to 58) inhibited telomerase with IC50 values of 8 nM and 10 nM for 2'-OMe RNA 8 and 2'-OMOE RNA 9 respectively (Table 3). 42,45 Inhibition by the analogous 13-mer PNA gave an IC50 value of 20 nM (PNA 10, Table 3). 2'-O-alkyl RNA also shows high sequence selectivity. For example 2'-OMOE RNA 13 with 2 mismatches within the sequence was 1000 fold less active than complementary 2'-OMOE RNA 9 (Table 3).45 The incorporation of 4 mismatches within the sequence completely abolishes the inhibitory effect (2'-OMe RNA 12, Table 3). It is interesting to note that 2'-O-alkyl RNA 8 and PNA 10 (Table 3) inhibit telomerase with IC50 values of 8 nM and 20 nM respectively whereas the Tm values for target RNA are 58°C and 76°C respectively.42 This illustrates that for antisense inhibitors of telomerase, high target affinity does not necessarily correlate with higher potency.
Table 3
Specificity and potency of human telomerase inhibition in vitro by template-targeting 2'-OMe RNA, 2'-OMOE RNA, PNA and DNA.
PNA and 2'-OMeRNA are promising lead candidates for in vivo investigation, combining potency, sequence selectivity and minimized size. Despite having relatively poor sequence specificity, PS-DNA may still target telomerase selectively enough to be used as a lead compound. Although each of the above oligonucleotide mimics have significantly improved the properties of natural oligomers, none have entirely fulfilled the requisite criteria for therapeutics. Therefore a combination of chemical modification approaches may have to be used in order to optimise their benefits.46 One form of modification that has been explored is the combination of 2'-O-alkyl RNA with PS-DNA linkages. The terminal phosphorothioate linkage has been used to cap 2'-O-alkyl RNA and generate improved inhibitors. The presence of phosphorothioate linkages at both end of 2'-O-alkyl RNA confer enhanced resistance to nucleases whilst maintaining the high specificity and potency of 2'-O-alkyl RNA (Table 3, 2'-OMe RNA 14, 2'-OMOE RNA 16). Mismatch sequences (2'-OMe RNA 15, 2'-OMOE RNA 17) still possess IC50 values at least 1000-fold less potent than the corresponding matched sequences.42,45 In contrast, the systematic substitution of all the phosphodiester linkages by phosphorothioates substantially reduces the sequence selectivity of these inhibitors.42,45 The potency of these chimeras in vitro is improved with IC50 values of 7 nM (i.e., 2'-OMOE RNA 20) but mismatch sequences are also potent inhibitors becoming only 25- to 100-fold less active than complementary and unmodified 2'-O-alkyl RNA (2'-OMe RNA 19, 2'-OMOE RNA 21, Table 3).
Inhibition of Telomerase by Non-Template Directed Oligonucleotides
Early in vitro studies suggested that PNA targeted to sequences of hTR outside the template region were relatively poor inhibitors of telomerase activity. More recently, however, Corey and co-workers demonstrated that PNA complementary to structurally and functionally important sequences of hTR represent a new and potentially valuable class of telomerase inhibitors.47The ability to reconstitute telomerase activity from recombinant hTERT and hTR in the rabbit reticulocyte lysate enabled the authors to determine the effect of PNA inhibitors on the assembly pathway of telomerase. When added prior to the holoenzyme formation, IC50 values for these non-template directed PNAs range from 0.01 to 15 μM, depending on the target sequence.47 The most potent inhibitors were those complementary to specific sequences believed to be important either for hTR structure or binding to hTERT. For example, PNA 23 and PNA 22 in Table 4 inhibit telomerase activity with IC50 values of 10 and 100 nM, respectively.47
Table 4
Telomerase inhibition by non-template directed PNA (compiled from Hamilton et al).
Presumably, the mode of action of such PNA inhibitors is by trapping the RNA subunit of telomerase before it complexes with hTERT to form active telomerase. Alternatively, hybridisation of PNA to hTR may disrupt the RNA folding, distort the holoenzyme structure and adversely perturb telomerase activity. Interference in the formation of hTR RNA structure is expected by PNA 22 and PNA 23, affecting the pseudoknot domain of hTR adjacent to the template region (nt 65 to 144), and the P3 helix (nt 174 to 182), respectively.19,48 PNA 24 complementary to sequence spanning nucleotides 291 to 303 in the H/ACA box domain of hTR is also a potent inhibitor with an IC50 value of 50 nM. This region of hTR of conserved sequence is functionally important for binding hTERT as shown by Autexier et al48and Collins et al.49 When evaluated on a matured enzyme, the inhibitory effect of the non-template PNA inhibitors of telomerase decrease significantly with IC50 values ranging from 2 μM to above 100 μM (Table 4) presumably because the sequences targeted are buried within the holoenzyme and not accessible for hybridisation.47 Other approaches have been used to target non-template regions of hTR. The inhibitory effect of oligonucleotides conjugated with a 2',5'-oligoadenylate (2–5A) have been investigated.50,51,52,53 The approach relies on the mediated degradation of single-stranded regions of hTR upon binding of the oligonucleotide and activation of endoribonuclease RNase L by the 2',5'-oligoadenylate segment (Fig. 4).
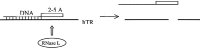
Figure 4
Schematic representation of RNase L-mediated cleavage of hTR by DNA-2',5'-oligoadenylate chimera.
The hammerhead ribozyme has also been explored for cleaving the RNA component of human telomerase.54,55,56,57 This class of inhibitors consists of two flanking antisense sequences used to position the catalytic core of the ribozyme on the site of cleavage. Yokoyama and co-workers have qualitatively demonstrated that hammerhead ribozymes have the potential to cleave hTR and reduce telomerase activity.54 However, detailed quantitative studies will be required to fully evaluate the scope of this strategy.
Cell Based Studies and in vivo Therapy
Most of the studies described above were carried out in a cell-free environment. However, a major issue concerning the development of anti-telomerase oligonucleotide inhibitors is the requirement for efficient cellular uptake and localisation. Duncan et al illustrated this with a potent PS-DNA inhibitor of telomerase in crude extract which had no inhibitory effect on telomerase in intact cells due to the oligonucleotides not being able to cross the cell membrane.58 In the last decade, significant progress has been achieved with delivery methods for transporting oligonucleotides into cells.
Delivery of Antisense Agents into Cells
The inefficient transportation of oligonucleotides and PNA across cell membranes is a limiting factor for using anti-telomerase oligomers directly in therapy.59,60 Various studies have demonstrated that the covalent conjugation of delivery vehicles to oligonucleotide and PNA can improve their uptake into living cells.61,62 The efficacy of cell-penetrating peptides, such as Antennapedia, to internalise PNA has been demonstrated in human prostate tumor DU 145 cells and human melanoma cell lines JR8 and M14.61,62 It was also demonstrated that the presence of cationic peptides (i.e., polylysine) and polyamine analogues at the termini of PNA and oligonucleotides facilitates transportation into mammalian cells.63,64 The attachment of positively charged moieties to PNA also enhances the solubility of these otherwise hydrophobic molecules. Furthermore it was demonstrated by Harrison et al that the chemical conjugation of cationic peptides to PNA enhances the inhibition of telomerase activity.65 Therefore, it is conceivable that such a modification may serve to have multiple benefits for the inhibition of telomerase in cells. The versatility of the PNA synthesis based on the well established solid phase peptide chemistry is particularly well suited for the rapid and easy development of such PNA chimeras.61,66 Alternatively, Corey and co-workers have successfully used cationic lipids to “encapsulate” 2'-O-alkyl-RNA and phosphorothioate substituted derivatives for transfection into human prostate tumor DU 145 cells.42,67 This simple lipid-mediated uptake technique is known as lipofection and it is widely used in the antisense field, but it fails to shuttle PNA into intact cells due to the neutral peptide-like backbone of the PNA which does not interact with the cationic lipids. This problem has been ingenuously overcome by using a “suicide” oligonucleotide whose function is merely to hybridise to the PNA and allow transfection by providing the negatively charged backbone required for interaction with the cationic lipids (Fig. 5).47 Upon internalisation, the partially hybridized DNA strand is degraded by endogeneous nucleases and the PNA released into the cytoplasm. Once the PNA has been delivered inside cells, it has been demonstrated by Corey and co-workers using fluorescence labelling, that PNA is retained by cells 3 to 5 days after transfection and preferentially localized within the nucleus.44,47 Such methodologies are being applied to evaluate the effects exerted by the most promising in vitro inhibitors of human telomerase in various human tumor cell lines.
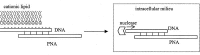
Figure 5
Schematic illustration of cationic-lipid/DNA mediated lipofection of PNA.
Effects Mediated by Antisense Telomerase Inhibitors
Based on the telomere hypothesis, anti-telomerase inhibitors should selectively interfere with telomere maintenance in telomerase-positive cancer cells. Inhibition of telomerase would be expected to result in the gradual erosion of the telomere due to the end replication problem. Sustained telomerase inhibition would lead to chromosome instability, cellular senescence and death of the cells. Present data indicate that the effect of such inhibitors in various human cancer cell lines can lead to the inhibition of telomerase activity, telomere shortening and cell death, consistent with the telomere hypothesis.44,67
Short-Term Effect of Oligonucleotides in Human Cells
The introduction of PNA and 2'-O-alkyl RNA complementary to the template of hTR has been shown to inhibit telomerase activity in various human tumor cell lines. For example, the lipid-mediated lipofection of the 13-mer PNA of sequence 5'-Gly-CAGTTAGGGTTAG-Lys-3' which inhibited telomerase activity with an IC50 value of 20 nM in vitro (PNA 10, Table 3) resulted in 90% telomerase inhibition in the human 293 cells and DU 145 prostate cancer cell lines 24 hour after transfection.47 The presence of amino acid residues at the amino and carboxy-termini of the sequence enhances the solubility of the PNA.37 The non-template directed PNA 24 (Table 4) complementary to sequence 291 to 303 of hTR was delivered into cells and inhibited telomerase by 84%.47Phosphorothioate-modified 2'-O-alkyl RNA targeted to the template region were also highly effective inhibitors of human telomerase. 2'-OMe RNA 14 (Table 3) reduced telomerase activity by 95% in human breast epithelial cells after 1 day, and 82% inhibition of telomerase activity was observed with 2'-OMOE RNA 16 (Table 3) in DU 145 cells also after 1 day.45 A significant level of telomerase inhibition (>70%) persisted for up to 3 days (∼ 3 population doublings) after lipofection of PNA 10 and 2'-OMe RNA 14. 2'-OMOE RNA 16 even continued to block telomerase activity by 76% 7 days after transfection (∼ 6 population doublings).45 These results highlight the efficacy of these oligonucleotide mimics inside cells and suggest that the amount of inhibitor initially delivered by the cationic lipid-mediated lipofection technique is sufficient to withstand dilution over successive cell divisions. Transfection of the analogous oligomers which contained 2 mismatches (2'-OMe RNA 12, 2'-OMOE RNA 13; Table 3) resulted in less effective inhibition of telomerase (typically <30% inhibition after 1 day and undetectable beyond 1 day).45
Long-Term Effect of PNA and 2'-OMe RNA Inhibitors in Cells
To assess the effect of prolonged exposure of telomerase inhibitors in living cells, Corey and co-workers transfected human HME50-5E breast epithelial and DU 145 cells every 3 to 4 days over a period of 120 days.67 The proliferation of HME50-5E and DU 145 cells treated with PNA 10 and 2'-OMe RNA 8 (Table 3) was affected with the cell number beginning to decline following 25 and 60 days of treatment, respectively.44,67 Progressive shortening of the telomere was also observed. For example, after 60 days, the median telomere length of HME50-5E treated cells decreased from 2.0 kilobase (kb) to 1.7 kb (i.e., 15%) and from 3.6 to 2.2 kb (i.e., 39%) in DU 145 cells after 76 days. The transfected HME50-5E and DU145 cells underwent apoptosis after a prolonged treatment for 110 and 120 days, respectively. In contrast, the treatment of HME50-5E and DU 145 cells with mismatched oligomers did not lead to shortened telomere or decreased cell proliferation. These results strongly suggest action through a telomerase-dependent mechanism rather than a non-selective cytotoxic effect induced by PNA or 2'-OMe RNA oligomers. The time delay between administrating the telomerase inhibitors and the observed phenotypic effects and loss of cell viability is in agreement with the hypothesis that telomerase inhibition causes gradual erosion of the telomere. The delay in each cell line appears to be dependent upon the initial telomere length. HMV50-5E cells have shorter telomeres than DU 145 cells (2.0 kb vs 3.6 kb) and thus undergo apoptosis more rapidly. Additional studies have been published that suggest a requirement for a prolonged period of inhibition before any adverse effects are apparent in the cells.8,9The lag phase has raised concern about the effectiveness of telomerase as an anti-cancer target. Owing to the need for a long-term treatment, telomerase inhibition may not be ultimately used as a primary therapy for cancer but rather in combination with conventional surgery and chemotherapy. On prolonged therapy there is also concern about severe cytotoxic side effects on highly replicative cells including the germ lines or stem cells where telomerase is expressed. However, other experimental data provide a contrary view to the above. Two oligonucleotide telomerase inhibitors have been reported to cause immediate cell death (apoptosis). Mata and co-workers have described an hexameric phosphorothioate oligonucleotide telomerase inhibitor directed to the template region of hTR that induces cell cycle arrest of lymphoma cells soon after addition.68Work by Kondo et al has also shown that a 19-mer oligonucleotide linked to a 2',5'-oligoadenylate designed to mediate the cleave of a non-template region of hTR (nt 76 to 94) triggers a 50% reduction in cell viability within 5 days of treatment in human glioma tumor (U251-MG) grown in nude mice.50 After 14 days, 79% of the cells treated with complementary oligomers underwent apoptosis. The rapid inhibition of cell proliferation exerted by 2-5A antisense (typically <7 days) was also reported in HEY-1B ovarian cancer cells,52 prostate cancer cells53 and malignant glioma cells51 demonstrating that the response observed was not specific to a particular cell type. These surprising results suggest that telomerase inhibition may also lead to mechanisms of cell death that are distinct from the gradual shortening of the telomere typically observed with competitive inhibition of human telomerase. More studies are required to determine the mechanism of action of such inhibitors and understand the complex interplay between telomerase inhibition, cell viability and the different pathways that induce cell death.
Conclusion and Future Perspectives
The inhibition of telomerase by oligonucleotides and oligonucleotide mimics that target the RNA component (hTR) has been demonstrated in a number of studies by several groups. Cell-based studies using such inhibitors have provided support for a mechanistic hypothesis of telomerase-mediated telomere maintenance, although the time period required for some of the observable effects of such inhibitors does appear to vary between reports. Further work may elucidate more mechanistic details about the action of such compounds in cells, and the consequences of inhibiting telomerase activity. There is considerable scope to improve the efficacy of this class of inhibitors by using novel oligonucleotide analogues, and also the chemical derivatisation of such molecules, for example with peptide conjugates. Whilst it has already been demonstrated how valuable such molecules are as biological tools, there is more work to be done to prove their therapeutic potential. It is likely that the outcome of clinical studies of oligonucleotide inhibitors of telomerase will be reported during the next few years.
Abbreviations
C - cytosine base or cytidine nucleotide
G - guanine base or guanosine nucleotide
hTERT - human Telomerase Reverse Transcriptase
hTR - human Telomerase RNA
IC50 - 50% inhibition coefficient
Kb - kilobase
Lys - lysine
nt - nucleotide
2'-OMe RNA - 2'-O-methyl RNA
2'-OMOE RNA - 2'-O-(2-methoxyethyl) RNA
PNA - peptide nucleic acid
PS-DNA - phosphorothioate DNA
T - thymine base or thymidine nucleotide
U - uracil base or uracil nucleotide
Acknowledgments
We thank Mark Farrow for proofreading the manuscript. We acknowledgethe support of the BBSRC, and the Cambridge European Trust.
References
- 1.
- Morin GB. The human telomere terminal transferase enzyme is a ribonucleoprotein that synthesises TTAGGG repeats. Cell. 1989; 59:521–529. [PubMed: 2805070]
- 2.
- Kim NW, Piatyszek MA, Harley CB. et al. Specific association of human telomerase with immortal cells and cancer. Science. 1994; 266:2011–2015. [PubMed: 7605428]
- 3.
- Shay JW, Wright WE. Telomerase activity in human cancer. Curr Opin Oncol. 1996; 8:66–71. [PubMed: 8868103]
- 4.
- Bodnar AG, Ouellette M, Frolkis M. Extension of life-span by introduction of telomerase into normal human cells. Science. 1998; 279:349–352. [PubMed: 9454332]
- 5.
- Newbald RF. Telomerase as an anti-cancer target: will it fulfil its early promise. Anti Cancer Drug Des. 1999; 14:349–354. [PubMed: 10625927]
- 6.
- Hahn WC, Stewart SA, Brooks MW. et al. Inhibition of telomerase limits the growth of human cancer cells. Nat Med. 1999; 5(10):1164–1130. [PubMed: 10502820]
- 7.
- Colgin LM, Wilkinson C, Englezou A. et al. The hTERT alpha splice variant is a dominant negative inhibitor of telomerase activity. Neoplasia. 2000; 2(5):426–432. [PMC free article: PMC1507985] [PubMed: 11191109]
- 8.
- Zhang XL, Mar V, Zhou W. et al. Telomere shortening and apoptosis in telomerase-inhibited human tumor cells. Genes Dev. 1999; 13(18):2388–2399. [PMC free article: PMC317024] [PubMed: 10500096]
- 9.
- Feng J, Funk WD, Wang SS. et al. The RNA component of human telomerase. Science. 1995; 269:1236–1240. [PubMed: 7544491]
- 10.
- Bisoffi M, Chakerian AE, Fore ML. et al. Inhibition of human telomerase by a retrovirus expressing telomeric antisense RNA. Eur J Cancer. 1998; 34(8):1242–1249. [PubMed: 9849487]
- 11.
- Gomez DE, Tejera AM, Olivero OA. Irreversible telomere shortening by 3'-azido-2'-3'-dideoxythymidine (AZT) treatment. Biochem Biophys Res Commun. 1998; 246:107–110. [PubMed: 9600076]
- 12.
- Yegorov YE, Akimov SS, Akhmalisheva AK. Blockade of telomerase function in various cells. Anti-Cancer Drug Design. 1999; 14:305–316. [PubMed: 10625923]
- 13.
- Perry PJ, Read MA, Davies RT. et al. 2,7-disubstituted amidofluorenone derivatives as inhibitors of human telomerase. J Med Chem. 1999; 42:2679–2684. [PubMed: 10411488]
- 14.
- Mergny JL, Mailliet P, Lavelle F. et al. The development of telomerase inhibitors: the G-quartet approach. Anti Cancer Drug Des. (1999);14:327–339. [PubMed: 10625925]
- 15.
- Phillips MI, Zhang YC. Basic principles of using antisense oligonucleotides in vivo. Methods Enzymol. 2000;313:46–56. [PubMed: 10595348]
- 16.
- Guinot P, Temsamani J. Antisense oligonucleotides: a novel therapeutic approach. Pathol Biol. 1998;46(5):347–354. [PubMed: 9769897]
- 17.
- Yokoyama Y, Takahashi Y, Shinohara A. et al. The 5'-end of hTERT mRNA is a good target for hammerhead ribozyme to suppress telomerase activity. Biochem Biophys Res Commun. 2000;273(1):316–321. [PubMed: 10873604]
- 18.
- Ludwig A, Saretzki G, Holm PS. et al. Ribozyme cleavage of telomerase mRNA sensitizes breast epithelial cells to inhibitors of topoisomerase. Cancer Res. 2001;61(7):3053–3061. [PubMed: 11306487]
- 19.
- Chen JL, Blasco MA, Greider CW. The secondary structure of vertebrate telomerase RNA. Cell. 2000;100:503–514. [PubMed: 10721988]
- 20.
- Shippen-Lentz D, Blackburn EH. Functional evidence for an RNA template in telomerase. Science. 1990;247:546–1270. [PubMed: 1689074]
- 21.
- Autexier C, Greider CW. Boundary elements of the Tetrahymena telomerase RNA template and alignment domains. Genes Dev. 1995;9(18):2227–2239. [PubMed: 7557377]
- 22.
- Nakamura TM, Morin GB, Chapman KB. et al. Telomerase catalytic subunit homologs from fission yeast and human. Science. 1997;277:955–959. [PubMed: 9252327]
- 23.
- Norton JC, Piatyszek MA, Wright WE. et al. Inhibition of human telomerase activity by peptide nucleic acids. Nat Biotechnol. 1996;14:615–619. [PubMed: 9630953]
- 24.
- Hamilton SE, Pitts AE, Katipally RR. Identification of determinants for inhibitor binding within the RNA active site of human telomerase using PNA scanning. Biochemistry. 1997;36:11873–11880. [PubMed: 9305980]
- 25.
- Shaw JP, Kent K, Bird J. et al. Modified deoxyoligonucleotides stable to exonuclease degradation in serum. Nucleic Acid Res. 1991;19:747–750. [PMC free article: PMC333706] [PubMed: 1850122]
- 26.
- Stein CA, Cohen JS. Oligodeoxynucleotides as inhibitors of gene expression. Cancer Res. 1988;48:2659–2668. [PubMed: 3282648]
- 27.
- Eckstein F. Phosphorothioate oligonucleotides: what is their origin and what is unique about them? Antisense Nucleic Acid Drug Dev. 10:117–121. [PubMed: 10805163]
- 28.
- Crooke ST, Groham MJ, Zuckermann JE. et al. Pharmacokinetic properties of several oligonucleotide analogues in mice. J Pharmacol Exp Ther. 277:923–937. [PubMed: 8627575]
- 29.
- Krieg AM, Stein CA. Phosphorothioate oligodeoxynucleotides: antisense or anti-protein? Antisense Res Dev. 1995;5:241. [PubMed: 8746772]
- 30.
- Levin AA. A review of issues in the pharmacokinetics and toxicology of phosphorothioate antisense oligonucleotides. Biochimica et Biophysica Acta. 1999;1489:69–84. [PubMed: 10806998]
- 31.
- de Smet MD, Meenken CJ, van den Horn GJ. et al. Fomivirsen- a phosphorothioate oligonucleotide for the treatment of CMV retinitis. Ocul Immunol Inflamm. 1999;7(3):189–198. [PubMed: 10611727]
- 32.
- Cunningham CC, Holmlund JT, Schiller JH. et al. A phase I trial of c-Raf kinase antisense oligonucleotide ISIS 5132 administered as a continuous intravenous infusion in patients with advanced cancer. Clin Cancer Res. 2000;5:1607–1610.
- 33.
- Monia BP, Lesnik EA, Gonzalez C. et al. Evaluation of 2'-modified oligonucleotides containing 2'-deoxy gaps as antisense inhibitors of gene expression. J Biol Chem. 1993;268:14514–14522. [PubMed: 8390996]
- 34.
- Monia BP, Johnston JF, Sasmor H. et al. Nuclease resistance and antisense activity of modified oligonucleotides to target to Ha-ras. J Biol Chem. 1996;271:14533–14540. [PubMed: 8662854]
- 35.
- Khatsenko O, Morgan R, Truong L. Absorption of antisense oligonucleotides in rat intestine: effect of chemistry and length. Antisense Nucleic Acid Drug Dev. 2000;10:35–44. [PubMed: 10726659]
- 36.
- Chen HX, Marshall JL, Ness E. et al. A safety and pharmacokinetic study of a mixed-backbone oligonucleotide (GEM 231) targeting the type I protein kinase A by 2-hour Infusions in patients with refractory solid tumors. Clin. Cancer Res. 2000;6: 1259–1266. [PubMed: 10778949]
- 37.
- Nielsen PE, Egholm M, Berg RH. et al. Sequence-selective recognition of DNA by strand displacement with thymine-substituted polyamide. Science. 1991;254:1497–1500. [PubMed: 1962210]
- 38.
- Egholm M, Buchardt O, Christiansen L. et al. PNA hybridises to complementary oligonucleotides obeying the Watson-Crick hydrogen bonding rules. Nature. 1993;365:566–568. [PubMed: 7692304]
- 39.
- Demidov V, Potaman VN, Kamenetskii F. et al. Stability of peptide nucleic acids in human serum and cellular extracts. Biochem Pharmacol. 1994;48:1310–1313. [PubMed: 7945427]
- 40.
- Blasco MA, Funk W, Villeponteau B. et al. Functional characterization and development regulation of mouse telomerase RNA. Science. 269:1267–1270. [PubMed: 7544492]
- 41.
- Glukhov AI, Zimnik OV, Gordeev SA. et al. Inhibition of telomerase activity of melanoma cells in vitro by antisense oligonucleotides. Biochem Biphys Res Commun. 1998;248:368–371. [PubMed: 9675142]
- 42.
- Pitts AE, Corey DR. Inhibition of human telomerase by 2'-O-methyl RNA. Proc Natl Acad Sci USA. 1998;95:11549–11554. [PMC free article: PMC21678] [PubMed: 9751703]
- 43.
- Matthes E, Lehmann C. Telomerase protein rather than its RNA is the target of phosphorothioate-modified oligonucleotides. Nucleic Acid Res. 1999;27(4):1152–1158. [PMC free article: PMC148297] [PubMed: 9927750]
- 44.
- Shammans MA, Simmons CG, Corey DR. et al. Telomerase inhibition by peptide nucleic acids reverse immortality of transformed cells. Oncogene. 1999;18:6191–6200. [PubMed: 10597217]
- 45.
- Elayadi AN, Demieville A, Wancewicz EV. et al. Inhibition of telomerase by 2'-O-(2-methoxyethyl) RNA oligomers: effect of length, phosphorothioate substitution and time inside cells. Nucleic Acids Res. 2001;29(8):1683–1689. [PMC free article: PMC31310] [PubMed: 11292840]
- 46.
- Yu D, Iyer RP, Lisziewicz J. et al. Hybrid oligonucleotides:synthesis, biophysical properties, stability studies and biological activity. Bioorg Med Chem. 1996;4(10):1685–1692. [PubMed: 8931938]
- 47.
- Hamilton SE, Simmons CG, Kathiriya IS. et al. Cellular delivery of peptide nucleic acid and inhibition of human telomerase. Chem Biol. 1999;6:343–351. [PubMed: 10375543]
- 48.
- Bachand F, Autexier C. Functional region of human telomerase reverse transcriptase and human telomerase RNA required for telomerase activity and RNA-protein interactions. Mol Cell Biol. 2001;21(5):1888–1897. [PMC free article: PMC86762] [PubMed: 11238925]
- 49.
- Mitchell JR, Collins K. Human telomerase activation requires 2 independent interactions between telomerase RNA and telomerase reverse transcriptase. Mol Cell. 2000;6: 361–371. [PubMed: 10983983]
- 50.
- Kondo S, Kondo Y, Li GY. et al. Targeted therapy of human malignant glioma in a mouse model by 2–5 antisense directed against telomerase RNA. Oncogene. 1998;16(25):3323–3330. [PubMed: 9681832]
- 51.
- Mukai S, Kondo Y, Koga S. et al. 2–5A antisense telomerase RNA therapy for intracranial malignant gliomas. Cancer Res. 2000;60(16):4461–4467. [PubMed: 10969793]
- 52.
- Kushner DM, Paranjape JM, Bandyopadhyay B. et al. 2–5A antisense directed against telomerase RNA produces apoptosis in ovarian cancer cells. Gynecologic Oncology. 2000;76(2): 183–192. [PubMed: 10637068]
- 53.
- Kondo Y, Koga S, Komata T. et al. Treatment of prostate cancer in vitro and in vivo with 2–5 anti-telomerase RNA component. Oncogene. 2000;19(18):2205–2211. [PubMed: 10822370]
- 54.
- Yokoyama Y, Takahashi Y, Shinohara A. et al. Attenuation of telomerase activity by a hammerhead ribozyme targeting the template region of telomerase RNA in endometrial carcinoma cells. Cancer Res. 1998;58(23):5406–5410. [PubMed: 9850072]
- 55.
- Wan MS, Fell PL, Akhtar S. Synthetic 2'-O-methyl-modified hammerhead ribozymes targeted to the RNA component of telomerase as sequence-specific inhibitors of telomerase activity. Antisense Nucleic Acid Drug Dev. 8:309–317. [PubMed: 9743468]
- 56.
- Kanazawa Y, Ohkawa K, Ueda K. et al. Hammerhead ribozyme mediated inhibition of telomerase activity in extracts of human hepatocellular carcinoma cells. Biochem Biophys Res Commun. 1996;225:570–576. [PubMed: 8753802]
- 57.
- Folini M, Colella G, Villa R. et al. Inhibition of telomerase activity by a hammerhead ribozyme targeting the RNA component of telomerase in human melanoma cells. J Invest Dermatol. 2000;114(2):259–67. [PubMed: 10651984]
- 58.
- Duncan VE, Ajmani PS, Hughes JA. Cellular delivery is a major obstacle for oligodeoxynucleotide inhibition of telomerase activity. Anticancer Res. 1998;18(6):4105–4108. [PubMed: 9891453]
- 59.
- Wickstrom E. Strategies for administrating targeted therapeutic oligonucleotides. Trends Biotechnol. 1992;10:281–287. [PubMed: 1368381]
- 60.
- Wittung P, Kajanus J, Edwards K. et al. Phospholipid membrane permeability of peptide nucleic acid. FEBS Lett. 1995;27(1):27–29.
- 61.
- Simmons CG, Pitts AE, Lynn D. et al. Synthesis and membrane permeability of PNA-peptide conjugates. Biorg Med Chem Lett. 1997;7(23):3001–3006.
- 62.
- Villa R, Folini M, Lualdi s. et al. Inhibition of telomerase activity by a cell penetrating peptide nucleic acid construct in human melanoma cells. FEBS Lett. 2000;473:241–248. [PubMed: 10812083]
- 63.
- Leonetti JP, Degols G, Lebleu B. Biological activity of oligonucleotide-poly(L-lysine) conjugates: mechanism of cell uptake. Bioconjug Chem. 1990;1(2):149–153. [PubMed: 1965625]
- 64.
- Thomas RM, Thomas T, Wada M. et al. Facilitation of the cellular uptake of a triplex-forming oligonucleotide by novel polyamine analogues: structure-activity relationship. Biochemistry. 1999;38:13328–13337. [PubMed: 10529208]
- 65.
- Harrison J, Frier C, Laurant R. et al. Inhibition of human telomerase by PNA-cationic peptide conjugates. Bioorg Med Chem Lett. 1999;9:1273–1278. [PubMed: 10340613]
- 66.
- Norton JC, Waggenspack JH, Varnum E. et al. Targeting peptide nucleic acid-protein conjugates to structural features within duplex DNA. Bioorg Med Chem. 1995;3(4):437–445. [PubMed: 8581427]
- 67.
- Herbert BS, Pitts AE, Baker SI. et al. Inhibition of human telomerase in immortal human cells leads to progressive telomere shortening and cell death. Proc Natl Acad Sci USA. 1999;96(25):14276–14281. [PMC free article: PMC24427] [PubMed: 10588696]
- 68.
- Mata JE, Joshi SS, Palen B. et al. A hexameric phosphorothioate oligonucleotide telomerase inhibitor arrests growth of Burkitt's lymphoma cells in vitro and in vivo. Toxicol Appl Pharmacol. 1997;144:189–197. [PubMed: 9169084]
- PNA and Oligonucleotide Inhibitors of Human Telomerase - Madame Curie Bioscience...PNA and Oligonucleotide Inhibitors of Human Telomerase - Madame Curie Bioscience Database
Your browsing activity is empty.
Activity recording is turned off.
See more...